Acid–Base Disturbances
Acid–base disturbances are frequently encountered in veterinary medicine as a result of illness. This chapter provides a basic understanding and recognition of acid–base problems.
Terminology
The terms used to describe changes in blood pH and blood gas concentrations are presented in Table 57-1.
Physiology
Buffers. Buffers act to resist changes in pH and typically exist as a buffer pair—a weak acid (proton donator) and its conjugate base (proton acceptor). There are four major buffers in the extracellular fluid (ECF) compartment. Hemoglobin is an important ECF buffer and accounts for most nonbicarbonate buffering. The red blood cell membrane is permeable, allowing hemoglobin, an intracellular molecule, to have an important role in ECF buffering. Plasma proteins such as albumin, immunoglobulins, and hormone-binding proteins also contribute to ECF buffering. Phosphate, due to its low concentration in the ECF, contributes little to ECF buffering. The bicarbonate buffer system is the most important, but does not fit the classic buffer model. Although bicarbonate can accept a proton and is considered a conjugate base, carbon dioxide (CO2) has no proton to donate. Carbon dioxide, though, when dissolved in an aqueous solution reacts chemically with water to produce carbonic acid (H2CO3), which rapidly disassociates to form bicarbonate and free hydrogen ions (H+).
CO2 + H2O ↔ H+ + HCO3−
In this reaction, carbon dioxide does act as a weak acid and bicarbonate acts as its conjugate base. The equilibrium of this reaction may shift by altering either the concentration of CO2 or HCO3−. In health, PCO2 is held constant by changing the body’s rate and depth of respiration to match the body’s production of CO2. Acid, continually produced as a metabolic by-product, enters the ECF compartment and consumes plasma bicarbonate. In health, bicarbonate is reabsorbed in the renal tubules and regenerated via the excretion of titratable acids that are buffered by nonbicarbonate buffers and ammonium excretion.
Ion Shifts. Ion shifts between the ECF and the ICF compartments are another mechanism that helps maintain pH homeostasis. The most clinically important ion exchange is potassium for hydrogen (K+ ↔ H+). The influx of hydrogen ions diffusing into the cell is exchanged for intracellular potassium moving out of the cell. In metabolic acidosis, hyperkalemia can occur because the intracellular concentration of potassium is significantly greater than the plasma concentration, and the shift of potassium to the ECF compartment in exchange for the increased hydrogen ions causes increased plasma K+ concentrations. The occurrence of metabolic acidosis due to diarrhea may cause hypokalemia if severe dehydration is present. The loss of ECF volume causes activation of the renin–angiotensin–aldosterone system (RAAS). This causes an increase in blood pressure, the renal tubular reabsorption of sodium, chloride, and water and the renal tubular excretion of potassium. Hypokalemia with metabolic acidosis can be associated with severe body potassium depletion, which is important clinically.
TABLE 57-1. Terms used to describe acid–base abnormalities and blood gas concentrations
Term | Description |
Acidemia | Acidic blood |
Acidosis | Process that causes pH to decrease |
Alkalemia | Alkaline blood |
Alkalosis | Process that causes pH to increase |
HCO3− | Bicarbonate (mmol/L) |
Hypercapnia | Increased CO2 in the blood |
Hypocapnia | Decreased CO2 in the blood |
pH | Measure of the concentration of hydrogen ions ([H+]) in plasma |
PCO2 | Partial pressure of carbon dioxide in blood (mm Hg) |
PO2 | Partial pressure of oxygen in blood (mm Hg) |
TCO2 | Total carbon dioxide dissolved in blood; on the serum biochemistry profile and can be interpreted as an indirect measurement of [HCO3−] |
Brackets [] | Concentration |
Categories
The four primary categories of acid–base disturbances are summarized in Table 57-2.
Metabolic Acidosis. Metabolic acidosis is the most common acid–base disturbance in small animals. It is characterized by a decrease in pH, a decrease in the [HCO3−], and compensatory hyperventilation to decrease PCO2. Metabolic acidosis due to an increased accumulation of endogenous or exogenous metabolic acids is frequently encountered in small animal medicine and is often referred to as an anion gap metabolic acidosis. The causes of metabolic acidosis are listed in Table 57-3.
TABLE 57-2. Primary acid–base disturbance and compensatory change

TABLE 57-3. Select causes of metabolic acidosis
Non-anion gap |
Diarrhea |
Renal tubular acidosis |
Ammonium chloride administration |
Methionine sulfate administration |
Dilutional (rapid infusion of intravenous saline) |
Anion gap |
Ketone accumulation |
Azotemia |
Lactic acid accumulation |
Toxins |
Ethylene glycol |
Salicylate |
Paraldehyde |
Lactic acid results from anaerobic tissue metabolism and can occur in any condition (dehydration, shock, cardiac disease) that causes poor tissue perfusion, which decreases oxygen delivery to tissues. Excessive lactic acid production may also occur due to grain overload and increased muscle activity (vigorous exercise, seizure activity) that leads to anaerobic metabolism. Some patients with malignant neoplasms develop lactic acidosis.
Ketoacidosis occurs in states of increased lipid metabolism (diabetes mellitus, starvation). Urine ketones should be positive on a urine dipstick since increased ketones are detected in the urine before they are increased in the blood.
Exogenous compounds that increase metabolic acids are ethylene glycol, salicylates, and paraldehyde. The increase in either endogenous or exogenous acids causes an increase in the anion gap (see below).
The loss of bicarbonate with severe diarrhea is a common cause of metabolic acidosis. In addition to causing significant loss of bicarbonate, there is loss of electrolyte-rich (potassium, sodium, and chloride) fluid, leading to severe dehydration and electrolyte derangement. Animals with metabolic acidosis due to gastrointestinal loss of bicarbonate may be hyperchloremic and hypokalemic. Hypokalemia results from potassium loss in the diarrheal fluid and is exacerbated by increased aldosterone production from RAAS activation, causing increased renal excretion.
Other causes of metabolic acidosis in small animals include renal disease (renal failure and renal tubular acidosis), hypoadrenocorticism, rapid intravenous infusion of saline, and hyperphosphatemia (see Table 57-3).
Anion Gap. The calculation of the anion gap is based on the law of electroneutrality. This law states that there are an equal number of both positive and negative charges in an aqueous solution (Fig. 57-1 ). Sodium is the most important cation and is present in plasma in high concentrations. Potassium, magnesium, calcium, and hydrogen ions are all contributing cations but are in low concentrations, and with the exception of potassium, not all forms are measured during routine laboratory analysis. The chief anions in plasma are chloride and bicarbonate. The remaining negatively charged particles are sulfates, phosphates, organic anions, and proteins, and are also not routinely measured. The formula uses only sodium and potassium as the total measured cations and bicarbonate and chloride as the total measured anions. The difference between cations (Na+ + K+) and anions (Cl− + HCO3−) represents a portion of the unmeasured anions that comprise the anion gap (Fig. 57-2 ). Figures 57-1 to 57-3b should help to clarify this concept. Calculating the anion gap is a diagnostic tool that may assist in determining the underlying cause of the metabolic acidosis.
FIGURE 57-1. Law of electroneutrality. The number of positive charges equals the number of negative charges in plasma.

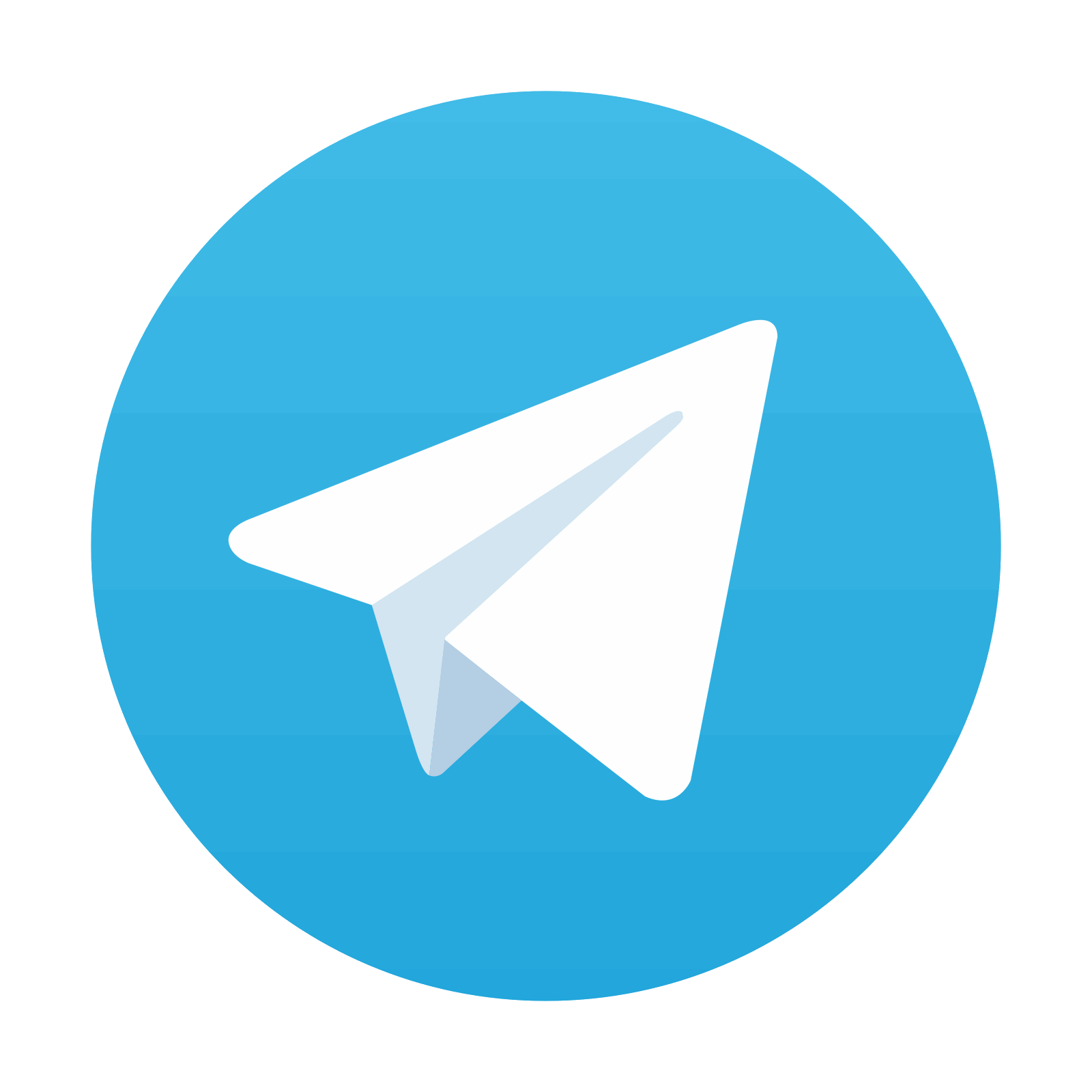
Stay updated, free articles. Join our Telegram channel

Full access? Get Clinical Tree
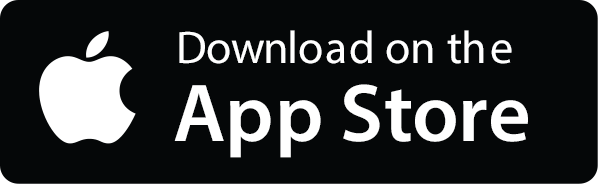
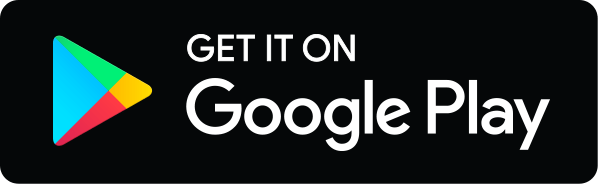