Animal species
Fluoride in feed (ppm)
Fluoride in water (ppm)
Beef and dairy heifers
30
2.5–4
Mature dairy cattle
30
3–6
Mature beef cattle
40
4–8
Sheep
50
12–15
Horse
60
4–8
Swine
70–100
5–8
Dog
100
–
Poultry
100
10–13
Sheep and goats can tolerate somewhat higher dietary levels of fluoride than cattle, although clinical observations in hydrofluorotic areas may show wide variation. In a population of 780 goats and 564 sheep inhabiting areas where mean water fluoride concentration was 3.2 ppm, none revealed any evidence of osteo or dental fluorosis (Choubisa 1999). But later on, authors in a survey of 356 goats and 248 sheep inhabiting areas where mean water fluoride concentration varied between 1.5 and 1.7 ppm, observed that the prevalence of osteo and dental fluorosis in goats (10.7 and 8.4 %) was higher than in sheep (7.3 and 5.6 %; Choubisa et al. 2011). Clinical signs recorded in different stages of experimental fluoride toxicity in sheep included anorexia, poor body condition, lameness, mandibular thickening, excessive wear of molar teeth, and pale mucosa (Zhou and Zhai 1991). As with cattle and water buffaloes, dental lesions appear early and more frequently than bony lesions in natural cases of fluorosis in sheep and goats (Guo et al. 2002; Singh et al. 2002).
The dromedary camel has high fluoride tolerance as evident from the absence or very low frequency of dental and bony lesions in camels reared in hydrofluorotic areas (Choubisa et al. 2011; Choubisa 2013a). This is perhaps due to their unique morphoanatomic and physiological adaptations such as low water turnover rate, ability to survive without water for up to 20 days, and some other unknown reasons. Nevertheless, dental and bony lesions of fluorosis may appear in camels reared in areas where water fluoride concentration is as low as 1.45 ppm (Choubisa 2013b). Anemia was reported as a significant hematological finding in camels suffering from industrial fluoride pollution (Karram and Ibrahim 1992). Dental lesions in camels appear as nonstriated, vertical, homogeneous, light-brown discoloration which is different from bovines where dental lesions are characterized by the presence of horizontal striated deep-brown discoloration. The frequency and severity of dental and bony lesions in camel calves are lesser than in adults and aged animals, which is opposite to that observed in cattle and water buffaloes (Choubisa 2013b).
Reports on the natural occurrence of fluorosis in horses are very few. In fluorosis-endemic areas, horses do not suffer as frequently as cattle, sheep, or swine. Horses are considered to have a higher tolerance to fluoride than other domestic animals. However, horses grazing in areas where fluorotic lesions are common in cattle and sheep may develop similar dental lesions (Shupe and Olson 1971). In a research report, it was claimed that artificially fluoridated water was responsible for colic, stiffness, and lameness observed in horses on a farm in Colorado, as the frequency of these problems gradually reduced when water fluoridation was stopped (Burgstahler 2006). However, such claims require further investigation for scientific validation.
Swine perhaps have the highest fluoride tolerance among different domestic animals. Domestic pigs are largely raised on high grain diets which usually have low fluoride and high calcium concentrations. Dental lesions include enamel hypoplasia and mottling, impaired milk production, and loss of body weight; a disturbed estrous cycle was recorded in sows fed on diet containing more than 500 ppm fluoride concentration (NRC 1960). Pathological changes in teeth including disturbed enamel formation along with high F accumulation was reported in wild boars from the Czech Republic and Germany (Kierdorf et al. 2000, 2005).
Fluoride tolerance appears high in dogs as there is a paucity of reports on occurrence of dental and bony lesions in dogs reared in fluorosis-endemic areas. Adult dogs can tolerate a daily intake of 5 mg of fluorine as sodium fluoride per kg BW for years, although a similar dose may induce dental lesions in puppies (Greenwood 1956). In a study, the daily intake of 10–24 mg F/kg BW for 2–8 months in pups after weaning resulted in brown discoloration, erosions, and fissures in the enamel of permanent molars and incisors together with bone lesions (Grancher et al. 1988). The lethal dose of sodium fluoride for dogs is 47 mg/kg BW. Fluoride concentration as high as 460 mg/kg in dog food (wet basis), given for 2 years neither produced any bony lesions nor any adverse effects on reproductive performance (Shellenberg et al. 1990). However, in another study, it was observed that threshold F concentration for effects on structural and functional changes in gastric mucosa was approximately 1 m mol/L, and maximum or near-maximum effects were caused by 10 m mol F/L (Whitford et al. 1997).
5.1.3 Wild Animals
The behavior and feeding patterns of wild animals in captivity differ from those in wild conditions. Some wild animals have a large territory where fluoride concentration in water and dietary substances may vary significantly. Hence, setting a tolerance level on the basis of experimental studies may be erroneous. To date, knowledge of fluoride toxicity in wild animals under natural conditions is largely based upon dental and bony changes and bone fluoride concentration recorded in dead animals.
In general, herbivores, particularly those grazing grasses and small vegetation, are more susceptible than leaf and fruit eaters and carnivores. Grasses and other small plants growing on soil containing a high fluoride concentration or in industrial fluoride polluted areas often contain a higher fluoride concentration than leaves of tall trees or fruits grown in the same area. Wild herbivores living in geographic areas where cattle and buffaloes suffer from chronic fluorosis also exhibit signs of fluorosis, suggesting they are equally sensitive to fluoride. Carnivores suffer less as they occupy the topmost position in the food pyramid and consume soft tissues of animals which have low F concentration. In a study in Britain, Walton (1984) observed that skeletal fluoride concentration in small mammals inhabiting the vicinity of an aluminum smelter was increased, but no increase in bone fluoride concentration was recorded in red foxes (Vulpes vulpes). Red foxes are omnivorous and small rodents comprise a major part of their diet. Fecal examination of red foxes revealed that skeletal parts of the prey pass into feces undigested, which may be the possible reason behind their low bone F concentration.
Cervids are highly susceptible to natural and industrial fluorosis. Dental fluorosis due to industrial fluoride pollution has been reported in several deer species including mule deer (Odocoileus hemionus hemionus), white-tailed deer (Odocoileus virginianus), and black tailed deer (Odocoileus hemionus columbianus) (Vikoren and Gudbrand 1996), although the susceptibility to fluoride toxicity varies with species. Roe deer (Capreolus capreolus) are reported as a sensitive bioindicator of industrial fluoride pollution (Kierdorf et al. 1993). In almost all deer species, dental lesions are more common than bony lesions in both natural and industrial fluorosis. Moreover, dental lesions in deer skulls collected from the forest areas where fluorosis was prevalent in domestic ruminants, very closely resembled those observed in fluorotic cattle. The pathological changes in mandibular bone including periodontal breakdown, periostitis, ostitis, and chronic osteomyelitis, resulted in early tooth loss and a decrease in the life expectancy of wild deer (Schultz et al. 1998). High fluoride concentration in bone and teeth of these skulls supported the hypothesis that the dental and bony changes were linked with fluorosis.
Fluoride concentrations in normal deer vary from 160 to 560 ppm in mandibles and 134–152 ppm in antlers (Newman and Yu 1976). In the bone of deer suffering from fluorosis, fluoride concentration may reach up to 7000 ppm. In Argentina, wild red deer (Cervus elaphus) developed severe dental lesions after a volcanic eruption and bone fluoride levels reached up to 5175 ppm (Flueck and Smith-Flueck 2013). The source of excess fluoride was ingestion of tephra (volcanic ashes) deposited on the herbage. Fluoride accumulation is perhaps more rapid and extensive in antlers than bone of the same animal (Suttie et al. 1985). However, a study revealed that bone and antler fluoride concentration in fluorotic deer did not differ significantly and authors concluded that antler material remains in equilibrium with fluoride elsewhere in the skeleton (Walton and Ackroyd 1988).
A population of eastern grey kangaroos (Macropus giganteus) living close to an aluminum smelter in Australia exhibited clinical signs of lameness. A diagnosis of fluoride toxicity was given after detailed gross and radiographic examination of bone and teeth and fluoride analysis in bone (Clarke et al. 2006).
Minks have high fluoride tolerance. Captive minks raised only for pelts are usually euthanized at 7 months of age. They can tolerate up to 100 ppm fluoride in their feed on wet weight basis or 270 ppm on dry weight basis without any adverse effects on pelt quality, growth rate, and appearance of bony or dental lesions. However, for breeding stock this level cannot be recommended as bony and dental lesions may appear after 7 months ultimately affecting their reproductive performance. The tolerance level of F in feed for breeding stock may be fixed as 50 ppm on wet weight basis or 135 ppm on dry weight basis (Shupe et al. 1987).
Wild rodents such as the cotton rat (Sigmodon hispidus) are also susceptible to fluoride toxicity and a high incidence of dental lesions along with high bone fluoride concentration was reported in cotton rats living in areas where land-treatment of petroleum waste products is practiced (Paranjpe et al. 1994; Rafferty et al. 2000).
In wild birds, short-lived, seed-eating birds accumulate more fluoride in bone than long-lived omnivore species. Bone fluoride concentration in wild birds living in the vicinity of fluoride-polluted areas may reach as high as 11,000 µg/g (Henny and Burke 1990).
5.1.4 Poultry and Other Birds
The fluoride tolerance of poultry is much higher than that of mammals (Shupe 1969). Spontaneous cases of fluorosis are rare in chicken, turkeys, and other poultry. Higher tolerance may be attributed to dietary factors, short life span, and some other unknown physiological factors. In poultry, fluoride is accumulated in high concentrations in bone without showing any pathological changes.
Chickens can tolerate up to 350 ppm F in their diet with no adverse effect on growth (NRC 1960). When the fluoride concentration in the diet of chickens reaches 700 ppm or more, growth is retarded, but there is no effect on egg production (Halpin and Lamb 1932). A high concentration of fluoride may be found in the egg yolk of poultry raised on a high F diet. Calcium and phosphorus concentration in bone decreases and generalized signs of lameness, leg weakness, decreased weight gain, decrease in feed consumption, and feed efficiency may be observed in advanced cases.
Turkeys are even more tolerant than chickens and can tolerate up to 300 to 400 ppm F in the diet (Phillips et al. 1955; NRC 1960). A decrease in appetite, feed efficiency, and weight gain were major clinical findings recorded in an experimental study when turkeys were maintained on a ration containing 400–800 ppm F for 16 weeks (Anderson et al. 1955). The postmortem changes in these turkeys included thickening of the duodenum mucosa suggesting local irritating effects of excess fluoride intake.
The Adelie penguin (Pygoscelis adeliae) is a unique bird that does not show any gross or radiographic symptoms of skeletal fluorosis despite having an exceptionally high bone fluoride concentration (up to 9000 µg/g). The major diet of the Adelie penguin is krill, whose average body fluoride concentration is about 1232 µg/g. The krill body is covered with a chitinous crust which accumulates a high fluoride concentration (as high as 5000 µg/g). However, high chitin concentration in the bone of penguins is assumed to prevent skeletal fluorosis, as chitin can combine with fluoride preventing abnormal bone mineralization (Yin et al. 2010).
5.1.5 Insects and Other Invertebrates
The susceptibility of insects and other invertebrates to fluoride can be very well understood by the fact that sodium fluoride, fluoroalcohols, and fluorophosphates were widely used for control of insects, cockroaches, and poultry lice in the past before the advent of DDT. Sulfuryl fluoride is an odorless, colorless, noninflammable, noncorrosive gas that was first registered as an insecticide and rodenticide in year 1959, but is still being used in some countries as anti-insecticide fumigation. Industrial fluoride pollution is assumed to have devastating effects on the silkworm and honeybee industries (Weinstein and Davison 2004). In addition, fluoride pollution has a significant impact on the overall insect population in industrial vicinities, but the absolute effect of fluoride is difficult to assess due to simultaneous exposure to other pollutants. Ground-dwelling invertebrates also accumulate fluoride, but the impact of excess fluoride on their growth and population is not well established and research findings are contradictory. In an experimental study, average whole-body fluoride concentration of Dikerogammarus villosus, a species of amphipod crustacean was 27.6 µg/g dry weight which increased up to 16, 994 µg/g 72 h after exposure to 10 mg F/L. The authors therefore, concluded that the capacity to rapidly accumulate a very high concentration of fluoride may be the reason behind its high sensitivity to fluoride (Gonzalo et al. 2010).
5.1.6 Fish and Other Aquatic Animals
In general, aquatic animals (invertebrates and fish) living in hard or seawaters are more tolerant to fluoride than those living in soft water with low ionic content. A high concentration of calcium and chloride in the water decreases the bioavailability of fluoride ions, thereby increasing fluoride tolerance (Camargo 2003). Moreover, large body size within a given aquatic species is associated with greater fluoride tolerance in both soft-water and marine animals (Camargo 2004). This happens perhaps due to a higher fluoride elimination or immobilization rate in larger individuals (Sigler and Neuhold 1972). The LC50 values for fluoride for freshwater, brackish, and marine invertebrates at different stages of development, exposure time, and temperature ranges from 10.5 to 308 mg F/L (WHO 2005).
In addition to fluoride concentration in an aquatic medium, susceptibility to fluoride toxicity in aquatic animals also depends upon the exposure time and water temperature. Fluoride concentrations in unpolluted freshwater usually range from 0.01 to 0.3 mg F/L, whereas in unpolluted seawater it ranges from 1.2 to 1.5 mg F/L (Camargo 2003). Naturally elevated levels of fluoride may be found in regions with high geothermal or volcanic activity or industrial units emitting fluoride-rich fumes or effluents. An increase in water temperature is associated with increased risk of fluoride toxicity, possibly due to an increase in metabolic rate and hence uptake of fluoride ions from the aquatic medium (Sigler and Neuhold 1972). Several experimental studies have also demonstrated a negative correlation between chloride ion concentration in water and susceptibility to fluoride toxicity in aquatic animals (Neuhold and Sigler 1962; Pimentel and Bulkley 1983).
Fluoride tends to accumulate in the exoskeleton of aquatic invertebrates and in the bony tissues of fish. This may be helpful to aquatic animals dwelling in polluted water by enhancing fluoride elimination from body circulation and increasing hardness of the exoskeleton and bone. Fluoride accumulation in soft tissues is low in most aquatic invertebrates and fish. Nevertheless, several aquatic invertebrates appear highly sensitive to fluoride toxicity. Juvenile and young stages are more sensitive than adults (Aguirre-Sierra et al. 2011). Industrial fluoride pollution to freshwater reservoirs has been claimed responsible for the rapid decline in the population of several invertebrates including snails, unionid mussels, and shrimp species in several water reservoirs around the world.
Among freshwater or soft water invertebrates and fish, net-spinning caddisfly larvae and upstream-migrating salmon are the most sensitive to fluoride. The growth and migration of these freshwater organisms are adversely affected when fluoride concentration reaches 0.5 mg/L or more. Hence, the safe fluoride concentration recommended for protection of all stages of freshwater life against the adverse effects of fluoride is 0.2 mg/L or less (Groth 1975; Damkaer and Dey 1989). In the case of hard water with high ionic content, safe levels of fluoride could be increased up to 1.0 to 1.5 mg F/L (Camargo 2003). Rainbow trout (Oncorhynchus mykiss) and water flea (Daphnia magna) are widely used in experimental fluoride toxicity studies. Sensitivity to fluoride toxicity has also been reported in freshwater-dwelling unionid mussels, Alasmidonta raveneliana, and several other invertebrate animals (Camargo and Tarazona 1990; Keller and Augspurger 2005). A laboratory study revealed that 96 h LC50 and 240 h NOEC values for Dikerogammarus villosus (also known as the killer shrimp, a species of amphipod crustacean native to the Ponto-Caspian region of Eastern Europe) is 5.8 and 0.95 mg F/L, respectively (Gonzalo et al. 2010).
5.2 Factors Affecting Fluoride Tolerance
Fluoride tolerance in domestic animals is governed by several factors including solubility of the fluoride compound, animal species, climatic conditions, and management practices. The amount of fluoride ingested, duration of fluoride ingestion, age at the time of ingestion, physiological status of the animal, nutrition, general health status, stress, exposure to health hazards, immunity, and individual response to fluoride are some other factors modulating the toxic effects of fluoride (Shupe 1980).
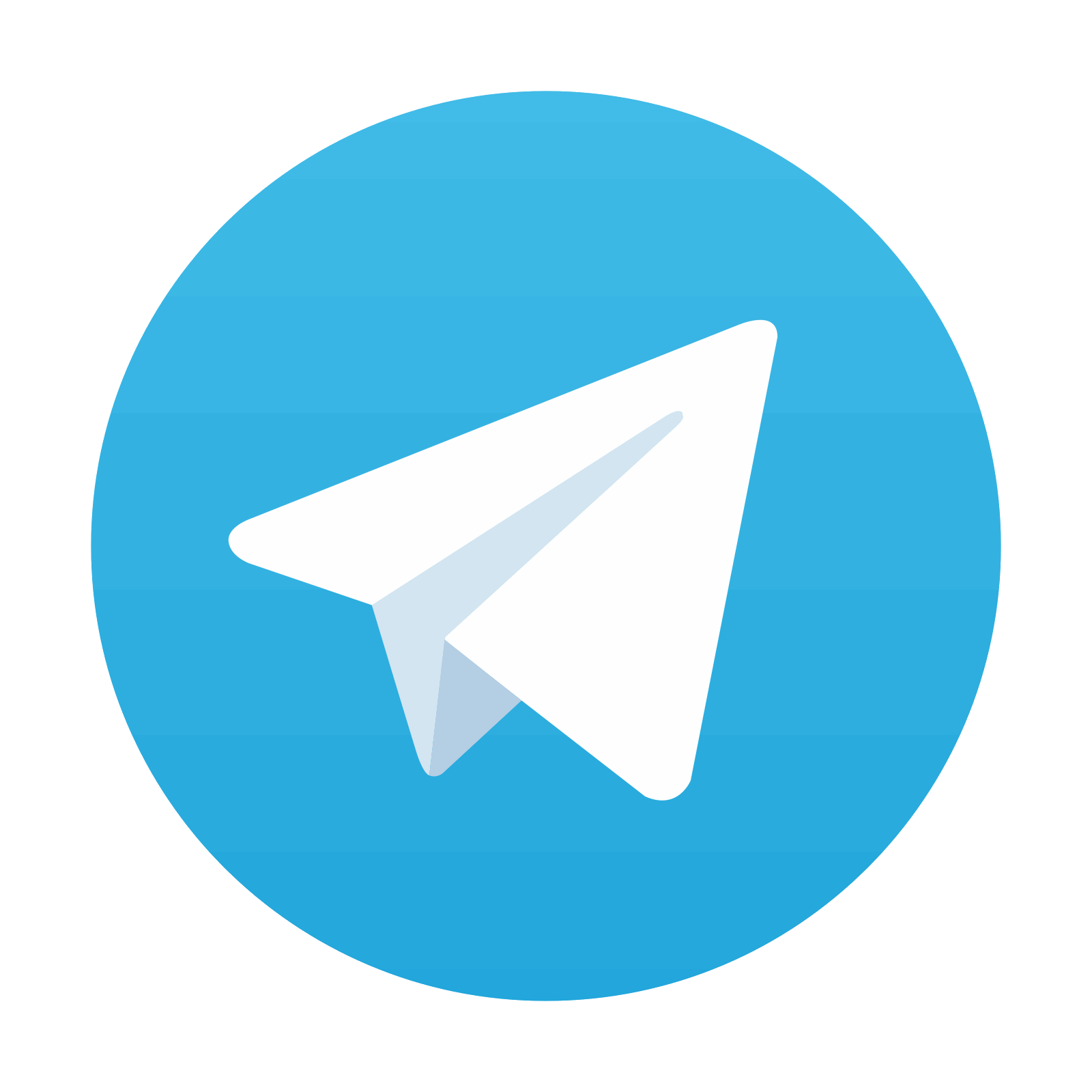
Stay updated, free articles. Join our Telegram channel

Full access? Get Clinical Tree
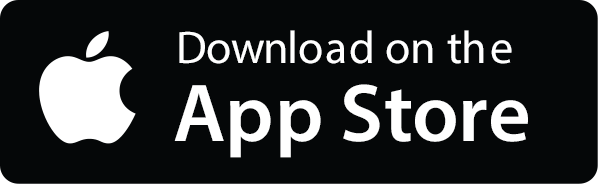
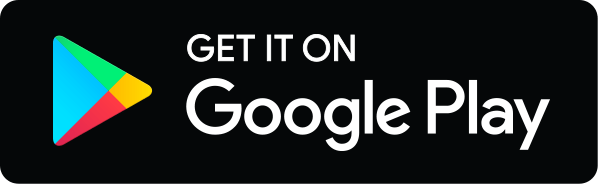