Fig. 6.1
The marmoset, Callithrix jacchus, is a small diurnal primate morphologically characterized by the presence of typical white tufts. (From Christiane A. Silva/LNRB)
Besides its easy maintenance in the laboratory, the marmoset has complex behavioral and vocal repertoires (Stevenson and Rylands 1988; Bezerra and Souto 2008), learning ability in cognitive tasks common to Old World monkeys (Dias et al. 1996; Spinelli et al. 2004; Mendes and Huber 2004), and physiological variables comparable to humans (McNees et al. 1984; Smith et al. 2001; Mansfield 2003), being also used as an animal model for aging studies (Tardif et al. 2011). Additionally, the marmoset behavioral repertoire has been described since the 1970s (Stevenson and Poole 1976), including the behaviors of anxiety and alertness, which are used as study variables in investigations into the administration of anxiogenic and anxiolytic drugs such as diazepam, amphetamine, and modafinil (Barros and Tomaz 2002; van Vliet et al. 2008; Cilia and Piper 1997 ).
We highlight, specifically, its importance in research on internal temporal organization, because in a PubMed search using only the keyword “marmoset” we found 4,166 indexed articles (April 2013), but with the keywords “marmoset and circadian” or “marmoset and sleep,” we found only 50 and 19 items, respectively. In this chapter we describe in detail the recent experimental findings produced in our laboratory and reported in the scientific literature, which allow us to propose the marmoset as a potential model for the study of circadian rhythmicity.
6.2 Marmoset Circadian Rhythmicity
The marmoset is a neotropical primate with diurnal habits, and a qualified literature describes the general characteristics of circadian rhythmicity in this species. Studies conducted in the laboratory (Crofts et al. 2001; Philippens et al. 2004; Hoffmann et al. 2012), in seminatural conditions (Menezes et al. 1993), and in the field (Castro et al. 2003) show a diurnal concentration pattern of the marmoset active phase.
In the natural environment the marmosets awake before sunrise, but only come out of their sleeping trees to begin locomotor activity after sunrise, and generally finish the active phase (alpha) about 1 to 2 h before sundown (Erkert 1989; Menezes et al. 1993; Moreira et al. 1991). Under an artificial light–dark cycle (LD 12:12), they present an average alpha of 11.1 ± 0.7 h (Erkert 1989), very similar to that found in studies in natural environmental conditions, 11.6 ± 0.5 h (Menezes et al. 1993). The activity pattern is usually observed as a bimodal profile for locomotor activity, with one peak early in the morning and another one in the afternoon (Fig. 6.2) (Erkert 1989; Menezes et al. 1993; Moreira et al. 1991), whereas other behaviors present a unimodal profile, such as self-grooming, whose peak of duration occurs in the first half of the light phase (Lampert et al. 2011; Menezes et al. 1993).
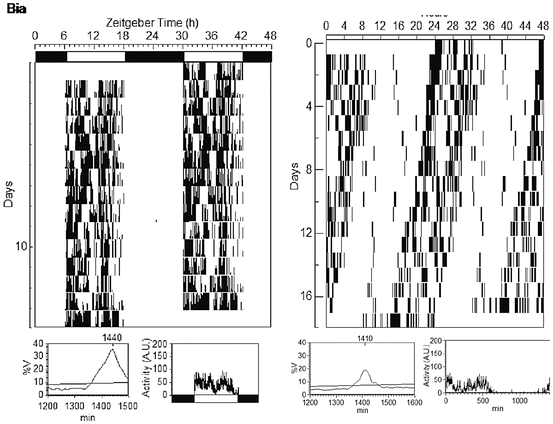
Fig. 6.2
At left, 14 days of an adult female under (light–dark) LD 12:12 (≈247:0 lux). At right, 18 days of an adult male under LL (≈225 lux). Actograms are in module = 24 h. Data are totalized in 5-min intervals
Under constant environmental conditions, that is, constant light, the marmoset exhibits stable motor activity circadian rhythms (Erkert 1989; Glass et al. 2001) with an endogenous period around 23.3 ± 0.4 h (Erkert 1989; Glass et al. 2001; Silva et al. 2005) and an alpha average duration of 11.3 ± 0.4 h (Fig. 6.2).
According to Erkert (1989) there is no change in the motor activity circadian rhythm of marmosets under different light intensities. However, our findings in marmosets with visual impairment, a congenital blindness caused by degeneration of rods and cones, showed that these animals synchronize the circadian rhythm of locomotor activity to the LD cycle, presented a free-running period different from the control group, and this followed Aschoff’s rule (Silva et al. 2005). On the other hand, a finding with Macaca nemestrina, an Old World monkey species, shows a positive correlation of period, activity duration, and amount of locomotor activity per period with light intensity (Tokura and Aschoff 1978).
Another study by our group showed that the self-grooming rhythm is also affected by luminous intensity. Under symmetrical LD cycles, the duration and frequency of self-grooming tend to increase when luminous intensity of the light phase varies from 500 to 200 lux but decrease notably under the luminous intensity of 10 lux (Lampert et al. 2011).
The photic phase-response curve of marmosets is characterized by phase delays after pulses in the early subjective night and phase advances after pulses in the late subjective night, similar to that which occurs in mammals in general. The only difference is that the marmosets presented great phase changes in motor activity during the subjective day, when low responsiveness of the circadian timing system to punctual changes in light intensity is expected (Wechselberger and Erkert 1994). According to the authors, the direct influence of light on the circadian system or a nonphotic effect of a behavioral activation induced by light may have caused these phase changes.
Other parameters, besides the observation of locomotor activity, also corroborate the earlier statement, which can be exemplified by temperature variation throughout the 24 hours. Marmosets have elevated temperatures during the day (because of their diurnal habits) that decline during the dark phase (Hoffmann et al. 2012). The levels of cortisol hormone also present circadian variation, with elevated cortisol levels during the beginning of the active phase that significantly decrease during the day (Cross and Rogers 2004). Furthermore, it is observed that when tested for warming level, significant results are allocated in the light phase (Van Vliet et al. 2008; Hoffmann et al. 2012). Therefore, based on all the points raised, there is no doubt that the marmoset is an animal genuinely diurnal.
Although the LD cycle is the main zeitgeber for marmosets, nonphotic cues can also act as zeitgebers for these animals, synchronizing the circadian rhythms in the absence of an external LD cycle or acting in conjunction with photic zeitgebers in the photic resynchronization. Exemplifying these conditions, it was observed that the photic resynchronization to phase delays was accelerated in marmosets by the presence of a conspecific previously synchronized to the new lighting time (Mendes et al. 2008). In two other studies, modulations in motor activity circadian rhythmicity in response to the vocalizations emitted from conspecifics were observed, suggesting synchronization by the sound. These vocalizations were audible in the experiment room and were able to promote positive masking and relative coordination, associated with an increase in activity frequency, in locomotor activity of the animals in one of the studies (Silva et al., unpublished data). In the other study, conducted in a room that allowed the entrance of more vocalizations from conspecifics kept outside than in the previous study, conspecific vocalizations were able to entrain the locomotor activity of three of a total of four marmosets in the experiment, and keeping the same phase relationship that the animals kept outside the room (Gonçalves et al. 2009).
Another important finding that confirms the marmoset diurnality is that, regardless of the latitude where the marmoset lives and seasonality throughout the year, the active phase remains in the light phase (Menezes et al. 1998; Melo et al. 2010). In experiments with short pulses of light of only 20 s and a 1-h induced activity pulse, Glass et al. (2001) were able to construct a phase-response curve to photic stimulus and also a phase-response curve for nonphotic stimulus, respectively. Then, it was observed that the phase-response curves of marmosets are similar to those of rodents, in which the key to the response is the phase of the circadian pacemaker and not that of the activity–rest cycle.
6.3 Photoperiodic Responses in Marmosets
One of the important features for evaluating a circadian system is its response to photoperiod, because the seasonal variations in environmental parameters also promote physiological and behavioral changes and are relevant to the survival of the species. Faced with recurring events, such as the seasons, the organisms can organize their activities to be previously prepared to changes, exhibiting an anticipatory behavior (Golombek and Rosenstein 2010). One of these recurrent events is the photoperiod, that is, the light phase of the day, which passes for different durations and intensities throughout the year and serves as an indicator of seasonality. The photoperiod, food availability, favorable variation in temperature, and rainfall are a set of factors that allow animals to reproduce, migrate, hibernate, dig burrows, and store food when environmental conditions are more favorable, which maximizes an appropriate response to a cyclic environment (Wehr 2001a).
Generally, studies related to seasonality are accomplished with mammalian species that present markedly large changes, resulting from changes in melatonin signaling. As this hormone is secreted at night, the information received by the organism about daylength on these photoperiodic species leads to integrated solutions throughout the system (Wehr 2001a; Nelson et al. 2002). However, not all mammals that are influenced by seasonality have broad answers in their temporal organization.
Among the mammals, seasonality can also be observed in human and nonhuman primates. In human species, studies point to a variation in the birthrate, disease incidence, and immune system responses, in hormonal rhythms (e.g., melatonin, cortisol, prolactin), mood changes, and eating behavior (Wehr et al. 1993; Wehr 2001b; Nelson et al. 2002; Walton et al. 2011). In nonhuman primates, we can mention some changes specifically caused by photoperiod, especially in studies with the nocturnal lemur Microcebus murinus, such as changes in activity–rest cycle, temperature rhythm, the steroid hormone DHEA secretion, and metabolic rates (Schilling et al. 1999; Perret and Aujard 2001, 2005; Perret et al. 1998). Changes in locomotor activity rhythm of this nocturnal lemur, which are common in aging, were also observed when the seasonal cycles were accelerated (Perret 1997; Cayetanot et al. 2005). Thus, this nocturnal primate has been pointed out as a model to study the changes in aging of rhythmicity (Aujard et al. 2006; Perret and Aujard 2006).
In our laboratory, we have developed studies of photoperiodic responses in the marmoset. Here we highlight some points along with the studies of other groups.
6.4 Behavioral Aspects of Seasonality in Marmosets
Despite the small variation in photoperiod, animals naturally from equatorial latitudes, such as C. jacchus, may have changes in their behavioral repertoire influenced by seasonality. Studies in seminatural conditions, with the time of sunrise varying as much as 40 min, showed a change at the beginning of activity and the bimodal pattern of locomotor circadian rhythm in infants and juveniles (1–10 months old: Menezes et al. 1998; 5–12 months old: Melo et al. 2010).
In artificial conditions of short (LD 8:16) and long (LD 16:08) photoperiods, very different from the marmoset natural environment but found at nonequatorial high-latitude regions, the adult marmosets showed changes in various parameters of circadian rhythmicity, although indicating synchronization of the activity–rest cycle to the photoperiod of long and short days (Carrijo 2013). In short days a decrease in total daily activity and the duration of alpha was observed. On the other side, in the long days there was an increase in the duration of alpha and a decrease in the amplitude and spectral power of the circadian rhythm period.
In our studies we found individual variation in the phase relationship between the onset of light and the beginning of activity, so we suggest that marmosets may use different strategies in response to short and long days, but they all result in synchronization to the tested photoperiods. Additionally, the phase transitions of one LD cycle to another did not occur similarly in all conditions. The decrease of daylength from 12 to 8 h appears to force further the timing system to adjust the onset of locomotor activity, whereas the increase from 12 to 16 h and the decrease from 16 to 12 h promoted the adjustment with greater speed of synchronization.
These differential post-effects during synchronization of the activity–rest rhythm to phase advances or delays were equally observed for the nocturnal species lemur Microcebus murinus (Schilling et al. 1999), suggesting that the circadian system functionally works in the same way in diurnal and nocturnal animals. Investigations such as these contribute to the findings on light as a cue of extreme relevance to the primate temporal organization. An example can be observed in humans when undergoing abrupt phase changes in transmeridian flights. There are indications that exposure to light is the best therapeutic strategy to reduce the effects of jet lag (Lee and Galvez 2012), but as the symptoms of jet lag are quite large and complex, further investigations to clarify and establish an adequate prevention strategy in every situation are necessary (Kolla and Auger 2011). We suggest that the marmoset model is an efficient model to conduct these investigations.
Ease or difficulty in adjustment of the circadian timing system to shifts in the environmental light cycle is the result of functional mechanisms related to the interactions among SCN oscillators. This process is determined by the coupling among these oscillators. The physiological and structural mechanisms of coupling among circadian oscillators in the SCN is still an open question, but some evidence suggests that results of several mechanisms such as gap junctions, GABAergic synapses, neuronal adhesion molecules, etc., are involved. Electron microscopy studies are being conducted with the intention of clarifying these mechanisms.
As the marmoset is originally from northeastern Brazil (Rylands et al. 2009), a region of little photoperiodic variation throughout the year (lower latitudes), we could imagine that these animals would not respond distinctly to short and long photoperiods (Fig. 6.3). Thus, the flexibility in temporal adjustment of the marmoset activity–rest cycle to different photoperiods allows these species to have an easy adaptation to different regions, as observed by the presence of C. jacchus from north to south in Brazil (Rylands et al. 2008). On the other hand, this flexibility also adds an advantage to the use of this animal as a nonhuman primate model because C. jacchus is found at research institutions in several countries (Abbott et al. 2003) with more pronounced photoperiodic variations (considering that the colonies may have a natural LD cycle, beyond the artificial 12:12).
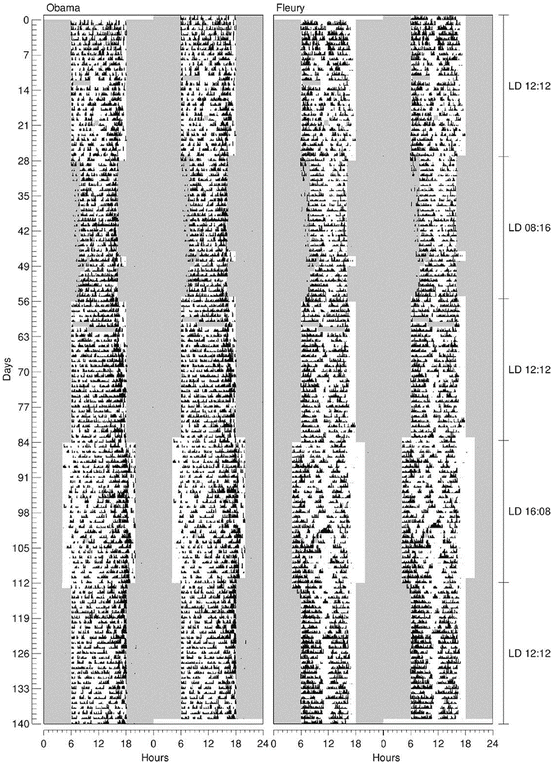
Fig. 6.3
Actograms of two marmosets under symmetrical, short, and long photoperiods: LD 12:12 (day 0), LD 08:16 (day 28), LD 12:12 (day 56), LD 16:08 (day 84), LD 12:12 (day 112). Locomotor activity was recorded by passive infrared sensors positioned over the individual cages. Observing the phase angles between light and activity, it is possible to see the different strategies of adjustment to the light–dark cycle to which the animals were exposed. Under LD 16:08 the animals lengthened the active phase, or by delaying the active phase ending (left), or by advancing the beginning of the active phase (right) (Carrijo 2013)
In our studies we also observed that marmosets altered everyday activities in response to the change of a symmetrical photoperiod (12 h light and 12 h dark) to a long photoperiod (16 h light and 8 h dark), simulating the daylength of summer at high-latitude regions. Considering the initial peak of activity usually seen in the marmoset, the animals exhibited a reduction in the rate of vocalization, impoverishment of the vocal repertoire, and decreased exploratory activity stimulated by unfamiliar objects. On the other hand, short days did not affect these parameters. Because in their home region the longer days (indicating summer) are characterized by drought and food shortages, the marmosets are more subject to predation and territory defense. Based on the vocalizations observed in the initial peak of activity in the long days in our study (absence of the long-distance calls, “loud shrill,” and decrease of the intragroup calls, “phee”), we can speculate that this is a possible mechanism of response to this situation in wild life for the marmosets not so exposed. Additionally, as the emission of agonistic and alarm calls (twitter, tsik, see, seep) did not change among photoperiods, we suggest that if the daylength is indeed a factor influencing the rate and the type of vocalization, it would not be advantageous to reduce the emission of alarm callings. In this way, the territory and group defense would be maintained when they are most vulnerable to predation and competition in the wild (Carrijo 2013).
Besides the availability of resources, we could imagine that it would not be problematic to allocate activities into the photophase of 8 h because the active phase of the marmoset is less than the daylength of 12 h. Thus, allocation of daily activities in a photophase of 16 h would cause a change in the distribution of activities during the long day. Thus, in relationship to the photoperiods tested, we suggest that increase of photophase is more challenging to marmoset behavioral responses, in contrast to the decrease in photophase duration. This observation is the opposite of what is discussed for species living at high latitudes because they undergo a winter marked by food shortages and low temperatures (Hill et al. 2003; Nelson et al. 2002).
In addition to the laboratory studies just cited, a seasonal pattern in the natural environment in marmoset feeding behavior has also been reported. Castro et al. (2003) observed changes in the pattern of space use by C. jacchus between feeding stations with additional behavioral plasticity according to food availability and distribution, even without the marmosets extending the size of the living area. On the other hand, Callithrix penicillata can vary the predominant activity pattern, alternating between foraging a punctual resource (insects) or displacing in search of other food items, depending on fruit availability and distribution (Vilela and Faria 2004). Similarly, considering other callitrichids, species of the genus Saguinus presented alternate responses according to the temporal and spatial distribution of resources over the months, intensively exploring a few food species or being opportunistic and exploring many species. They also experienced the influence of seasonality in resting, grooming, foraging, and displacement behaviors (Egler 2000).
These changes resulting from the seasons and, consequently, from food availability may be related to more propitious moments for breeding throughout the year, which has been observed for both diurnal (C. jacchus: Sousa et al. 1999a, b) and nocturnal (Aotus azarai: Fernandez-Duque et al. 2002) primates. Regarding C. jacchus, there is evidence that progesterone secretion by the corpus luteum can be induced by melatonin administration (Webley and Hearn 1987; Hearn and Webley 1987), the photoperiod biological signal, indicating that marmoset reproductive functions have the potential to be stimulated by photoperiodic variations. This point was suggested by Sousa and collaborators (1999a), who observed a seasonal pattern in the birth of baby marmosets in seminatural captivity, even without variation in food supply. The authors speculated that the observed birth pattern could be related to factors such as the rainy season or photoperiod, even in a region close to Ecuador, that is, with little photoperiodic variation. A discussion of the proximate and ultimate functions of births seasonality observed in several genera of neotropical primates is found in the review of Di Bitetti and Janson (2000).
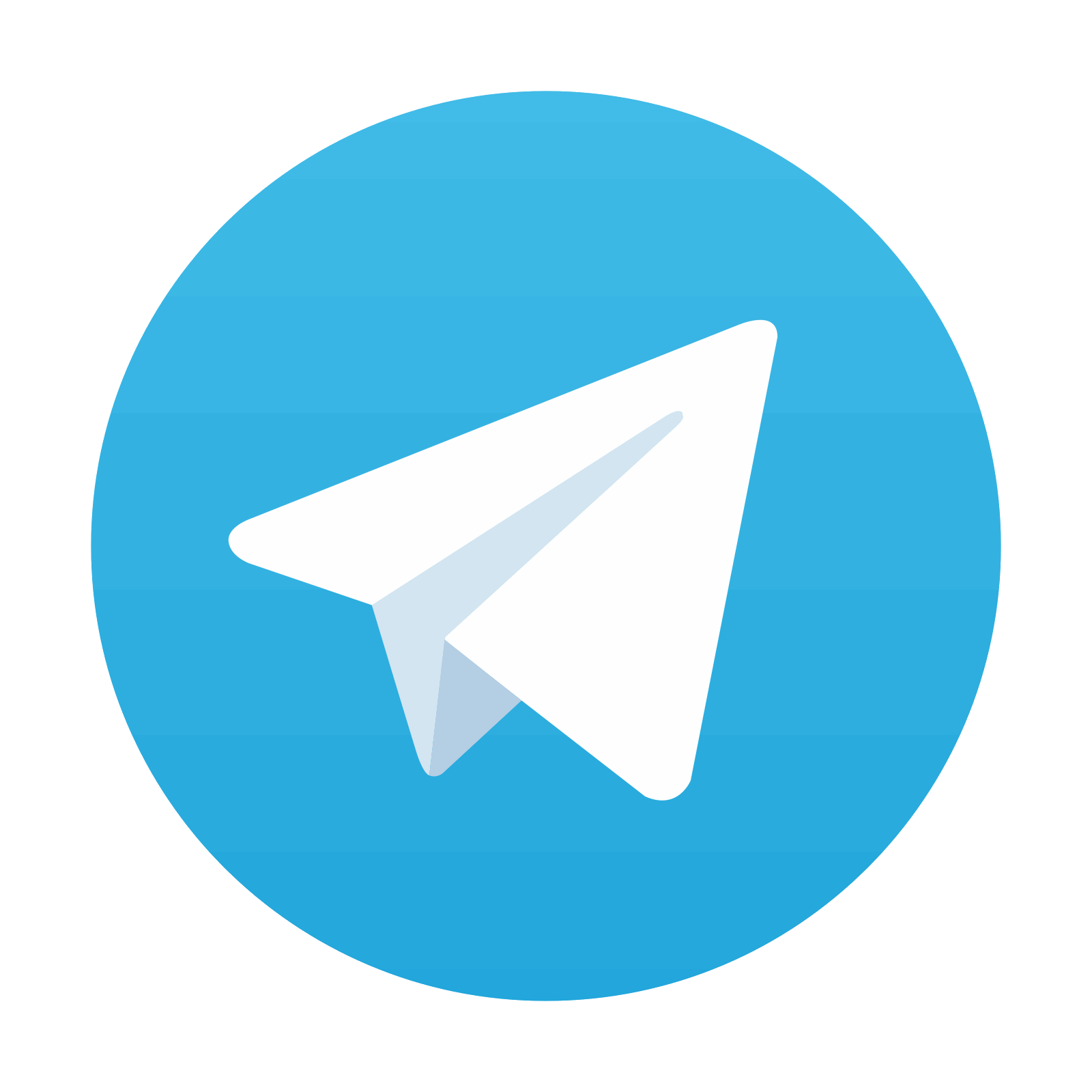
Stay updated, free articles. Join our Telegram channel

Full access? Get Clinical Tree
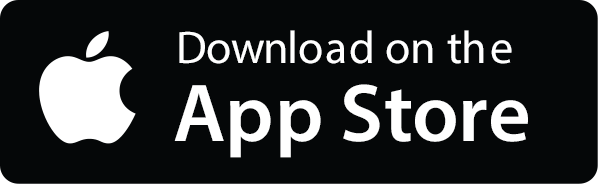
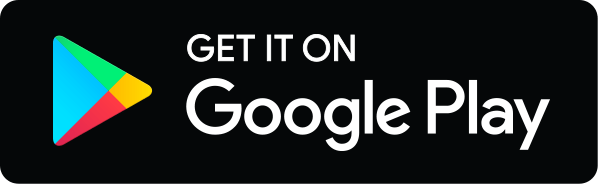