TABLE 11-2 Equine Muscle Fiber Types and Their Characteristics

Speed, like pulling or lifting a heavy load, requires strength. Strength requires large muscles, whereas prolonged activity requires oxygen-utilizing ability, which requires small muscle fibers—not the strength provided by big muscle fibers. Increased muscle size for strength is provided by an increase in both the number and size of muscle fibers, both of which are increased by genetics and strength (speed and weight) training; whereas increased oxygen-utilizing capacity for endurance is influenced by genetics and cardiovascular training. Thus, a horse may have the genetic ability to be good at a particular type of activity, and appropriate training will increase this ability.
The fast-twitch (FT) muscle fibers are large fibers that have high strength but low oxidative capacity because the fiber is high in myofibrils rather than mitochondria, where oxygen is utilized. The reverse is the case for the small slow-twitch (ST) muscle fibers. Because of their large size, oxygen is less able to diffuse into the FT fibers, but they are low in fat, which requires oxygen for its use, and are high in glycogen, which doesn’t require oxygen for its use. Thus, the FT muscle fibers can produce energy and high power very rapidly anaerobically, and thus high power, but not for very long before fatigue occurs, since lactic acid is produced and little energy can be produced from glycogen as compared to fat.
These are the reasons sprinters and weight lifters or pullers have high strength but rapidly fatigue, and that the marathoner has low strength but endurance. Regardless of the muscle fibers present, as speed or exertion increases, the nerves activate more muscle fibers, so that at a walk only 10% of muscle fibers are used and all are ST, at a trot 50% of fibers are used and include all the ST and many FTH fibers, and at a full gallop or exertion 100% of all three fiber types are used.
Fatigue, and as a result a decreased ability to continue muscular activity, occurs because of numerous factors whose contribution to causing fatigue varies in different situations. These include the following:
The greater the amount of glycogen in the muscle and the slower its rate of utilization, the longer before fatigue occurs. Three procedures to increase muscle glycogen content are: (1) training to increase physical conditioning, (2) feeding diets high in either fat or soluble carbohydrates, such as starch, and (3) glycogen loading. Each of these three methods is described in the following sections of this chapter.
DIETS AND SUPPLEMENTS FOR ATHLETIC PERFORMANCE
The nutrients most needed and likely to be of benefit for the exercising horse are those lost in sweat and those used to provide energy. Those lost in sweat can be best provided by always ensuring that good-quality water and salt (sodium chloride) are easily available at all times. The amount of additional salt needed to replace lost sweat, and the effects, prevention, and treatment of water and electrolyte deficits, are described later in this chapter.
The increased amount of dietary energy needed for athletic training and performance can be provided by increasing the energy density of the diet. This can be accomplished by increasing its fat, protein, or starch, and decreasing its fiber content. Because cereal grains are high in starch and low in fiber, and forages are the opposite, dietary intake of starch is increased and fiber is decreased by feeding a relatively high-grain and, therefore, low-forage diet. However, forage should constitute at least one-half of the weight of the diet eaten. If this amount of forage is not being consumed, the amount of grain fed should be reduced until it is. This amount of forage is needed in the diet to decrease the risk of laminitis or founder; gastrointestinal disturbances, such as colic and diarrhea; and behavioral problems in stabled horses, as described in Chapter 20.
Increasing protein and fat in the diet also increases its energy density, although protein appears to be the least preferred, and fat a preferred, source of dietary energy for athletic performance. As a result, as described in the following sections, a high-fat diet appears to be beneficial, and a high-protein diet detrimental, for both sprint and endurance type of activities.
Increased energy production and utilization needed for exercise increases the need for vitamins A, E, B1 and folacin. Increased amounts of vitamin E and selenium are needed to prevent tissue damage caused by the increased oxidation needed for increased energy production. The additional amount that may be of benefit can be provided by adding to the diet 1 mg of selenium, or 2 mg of sodium selenite, and 1000 IU of vitamin E per horse daily. Additional selenium can instead be provided by using as the only salt mixes available, ones containing selenium. The additional amount of all of the vitamins that may be needed and beneficial for the equine athlete may be best provided by giving a balanced vitamin supplement. One recommended and used that appears quite good is given in Table 3–5. Whether this or other vitamin preparations are given, the amount should be sufficient to supply the horse’s requirements as given in Appendix Table 3.
In addition to a good, balanced vitamin supplement and selenium, adding probiotics, or live yeast cultures, to the diet for horses being used for athletic performance may be beneficial. Although the benefit of feeding probiotics is controversial, some studies in horses showed these products are of benefit for athletic performance. Because of this, and the lack of any harm from doing so, adding a good probiotics product to the equine athletes’ diet may be worthwhile. Adding fat or oils to the diet, as discussed previously, and administering sufficient sodium bicarbonate (baking soda) at the proper time prior to sprint-type exertion, but not in conjunction with other types of activity, as discussed later in the section on “Feeding and Supplements Before Athletic Performance,” may also be beneficial.
In contrast, there is no indication of any need for, nor any benefit from, giving “blood builders” or hematinics, such as iron or vitamin B12, or from giving vitamin C (ascorbic acid). For a discussion of all of these vitamins and minerals, see the sections on each of these nutrients in Chapters 2 and 3. In addition, in contrast to occasional anecdotal reports, like giving hematinics or vitamin C, there are no good, controlled, or convincing studies demonstrating increased performance or benefit from giving numerous substances promoted and sold as aids to athleticperformance. These substances include: (1) MSM (methyl sulfonyl methane, dimethyl sulfone or DMSO2), the oxidation end product of dimethylsulfoxide or DMSO (but without DMSO properties), (2) octacossanol (an alcohol present particularly in wheat germ oil), (3) the enzyme superoxide dismutase (SOD, which is probably destroyed in digestion and absorption), (4) gamma hydroxybutyrate (a free-radical scavenger), (5) gamma oryzanol (a ferulic acid ester proposed as a natural antioxidant that prevents membrane damage during anaerobic exercise), (6) bioflavanoids (a naturally occurring antioxidant that may enhance vitamin C availability), (7) inosine and carnitine (dietarily nonessential amino acids), (8) pangamic acid (sometimes referred to as vitamin B15 although it is not a vitamin or even a nutrient), and (9) drugs such as furosemide, amphetamine, and nandrolone. Most of these substances are promoted based on appealing rationales and many testimonials, but not on supporting data from properly conducted trials. When they are evaluated in controlled studies, these and numerous other substances fail to demonstrate any benefit. For example, when the performance times of 58 Standardbreds run 160 times without furosemide and 232 times with furosemide were compared, there was no significant difference although performance times averaged 0.14 second slower when furosemide was given.
Both carnitine and DMG have been used as nutritional supplements for athletes in the hope of reducing lactate accumulation and delaying fatigue. Both, when added to the horses’ diet, have been shown to lessen the exerciseinduced increase in plasma lactate concentration but neither had any effect on performance. In another study there was no improvement in oxygen transport or reduction in plasma lactate concentration after exercise in horses fed DMG. It was concluded that DMG had no beneficial effects on cardiac or respiratory function or lactate production in the exercising horse.
Dietary Protein for Athletic Performance
There are several disadvantages to using protein for energy. Protein is generally an expensive source of dietary energy compared to carbohydrates; e.g., soybean meal provides less energy than corn, yet costs several times more because it is several times higher in protein content. From 3 to 6 times more heat is produced in the utilization of protein for energy than in the utilization of carbohydrates or fats. This contributes to excess body heat and increased sweat-loss-induced water and electrolyte deficits during exercise. Protein utilization for energy also increases water and electrolyte losses in the urine necessary to excrete nitrogen. The nitrogen removed from protein, when protein is used for energy, is excreted in the urine as urea and ammonia. The increased urinary ammonia in the horse’s stall, if not well ventilated, may contribute to increased respiratory disease or impairment (as described in the section on “Stable Ventilation” in Chapter 9).
No studies have shown any benefit, and some have shown detrimental effects during athletic performance from feeding horses diets containing substantially more protein than needed, as given in Appendix Table 1. In one study, heart rate, respiratory rate, and sweating were higher in horses receiving a high-protein diet and performing submaximal long-duration activity. In another study, excessive protein intake was found to increase water intake and urine production, and to decrease the efficiency of digestible energy utilization in horses going 50 miles (80 km) at a medium trot or slow lope. At both high speeds (running at 20 mph, or 9 m/sec) and moderate speeds (fast trot, slow canter, or lope at 11 mph, or 5 m/sec), blood glucose concentration fell more and over a longer period in horses fed a high-protein diet (25%) than in those fed either a high-carbohydrate or a high-fat diet. It is recommended that the protein content of the equine athlete’s total diet dry matter be between 10 and 16%, to thus meet, but not greatly exceed, needs.
Dietary Fat for Athletic Performance
In contrast to a high-protein diet, a high-fat diet provides several benefits for athletic performance. These include:
As a result of these effects, high-fat diets have been shown to enhance both aerobic and anaerobic performance activities and to delay fatigue.
A high-fat diet (15% added soy oil) appeared to be better than either a high-starch (40%) or a high-protein (25%) diet for both high-speed (running at 9 to 10 m/sec) or moderate-speed (fast trot, slow canter, or lope at 5 m/sec) exercise. On the high-fat diet, the blood glucose concentration decreased less and for a shorter duration. At high speed, muscle glycogen use and plasma lactate concentration were both substantially lower than when the horses were consuming the high starch, low-fat (3%) diet. Whereas in two other studies muscle glycogen levels before exercise were substantially higher in horses fed high-fat diets (65% grain with versus without 10% added animal fat), and were similar following high-speed, short-duration activity. Thus, there was greater glycogen utilization during this activity which, as the investigators suggest, may enhance performance that requires primarily glycogen utilization for anaerobic energy production. This suggestion was supported in other studies where it was found that horses fed high-fat diets ran faster at a constant heart rate, and faster before their plasma lactate concentration began to increase sharply and reached 4 mM/L, than did horses fed non-fat supplemented diets. When Thoroughbred horses were fed a fat-supplemented diet, 14 of 15 had faster race times, and when in moderate or moderately high body-fat condition, utilized significantly more glycogen than when in moderately low body-fat condition. These studies emphasize the benefit of both fat supplementation and maintaining the horse at moderate to moderately high body-fat condition for maximum Thoroughbred race performance.
In addition to anaerobic activity, increased muscle glycogen in horses fed high-fat diets has also been shown to be effectively utilized for aerobic or endurance-type activity. Horses fed a diet with 10.5% added fat were able to trot for about 35 minutes before their heart rate reached 160/ min; this heart rate was reached after only 20 minutes in horses fed a diet with no added fat. Cutting horses fed high-fat diets were found to work harder than those fed a conventional diet. Full metabolic adaptation to a high-fat diet has been shown to be achieved in 11 weeks, but not 6 weeks. Fat-supplement sources, and procedures for feeding them, are described in Chapter 4.
FEEDING AND SUPPLEMENTS BEFORE ATHLETIC PERFORMANCE
Prior to athletic performance, procedures to increase muscle glycogen, referred to as glycogen packing or loading, have been utilized, most often in people. This is accomplished in people by decreasing or stopping exercise and increasing carbohydrate intake during the 2 to 3 days before a race. An even greater increase in muscle glycogen content, but one no longer recommended because of numerous problems associated with it, can be obtained by first decreasing muscle glycogen by decreasing carbohydrate intake while continuing strenuous anaerobic exercise for 2 to 4 days before beginning the glycogen loading phase. Similar regime’s increase muscle glycogen in horses, but, in contrast to people, appear to provide little or no benefit. However, impaired racing performances due to low muscle glycogen levels, and improvement in performance by increasing nonfiber carbohydrate intake (feeding an additional 2 lbs, or 0.9 kg of corn daily) for several days before a race, has been reported. Too much of an increase in grain intake, however, may cause founder and predispose to exertion myopathy. These risks are decreased by increasing grain intake slowly and by dividing it into several small meals.
In contrast to a possible benefit from increased grain intake during the last few days and up to 4 to 5 hours before a race, increased grain intake within a few hours or less before a race is detrimental. Blood glucose and insulin concentrations peak 2 to 3 hours after the horse eats grain, but not after forage. These effects decrease fat utilization and worsen the fall in blood glucose during exercise, which decreases both endurance and speed. The type of cereal grain or forage fed doesn’t appear to matter, as there is no difference in these effects between different cereal grains (barley, corn, oats, and a sweet feed were evaluated) or between chopped, cubed, pelleted, or long-stemmed hay. In contrast to feeding grain within less than 4 hours before a race, feeding grain before this period, and feeding small amounts of grain periodically during endurance-type activity, is not only not harmful but may extend the time before fatigue or exhaustion occurs.
Feeding 3 to 5 lbs (1.5 to 2 kg) of grain 4 to 5 hours before, and allowing free access to forage and water up until endurance-type activity begins, is recommended. The ingested grain will provide needed energy and, by 4 to 5 hours, the increase in blood glucose and insulin concentrations it induces will have returned to normal; they will therefore not be present to decrease fat utilization or exercise. Water and forage intake maximizes the amount of water and electrolytes in the gastrointestinal tract, and thus their availability to replace those lost during prolonged exercise. These are important for prolonged submaximal activity, as the horse’s endurance capacity is limited by their loss. Low-forage-high-grain diets, which decrease gastrointestinal water and electrolyte storage, have been found to correlate with increased risk of failure in endurance racing.
In contrast to allowing and encouraging water and forage intake before endurance activity, not allowing forage intake for a period prior to all types of physical exertion has been recommended to decrease gastrointestinal fill and, therefore, the weight the horse must carry. This amount may be quite substantial, as the horse’s gastrointestinal tract can hold an amount equal to as much as 25% of its body weight. Thus, gastrointestinal fill is certainly an added weight handicap for the horse, which offsets the benefit it provides as a storage source of water, electrolytes, and nutrients. The benefit of this nutrient storage source, however, offsets the detriment of added weight for endurance-type activity, but not for short-duration maximum- or near-maximum intensity activity. For races of several minutes duration or less, there is no need for or time to absorb nutrients from the gastrointestinal tract.
It has also been theorized that feeding before exercise may be detrimental because it will increase blood flow to the gastrointestinal tract and away from the skeletal muscle. However, it has been shown that although feeding before exercise did result in a higher blood flow to the gastrointestinal tract during exercise, blood flow to the skeletal muscle was also higher, not lower, as compared to ponies fasted for 24 hours prior to exercise. The fed ponies’ heart rates and cardiac outputs during exercise were also higher than in the fasted ponies.
Administering sodium bicarbonate (baking soda) prior to maximum exertion of a few minutes or less, such as races of 1.5 miles (2400 m) or less, may be beneficial. The sodium bicarbonate buffers the high amount of lactic acid produced with the high amount of anaerobic glycolysis this type of activity requires (Table 11-1). Buffering the lactic acid produced allows greater glycogen utilization to occur, which permits greater exertion and prolongs fatigue, and thus enhances performance. These effects and benefits have been shown in several studies. However, the proper amount of sodium bicarbonate must be given at the proper time to be beneficial and not harmful, and it is illegal to give it at many tracks and events.
A dosage of 0.3 g/kg body weight has only a moderate effect, and administration of 0.4 g/kg only 1 hour before racing has been shown to not improve performance. Peak blood buffering capacity does not occur until 2 to 6 hours, after administration of sodium bicarbonate by stomach tube. A dosage of 0.4 g/kg has been found to be more effective than 0.2 g/kg, and as effective as 0.5 g/kg, but with less detrimental effect. Giving 1 g/kg results in a major and potentially deleterious increase in plasma sodium and decreases in plasma potassium, chloride, and calcium concentrations. These electrolyte concentration alterations are the same as those induced by heat stress, sweating, and furosemide administration, and, therefore, a combination of these factors would exacerbate these potentially deleterious alterations.
Thus, 0.4 to 0.6 g/kg body weight of sodium bicarbonate (200 to 300 g, 7 to 10 oz or about one half of a 1-1b box of baking soda/1100-lb or 500-kg horse), dissolved in several quarts (liters) of water and administered by stomach tube 3 to 6 hours before an event requiring primarily anaerobic type exertion (Table 11-1), may enhance performance. More than this amount should not be given, and it should not be given before primarily aerobic or endurance-type activity (Table 11-1); doing so is of no benefit and is likely to be harmful because of the electrolyte alterations it induces. Adding sodium bicarbonate to the feed instead of giving it by stomach tube may not be effective. At least in one study, horses would not eat the amount needed when it was added to 4.6 lb (2.1 kg) of grain-mix.
Red blood cell transfusions (blood doping, boosting, or packing) is another procedure that has been used to try to enhance athletic performance. Although it may increase human endurance, its benefit is slight, and in dogs, it appears to be detrimental, causing increased blood viscosity, which results in increased resistance to blood flow, decreased cardiac output, and decreased blood return to the heart, which were greater the higher the dogs hematocrit. Although the effect of red blood cell transfusions on horses’ athletic performance has not been determined, it quite likely would be even more detrimental for them than for dogs, because splenic contraction in horses during exercise results in a high hematocrit and blood viscosity.
WATER AND ELECTROLYTES FOR ATHLETIC PERFORMANCE
Heat Production, and Water and Electrolyte Losses
About 75 to 80% of energy used in the body is given off as heat. Energy utilization and, therefore, heat production greatly increase during exercise. Even at gentle exercise (a trot, canter, or lope at 9 to 11 mph, or 4.2 to 5 m/sec), heat production increases 10 to 20 times that produced at rest, and during sprinting it can increase 40 to 60 times. Without heat loss, this would increase the horse’s body temperature to a life-threatening level above 106°F (4l°C) within 4 to 6 minutes. Even at 50% of exertion capacity, the horse’s body temperature without cooling would approach the critical level after 10 minutes. Fortunately, with normal heat dissipation, these increases in body temperature do not occur. The heat produced in the body, which during exercise is produced primarily by the muscles, is transported by the blood to the skin and, to a lesser extent, the respiratory tract, where it can be dissipated. The greater the increase in body temperature and, therefore, cooling need, the more blood is shunted to the skin. This may contribute to fatigue, as it decreases oxygen delivery to the muscle, which would increase glycogen utilization and lactic acid production and decrease lactic acid removal.
The evaporative cooling of sweat accounts for about 55 to 60%, and evaporative cooling from the respiratory tract about 25%, of heat dissipation by the horse. Evaporative cooling occurs even at ambient temperatures above the horse’s body temperature, but is greatly impaired as the humidity increases. In contrast, the remaining 15 to 20% of heat dissipation by the horse is primarily by convection, but only if the ambient temperature is less than the horse’s body temperature. Both evaporative cooling of sweat and heat loss by convection are enhanced by air movement over the body, which occurs when the horse is moving or there is a breeze, and both are impaired with increasing ambient temperature and humidity. When the sum of the ambient temperature in degrees Fahrenheit and the percent relative humidity combined are under 130, heat loss for horses or people should not be a problem. When the sum exceeds 150, especially if humidity contributes over 50% of the sum, heat loss is severely compromised; and when the sum is over 180, little heat dissipation can occur. Neither equine nor human competition or exertion should occur when the value is over 170, and at values between 150 to 170 they should be done with caution. Heat loss is further impaired in the poorly conditioned horse, the fleshy horse, and the horse that still has its winter coat. Not only is heat loss decreased, but heat production is greater for a similar amount of exercise the poorer the horse’s physical condition. In one study, rectal temperature increased 50% more in unfit than fit horses following the same amount of exercise.
The evaporation of 1 liter of sweat dissipates 580 kcal of heat, which is the amount of heat produced by 7 to 8 minutes of trotting or loping at 5:30 to 6:40 min:sec/mile (9 to 11 mph, or 4.2 to 5 m/sec). Horses’ sweat contains protein, whose concentration decreases after the early stages of sweating. This protein has detergent-like properties that help disperse sweat droplets into a thin film along the hairs; this helps evaporation. This is the reason-horse’s sweat lathers. With prolonged or frequent sweating, this protein becomes depleted, resulting in a more watery sweat, more likely to run off the horse instead of evaporating and thus cooling the animal. Evaporated cooling from sweat, and thus the efficiency and benefit derived from sweating, also decrease with increasing humidity. As a result, a horse that is only slightly wet with sweat may be losing more heat by rapid evaporation of its sweat than one that has sweat running off it. Wiping sweat off is counterproductive, as it prevents its evaporative cooling effect; putting water on the horse and/or enhancing evaporation enhances cooling.
The horse’s maximum sweating rate is 10 to 15 L/hr and is 6.5 to 9 L/hr at endurance racing speeds (trotting or loping at 4:20 to 6:40 min/mile, or 4.2 to 5.8 m/sec). Thus, as shown in Table 11-3, an extensive amount of body water is lost during athletic performance by the horse resulting in up to a 50 (qt) sweat loss, an amount equal to the horse’s total blood volume. If not replaced, this would result in 7 to 11% dehydration or decrease in body weight. From 12 to 15% dehydration is fatal. The effects of moderate dehydration in horses are probably similar to those in people, in which it has been shown that physical performance is impaired with fluid losses that decrease body weight by only 2 to 4%. A dehydration-induced decrease in blood volume decreases blood flow to both the muscles and the skin. Decreased blood flow to the muscles decreases muscle performance and leads to fatigue or exhaustion. Decreased blood flow to the skin decreases heat dissipation both by decreasing heat transport to the skin and by decreasing sweating, which increases the risk and onset of heat stress or exhaustion.
TABLE 11-3 Weight Loss by Horses Due to Athletic Competition a
Weight Loss b | ||
Event | Lbs. | Kg |
Thoroughbred galloping or breezing | 10–16 | 4.5–73 |
Standardbred 1 mile (1.6 km) race | 12–33 | 5.5–15 |
Field Hunters 3 hrs fox hunting | 24–100 | 11–45 |
After phase D of endurance day in a 3-day | 40 ± 18 | 18.4 ± 8.3 |
event and 18 to 24 hrs later | 12 ± 25 | 5.5 ± 11.4 |
50 mile (80 km) endurance race | 65–110 | 30–50 |
a Greater the higher the environmental temperature and humidity, the poorer the condition of the horse, and the more the exertion required.
b 90% of which is water.
Sweating results in the loss of not only water but also sodium, chloride, potassium, and lesser amounts of calcium and magnesium, and if excessive, may result in a significant body deficit of these electrolytes. At similar sweating rates, the horse will lose 3 times more sodium and chloride and 5 to 10 times more potassium than do people. However, plasma sodium and potassium concentrations generally remain within the normal range. But, particularly with exhaustion, a significant decrease in plasma potassium may occur. Decreased sodium concentrations may also occur due to sodium losses, followed by partial replacement of the water deficit by drinking. However a decrease in chloride is the most consistent plasma concentration alteration in profusely sweating horses. Calcium and magnesium concentrations also tend to fall with excessive sweating, whereas phosphate concentration tends to increase as a result of phosphate release from muscles upon physical exertion.
A sweat-loss-induced decrease in plasma chloride concentration results in alkalosis. Alkalosis inhibits respiration, resulting in a decrease in carbon dioxide excretion and in oxygen intake and thus oxygen’s availability for energy production. This contributes to glycogen depletion, lactic acid accumulation, inadequate energy production, and consequently fatigue or exhaustion. Oxygen availability to the tissues is decreased further by inadequate tissue perfusion due to electrolyte depletion and dehydration-induced decrease in blood volume, and an increase in blood viscosity.
A decrease in plasma potassium concentration decreases muscle strength and tone. It and a sodium deficit also decrease appetite and thirst, which decrease water and food intake necessary to correct the deficits and the energy depletion.
These acid-base and electrolyte alterations as a result of endurance-type activity-induced sweat losses are opposite to what occurs with anaerobic sprint-type exertion at speeds near or above 2:40 min/mile (10 m/sec). The anaerobic exertion-induced increase in lactic acid production tends to cause acidosis and an increase in the plasma potassium concentration. Potassium, along with phosphorus and cellular enzymes such as creatinine kinase and alkaline phosphatase, are also lost from the muscle in response to strenuous exertion. Following exercise, the plasma potassium concentration returns to normal within 4 to 5 min, whereas it takes from 40 to 70 minutes for the plasma lactate concentration to return to normal.
A decrease in the plasma calcium concentration following extensive sweating by the horse is generally accompanied by a decrease in plasma magnesium concentration. This decreases, and if sufficiently severe, causes anxiety, increased neuromuscular excitability resulting in muscle twitching, increased muscular tension (particularly of the extremities), incoordination, synchronous diaphragmatic flutter (thumps), and hypocalcemic tetany or tying up, as described later in the section on these diseases.
The presence of dehydration can be detected clinically by: dryness of the mucous membranes and eyes, and decreased jugular vein distensibility, rate of capillary refill, and skin elasticity. However, physical performance is reduced prior to any indication of dehydration. Dehydration of 4 to 5% (i.e., a 4 to 5% water-loss-induced decrease in body weight) or greater can be detected by delayed recoil of a fold of pinched-up skin, which is best observed in the skin over the shoulders. The skin over the neck is looser so when pinched, it stands up more readily, making it a less accurate indication of hydration. In the normally hydrated horse, a pinch of shoulder skin should return to its original position within 1 second, and capillary refill time should be less than 3 seconds. Both become increasingly delayed with increasing dehydration. With further dehydration, additional effects become noticeable, including sunken eyes, dry mouth, dry feces, and decreased urine volume. The plasma protein concentration and hematocrit are increased. The hematocrit of the dehydrated, exhausted horse used for endurance activity is generally, but not invariably, greater than 45 to 50% and may be up to 60%. The hematocrit seldom exceeds 45% unless the horse is dehydrated. Treatment of these effects, which result in fatigue and exhaustion, are described in the section on “Diseases Due to Athletic Performance” later in this chapter. A number of things may be done to minimize exercise-induced water and electrolyte deficits and, as a result, help prevent decreased performance, fatigue, and exhaustion.
Water and Electrolyte Deficit Prevention
In contrast to many nutrients, there are no body stores of water or electrolytes other than those carried in the gastrointestinal tract. Any excess absorbed is rapidly excreted in the urine. Thus, body water and electrolyte deficits cannot be prevented by giving them before they are lost. But severe deficits can be prevented by replacing them as they are lost. To do this, salt (sodium chloride), like water, should be always available for the horse to consume as much, as often, and whenever it wants.
All feeds are low in sodium and below that needed to meet the frequently sweating horse’s needs (Appendix Table 2). Many feeds contain even less sodium than that needed to meet the nonsweating horse’s needs. For the frequently or profusely sweating horse, loose salt may be preferred, as some horses may not lick enough from a block.
Horses need 125 g of sodium and 175 g of chloride (318 g, or 11 to 12 oz of salt) per day of excessive sweating. The horse will consume the additional amount of salt needed if it is available. If it isn’t, this amount, or appropriately lesser amounts for shorter-duration or less-profuse sweating, should be added to the grain mix at each feeding for 1 to 2 days afterward. This amount of salt should not, however, be continually present in the grain mix fed. Over 15 lbs (7 kg) of a grain mix containing 4%salt would be needed to provide the amount of salt lost from a full day of excessive sweating. However, since workouts and, therefore, excessive sweating do not occur daily, and because consumption of excessive amounts of sodium is detrimental, it is recommended that if salt is added to the grain mix it not exceed an amount equal to 0.5% of the total diet.
Adding excess salt to the horse’s diet increases water and potassium excretion. This, particularly in conjunction with excessive sweating-induced potassium losses, may cause a harmful potassium deficit resulting in a low plasma potassium concentration, decreased performance, excessive urination, and mild dehydration. The potassium deficit is worsened by a high-grain-low-forage diet which is low in potassium, as potassium is low in grains is (0.3 to 0.5%) and high in forages (1 to 3%). At least 0.2% potassium in diet dry matter is adequate for the horse at rest, whereas 0.6% is needed during periods when sweating is frequent or profuse. The horse needs from 5 up to 13 lbs (2.3 to 6 kg) of forage daily to provide this amount of potassium.
Before beginning endurance-type activity, the gastrointestinal tract should contain as much water and electrolytes as possible. The gastrointestinal tract contains an amount of water and electrolytes equal to 6 to 10% of the horse’s body weight (8 to 12 gal, or 30 to 50 L, in the 1100-lb, or 500-kg, horse). The amount is increased by the consumption of forage, and is decreased by the consumption of grain and a complete pelleted feed. Thus, both water and forage consumption before, and as frequently as possible during, endurance activity is beneficial in preventing dehydration and electrolyte deficits. To encourage drinking during prolonged competition, the horse should be offered water frequently during training. It has been noted that horses that drink little during endurance races, particularly races that are long, difficult or during hot humid weather, are less likely to finish the race, or more likely to finish slowly, than horses that drink sufficiently during the race to prevent excessive dehydration. Thus, the horse’s learning to drink during prolonged activity is a helpful aspect of training.
Particularly during and following exercise, cold water is preferred over warm water, as it helps cool the horse and is emptied from the stomach, and thus absorbed, faster. Drinking even large amounts of cold water during exercise does not lead to colic or any other detrimental effects, and in contrast may be quite beneficial.
During endurance activity, if the temperature and humidity are high (if °F + % humidity exceeds 150) and it is more than 1 to 2 hours between watering places, carrying a couple of gallons (8 L) of water and giving it to the horse in a hat or collapsable pail between otherwise available watering places may be warranted. If doing this in competition is anticipated, it should be done during training to encourage the horse to drink. Feeding a small amount of grain as a source of glucose, and replacing electrolyte losses, during endurance activity may also be beneficial for some horses. Giving electrolytes is of little benefit unless sweating and, therefore, electrolyte losses, are fairly high. As has been shown in people, and is quite likely valid in horses, drinking a glucose-electrolyte-containing solution delays fatigue and improves performance more than water during endurance activities. Providing horses water, sodium, potassium, chloride, and glucose or grain during extended work in hot weather has been shown to increase water consumption, thus decreasing dehydration and does seem to enhance their aerobic performance capacity and delay fatigue.
The major electrolytes in the 6.5 to 9 qts./hr of sweat the horse loses at endurance speeds may be replaced by giving the horse 3 oz (6 level tablespoons or 85 g)/hr of a mixture of equal parts common salt (sodium chloride) and “lite” salt. This should be given just before, every 1 to 2 hours during, and after endurance activity by mixing it into about 1 qt (1 L) of grain mix containing molasses. The molasses in the grain keeps the salt mixture from sifting out. The grain mix not only serves as a vehicle to get the horse to eat the electrolytes needed, it also provides glucose. Following its consumption, water should be offered. Consumption of the grain and the electrolytes stimulates drinking, as does their benefit in preventing a decrease in the plasma sodium concentration which inhibits thirst.
“Lite” salt, or low-sodium salt, is one-half sodium chloride and one-half potassium chloride. It can be purchased at most grocery stores. Instead of “lite” salt, an identical mixture can be prepared by mixing 3 parts sodium chloride and 1 part potassium chloride, although potassium chloride is generally less readily available and more expensive than “lite” salt. Either salt mixture, however, is an economical way to provide the major electrolytes lost in sweat.
The salts should not be added to the horse’s drinking water, as they will decrease most horses’ water consumption and, therefore, are counterproductive. In addition, if added to the horse’s water, they prevent the horse from compensating if intake of any of the electrolytes is more than that needed. Providing that water is available for the horse to drink to satiety, there is little or no risk of giving too much of the salt or electrolyte mixture recommended. Whether giving them is of benefit depends on the amount of sweating-induced losses. Thus, giving electrolytes as recommended, whether needed or not, will not be harmful; if not needed, they won’t be of any benefit, but if needed, they may be quite beneficial and, therefore, are recommended in situations where there is prolonged, profuse, or frequent sweating.
Feeding a high-fat diet and good physical conditioning, (both of which decrease body heat production), cooling the horse, and minimizing or avoiding the factors that cause or predispose to hyperthermia, as discussed later in this chapter in the section on “Heat Stroke,” are also quite helpful in minimizing sweating and, therefore, water and electrolyte deficits.
TRAINING TO INCREASE FITNESS FOR ATHLETIC PERFORMANCE
Much of a horse’s ability is genetic. However, the genetic ability the horse is born with may be altered greatly by life’s experiences, one of which may be training. Training has many effects that alter a horse’s behavior and physical ability. These include: (1) mentally and physically learning what, when, and how to do, and (2) improved physical fitness to do what it is trained to do. Optimal training enhances both the horse’s desire and its ability to improve its performance. In contrast, inadequate, excessive, or improper training will decrease either or both the horse’s desire and its ability to improve and maximize its performance.
Factors that affect performance include:
Training can influence all of these factors to varying degrees. The importance of training on improving these factors depends on the horse and the type of athletic activity for which the horse is being trained, although for nearly all types of activity, training to enhance durability is beneficial. For example, show jumping requires training of biomechanical skills as well as enhancing both aerobic and anaerobic capacity. In contrast, for endurance racing an improvement in cardiovascular and respiratory function for increased aerobic fitness or stamina is the major aim, whereas for sprint or strength events such as Quarter Horse racing or draft horse pulling contests, training should emphasize improving anaerobic fitness, speed, and muscle strength. Training that increases physical fitness that enhances performance ability does so in many ways. These include training effects that: (1) increase muscle size or strength, contractile speed, and endurance; (2) increase skeletal, tendon, and cartilage strength and flexibility; (3) improve thermoregulation with decreased water and electrolyte losses; (4) increase oxygen intake, delivery, and utilization capability; and (5) increase the rate of energy production and utilization.
Exercise increases aerobic capacity by increasing: (1) the percentage of oxidative muscle fibers and the oxidative capacity of individual Type IIB fibers, (2) muscle capillarity; (3) blood volume; (4) red blood cells release of oxygen to the tissues, and (5) muscle mitochondria. Increased muscle capillarity enhances carbon dioxide and lactic acid removal and oxygen delivery by allowing for a greater blood flow and shorter distance for diffusion into and out of the muscle fibers. Increased capillarity, or size of the vascular compartment, requires an increase in blood volume which also occurs as a result of training. Training increases the horse’s plasma volume 25 to 35%, with 90% of the increase occurring in the first week of training. The ability of the muscles to use the additional oxygen is increased by an increased proportion of high oxidative muscle fibers (I and IIA) and oxidative enzymes in Type IIB muscle fibers, and by an increase in muscle mitochondria, which is where oxygen and fat utilization for energy production occur.
Training at speeds that maintain a blood lactate concentration of 4 mM/L is considered to be optimal in enhancing both aerobic and anaerobic metabolism needed for middle-distance (Table 11-1) racing performance. In most horses, this speed produces heart rates of about 190 to 220 bpm and corresponds to about 80 to 85% of the horse’s maximal speed. Running at this speed for 2 to 5 furlongs (400 to 1000 m) or incorporating short sprints into a fartlek routine, increases heart and lung capacity to deliver oxygen to the muscle, which is probably the limiting factor for aerobic capacity in highly conditioned race horses. For other horses, the most limiting factor for aerobic capacity is probably the rate at which the muscles can utilize oxygen, which can be doubled with training by long, slow-distance exercise (i.e., galloping continuously for long distances at a speed that maintains the heart rate at 140 to 150 bpm).
Training to improve physical condition increases exercise capacity not only by increasing the rate at which oxygen can be delivered and utilized and, therefore, that energy or ATP can be produced without using glycogen or producing lactic acid, but also by increasing the amount of glycogen that can be stored in muscle and by increasing the use of fat as an energy source, thus sparing glycogen. These effects delay glycogen depletion and lactic acid accumulation, and as a result delay fatigue and exhaustion. Two procedures (besides training for increased physical fitness) used to increase muscle glycogen content, or delay its depletion and lactic acid accumulation during physical exertion, are feeding diets high in either soluble carbohydrates (starch from cereal grains) or fat, and glycogen loading. Both were discussed previously in the sections in this chapter on “Dietary Fats for Athletic Performance” and “Feeding and Supplements Before Athletic Performance.”
A training program should consist of three parts: an initial stage, a developmental stage, and a maintenance stage. In horses, the cardiovascular and muscular systems respond rapidly to incremental increases in their workload or training with significant changes being produced in only a few weeks. In contrast, supporting structures such as bone, cartilage, ligaments, tendons, and hooves adapt more slowly over a period of several months. Consequently, early improvements in the horse’s cardiovascular and muscular systems allow exertion sufficient to cause injury to these supporting structures. To prevent this, the early stages of training must be kept at levels of exertion below what the horse is able to do. To do this the initial stage of training for any type of competitive event should be long, slow/distance (LSD) workouts for at least 1 month for mature horses sufficiently fit for competition without injury the previous season to as long as 3 to 12 months for the young horse in first year of training. The objective of the LSD training phase is to prepare the horse to cope with 45 to 60 min of easy exercise at a walk, trot and canter, at an average speed of 4 to 5 mph (6 to 8 km/ hr), including 2- to 3-min periods of cantering at moderate speeds of 10 to 12 mph (16 to 18 km/hr). This type of exercise is performed 3 times a week, alternating with schooling days. By the end of the ISD phase of training the horse should be able to gallop up to 6 mi (10 km) in 20 to 24 min and maintain a heart rate of near 140 bpm. The initial stage of LSD training increases the horse’s suppleness, mobility, and adaptation to saddle and rider, or to pulling a sulky. It strengthens structural components, decreasing the occurrence and risk of training and racing injuries, including stocking-up, bucked shins, splints, bowed tendons, carpal bone chips, fractures, etc. Bone, tendon, and ligament strength increase with exercise that loads these structural components. Both the number of repetitions and the intensity of load factors increase their strength. Long, slow-distance, or aerobic exercise also increase the horse’s cardiovascular ability, but do little to improve anaerobic performance ability. As a result, long, slow-distance exercise will not help make a slow horse run fast. But increasing aerobic or oxygen utilization ability by long, slow-distance exercise will help all horses maintain a faster speed longer, which for distances of more than about ⅝ mile (1000 m) may increase the speed that distance can be run. It’s major benefit and necessity, however, is to prevent the exertion induced injuries described later in this chapter that occur so commonly in training and competition.
The second or development stage of training is for the development of the cardiovascular system and muscles specific for the future competitive event desired. There should be a good balance between high-intensity and prolonged duration, with this balance depending on the type of competitive performance event for which the horse is being trained. The more intense the exertion, the more important high-intensity, speed, or anaerobic training is; the less intense the exertion, the more important duration or aerobic training becomes. It is the intensity of competitive exertion that is important, not the duration of exertion or event. For example, although the speed of show jumping is slow and the duration long, this event requires very intense exertion, and therefore the use of anaerobic metabolism. Draft horse pulling competition is another example of slow activity which requires maximum, intense exertion, whereas Quarter Horse racing is an example of maximum intense exertion for speed. Both require strength and anaerobic energy production. Thus, the development stage of training for the show jumper and the draft pulling horse—like the Quarter Horse sprinter, cutting, rodeo event, and barrel racing horse—should emphasize training to increase anaerobic capacity (i.e., speed and strength). For endurance sports, training should concentrate on just that: i.e., endurance to increase aerobic capacity. For middle-distance events, such as Standardbred trotter racing and Thoroughbred racing and jumping, both anaerobic and aerobic work must be done. Thus, the development stage of training should be exercise in performing the same task as planned for future competition. This is necessary not only for conditioning all parts of the body used for that type of competition, but also for neural learning of the task for neuromuscular coordination to perform that task most efficiently. Neural learning may account for a large proportion of the improvement from training.
For high-intensity exertion or sprint strength training, a thorough warm-up is followed by running the horse several times at near maximum speed for about 200 m (1 furlong), with complete recovery to a heart rate of near 70 bpm in between each sprint. Complete recovery will take about 10 min, depending on the horse’s fitness. Running uphill also increases strength and thus sprint speed. Although these high-intensity sprint workouts are beneficial in increasing anaerobic capacity, they should be limited to once every 3 to 5 days depending on the number of sprints and their duration, to prevent musculoskeletal injuries. Barring severe or clinically apparent tissue damage, short anaerobic sprints require about 48 hours for recovery, while long-duration anaerobic and aerobic exercise can require 3 to 7 days for recovery. In contrast, with lower-intensity exercise (at heart rates below 150 bpm), the horse can work out 5 times a week. Usually it is best to vary the intensity of work between sessions.
Anaerobic capacity, the essence of raw speed, is a genetic characteristic that may perhaps not be greatly improved with training. But even in a quarter-mile (400 m) race, some aerobic metabolism is used, with its use increasing as the distance increases. Sprint training increases lactic acid buffering and increases oxygen intake and utilization, thus delaying fatigue and allowing the horse to maintain a faster speed throughout the race. Such horses appear to finish fast at the end of the race, whereas in actuality what is happening is that other less-fit horses are slowing down while the fit horse is maintaining its speed.
For all types of equine competitive activities, for maintenance training during the competition season, shorter duration (one-half), but near competition-intensity workouts, may be best for the fit horse to maintain fitness and optimum performance ability.
For middle-distance or traditional racehorse developmental training, the procedures currently used by most trainers include trotting and a slow lope or canter over a distance longer than the actual race distance. This is done every day. In addition, 2 to 4 times per week the horse is given a single sprint or breeze at about ¾ speed for 35 to 65 seconds going 3 to 5 furlongs (600 to 1000 m) followed by a cool-down. Interval training may be used by some racehorse trainers to try to increase performance.
Interval training refers to a series of two to six sprints at a fast lope or gallop from one-half to full speed for 1 to 8 furlongs (200 to 1600 m), with walking or trotting in between sprints until the heart rate decreases to 100 to 150 bpm. As this wide variation in interval training methods indicates, the best method varies with stage of training, its specific purpose, and the horse’s response to it. Each individual horse being interval trained should be evaluated daily and its training regimen continually revised, as indicated by the horse’s performance and response.
When beginning interval training, sprint duration will be longer and the number of repetitions fewer than as fitness develops. Interval training should be done only every 4 days to allow full recovery, with lower-intensity exercise on the days in between. A compromise between interval and conventional training is often used in Thoroughbred race horses. After warm-up, horses are run at moderate to high speed for a short duration with rest periods in between each run. For example: 4 runs of 3 furlongs at 45 seconds each with a 3:30-min recover period between each run.
For interval training to increase stamina, initially it may be best to run fast enough to increase heart rate to 180 to 200 bpm, then with increasing fitness increase speed enough to increase the heart rate above 200 bpm. The duration of recovery should be sufficient to allow the heart rate to decrease to around 110 bpm. If the heart rate exceeds what is expected for that particular stage of the training, or if it takes longer for the heart rate to decrease, this indicates that the workout is too stressful and no further work intervals should be done. An unexpectedly high heart rate during warm-up or recovery may also be an early warning that something is wrong, in which case the horse should be examined thoroughly for a problem.
A variation of interval training is fartlek training, which is unstructured alternating periods of slow work and fast work generally below maximum speed but maintained until the horse begins to tire. It helps alleviate boredom for both horse and rider and is useful for both endurance and eventing horses, particularly for those that have an intermittent pattern of energy expenditure, such as polo, combined driving and eventing.
The basic idea of interval training is that it allows more intense work for a longer total duration than if that work were continuous, and that it requires a more frequent recruitment of a higher percentage of muscle fibers and lactate production. This allows adaptation, which increases aerobic metabolism capacity, thus decreasing reliance on anaerobic metabolism.
An increase in aerobic capacity or stamina, however, is not beneficial for sprint-type activities. Increased aerobic capacity is associated with decreased muscle size to allow increased oxygen movement into the muscle fiber, but a decrease in muscle size decreases its strength and, therefore, its rapid acceleration ability needed for sprint-type events. Sprinters, therefore, need training programs that build maximum strength, not maximum stamina. You cannot train for both, nor will an individual horse be good at both. This is why most horses have a certain distance at which they perform best, and why true sprinters cannot be stayers and vice versa. Interval training, therefore, appears to decrease maximum early speed. But it does appear to allow the horse to maintain a fast speed longer and to race well more frequently, both of which are of benefit for middle-distance-type events. Interval training has been shown to substantially delay fatigue as compared to what is obtained by long, slow distance work-outs. In one study, although the differences weren’t statistically significant, the average speed of four noninterval-trained Thoroughbred racehorses was faster at the first furlong (220 yds, ⅛ mile, or 200 m), but the average of the four interval-trained horses was faster for each of the next 4 furlongs, and for the 5 furlongs was 0.66 sec faster, which is equivalent to the interval-trained horse’s winning by about 11 yards (10 m) in a ⅝-mile (1600-m) race. Regardless of the benefit of interval training and of long, slow-distance training, some contend that because both take much more time, management and, therefore, cost than conventional training, they may not be practical for some horses (e.g., horses raced in claiming races).
For endurance training, emphasis should be placed on the development of aerobic metabolism capacity. To do this, the following training regimen has been recommended. Begin with walks and easy trots of 6 to 8 miles (10 to 13 km) or 30 to 60 minutes 3 times a week for 3 or 4 weeks. Increase to 10-mile work-outs during the second month, but at the same pace of 6 to 8 mph (10 to 13 kph) 3 to 5 times per week. Work-outs should last an hour or more with a heart rate of 80 to 100 bpm. Rest is indicated for a few days to a week if there are any signs of stiffness, soreness, or swelling, or if the heart rate is higher than it has been previously. The heart rate may be used as an indication of appropriate training level. At 10 minutes after beginning exercise, a heart rate of 60 ± 4 bpm indicates a good training level, 48 ± 4 indicates that you can increase training intensity, and 72 or greater indicates that you should decrease training intensity. Others feel that it is best to train at a slow gallop sufficient to maintain the heart rate at 140 to 150 bpm beginning with 10 minutes daily and increasing this gradually to 20 minutes 5 times per week. Following the third month of training, if no stiffness or soreness has occurred, then once or twice a week include two, gradually increasing to four, short sprints that increase the heart rate to 180 to 210 bpm for 40 to 60 sec. In between each sprint, walk to allow the heart rate to recover to 80 to 110 bpm. Ideally this should be continued for 3 to 6 months before competition begins. In the final 2 weeks before the endurance event, the overall intensity of the workout is decreased, although one moderate ride should be taken.
Age to Begin Fitness Training
It appears that beginning exercise conditioning to increase physical fitness when the horse is 18 months of age, but not much younger, is beneficial. Four-year-old Standardbreds exercised since 18 months of age had higher percentages of oxidative muscle fibers and oxidative enzymes in their muscles than those that were not given forced exercise until 3 years of age. These same results have also been obtained in Thoroughbreds and Quarter Horses. However, no metabolic benefit is obtained from forced exercise training prior to 18 months of age. Horses not given forced exercise until 18 months of age appear to catch up within a few months to the metabolic advantages obtained by forced exercise prior to this age.
Loss of Fitness with Rest
In horses—in contrast to people, who lose muscular adaptation to fitness training within a few weeks—the effects of training to increase fitness are maintained even after several weeks to months of rest, as may be necessary in the management of illness or injury. No decrease in fitness was observed in physically fit horses following 5 weeks of rest, and there is little loss of cardiovascular fitness during 6 months of paddock rest. Training effects on muscle oxygen utilization ability are maintained for 5 to 8 weeks. However, in non-sprint-type events requiring high levels of aerobic energy production, significant reductions in peak oxygen intake capacity can occur with as little as 2 weeks of inactivity. Based on results of this type, it’s been suggested that horses taken out of training for less than 4 weeks can return to initial levels of cardiopulmonary fitness with 2 to 3 weeks of retraining, but that longer periods of inactivity may require 6 to 8 weeks of retraining to attain maximum cardiopulmonary fitness. This or a longer period of retraining to bring the horse back to the same level of training it was at prior to an interruption in training of even as short as one to several weeks may be necessary to prevent stress-induced tendon, ligament or bone injuries, such as bucked shins. A rule of thumb is to allow a week of reconditioning for every week off, but this should be modified as indicated in the individual by such things as too rapid a heart rate for the situation or any soreness or stiffness.
FITNESS EVALUATION FOR ATHLETIC PERFORMANCE
There are two major reasons that the physical fitness of the equine athlete should be closely monitored: (1) so the progress of training can be determined, which is necessary so training procedures can be altered as indicated; and (2) so overtraining, lameness, soreness, respiratory problems, or any other illness, injury or dysfunction can be readily detected and appropriate measures taken to prevent and minimize their severity and effects. Training and performance are stressful and may result in musculoskeletal injuries and suppression of the immune system. Suppression of immune function predisposes to infectious diseases, particularly respiratory diseases.
Overtraining or overuse so that the horse doesn’t have sufficient time to recover from exertion may not only result in physical damage decreasing the horse’s ability, but may also suppress the horses desire and, therefore, effort. Overtraining or overuse may be indicated by two different blood tests. One is a persistently low ratio of the white blood cell types neutrophils/lymphocytes. An intermittent or transient decrease in this ratio is normal following physical exertion, but if it is persistently low, it suggests overuse-induced stress. The second blood test suggesting overuse is an increase in the plasma activity of the liver enzyme gamma-glutamyltransferase (GGT) to more than twice normal. About 30 days of small-paddock or pasture rest is often sufficient to allow the horse to recover from overtraining or overuse without losing a significant amount of previously achieved fitness.
Physical problems requiring rest are also common in the horse in training or competition. The most common causes of inadequate performance are: (1st) musculoskeletal pain, most commonly from multiple, not single, sources; (2nd) upper respiratory dysfunction, such as from laryngeal or pharyngeal muscle problems; and (3rd) lower respiratory dysfunction, such as bronchitis and exercise-induced pulmonary hemorrhage. From 60 to 90% of all Thoroughbreds in training and competition are reported to develop major lameness problems. Most, if detected prior to being visibly evident, such as by heart rate monitoring during exercise and recovery, will respond to an appropriate rest period with no permanent detrimental effects and minimal loss of training and competing time and fitness. Early detection and treatment of respiratory dysfunction also minimizes its detrimental effects and their duration.
Observing a horse before, during, and after workouts, including its respiratory patterns, gait, day-to-day progress, and overall performance as compared to other horses are routinely used subjective indicators of fitness or problems. The time it takes to cover a specific distance is also commonly used a gradual decrease being indicative of training progress, and an increase being indicative of a problem. However, there is a poor correlation between speed for a short distance (5 furlongs, or 1200 m, or less) and that achieved for a longer distance. A more precise indication of training progress and increased fitness is a decrease in either the plasma lactate concentration or the submaximal heart rate that occurs at any specific workload sufficient to increase them above resting levels. Thus, fitness and training progress can be assessed by determining either: (1) the amount of increase in either the plasma lactate concentration or the submaximal heart rate that occurs with a specific amount of exercise, or (2) the amount of exercise (speed, or duration at a certain speed) necessary to reach either a certain plasma lactate level or a certain submaximal heart rate. Typically, the amount of exercise that results in a plasma lactate concentration of 4 mmol/ L or a heart rate of 200 bpm is used as an indication of physical fitness. The amount of exercise necessary for either to occur also increases with increasing age from 2 to 5 years, at which age they plateau until 8 years of age before beginning to decrease.
The heart rate 5 minutes after exercise has stopped is also a meaningful indication of the horse’s degree of physical fitness. In contrast, neither resting nor maximum heart rates, rate of decline in plasma lactate concentration following exercise, body temperature, plasma activities of muscle enzymes, respiratory rate, or any blood parameters prior to exercise, including hematocrit, blood hemoglobin concentration, or red blood cell number, volume, or hemoglobin concentration or sedimentation rate, or any number or proportion of white blood cells is a meaningful indicator of the horse’s physical fitness.
The hematocrit at rest in hot-blooded horses is generally 32 to 46%, and in cold-blooded horses 26 to 32%. It tends to be lower in sprint than endurance horses because endurance horses perform more aerobic work, which stimulates increased red blood cell production. The hematocrit in some fitsprinters may be as low as 32%. It may be decreased by many tranquilizers and is increased by anything causing splenic contraction, which includes excitement, stress, or physical activity.
Body Weight as an Indicator of Fitness
A frequently overlooked but meaningful indication of physical fitness affecting racing performance is die horse’s weight. Horses, like all athletes, have an optimal performance weight. Thoroughbred racehorses’ optimal racing weight has been found to be within a 16-lb (7.3-kg) range. It was found that performance is more likely to be adversely affected when the horse is below its optimal weight than when it is above it. For most horses, optimal physical performance weight is at moderate body condition as described in Table 1-4. Below this condition, body energy-source nutrients, including muscle glycogen, are reduced before, as well as after, exercise. This decreases both endurance and sprint-type performance ability. However, above-moderate body condition is also detrimental because: (1) muscle glycogen content is not increased, (2) there is excess weight to carry, and (3) the dissipation of exercise-induced heat production is impaired, which increases sweating-induced water and electrolyte losses and body temperature. Most horses’ ideal racing weight is the same when they are 3 year olds as when they were 2 year olds. The average weight loss by Thoroughbreds during a race is about 9 lbs (4.1 kg). Those that perform well the next week gain this weight back within 48 hrs.
Effects on, and methods for obtaining, the horse’s weight are given in Chapter 6.
Heart Rate as an Indicator of Fitness
Of all the means of assessing physical fitness, measuring heart rate response to exercise and recovery is the easiest to do and the most meaningful. Numerous on-board heart rate monitors are available to measure heart rates during both exercise and recovery. These include Equistat Heart Rate Computer (Equine Biomechanics and Exercise Physiology, Inc., Unionville, PA), Hippocard (Speedtest Thoroughbreds, Inc., Ontario, Canada), and VMAX (Equine Racing Systems, Vevay, IN). The heart rate during recovery can, of course, easily be obtained by listening to the heart or counting the pulse. The pulse may be taken at the maxillary or facial artery on the inner aspect of the lower mandible just in front of the jaw, immediately before the artery crosses under and to the outside surface of the mandible. In most horses, the normal resting heart rate is 30 to 45 bpm; about 80 when walking; 120 to 140 when trotting; 160 to 200 when cantering, loping, or swimming; and over 200 when galloping. At about 210 bpm, the heart rate begins to plateau to its maximum of 220 to 250 bpm, which varies among individuals.
As people become more physically fit, their resting heart rate decreases. This occurs because there is an exercise-induced increase in heart strength which increases stroke volume (SV), allowing cardiac output (CO) to be maintained at a lower heart rate (HR) (since CO = HR × SV). However, unlike people, stroke volume in horses changes little with exercise, so change in heart rate is the only significant means of changing cardiac output. As a result, although some horses’ resting heart rate decreases somewhat with increased fitness, this doesn’t occur to any significant degree in most horses. Nor does increased fitness affect most horses’ exercising or their maximum heart rate by any significant amount. However, there is a direct correlation between increasing physical fitness of the horse and both (1) a faster speed, or longer duration at a certain speed, that can be obtained before a pre-plateau heart rate (up to 210 bpm) is reached; and (2) a faster decline of the heart rate following exercise. Both of these factors are reliable indicators of the horse’s degree of physical fitness. The heart rate at 5minutes after exercise stops appears to be the most useful criterion.
When no change occurs in either the pre-plateau heart rate reached during, or the heart rate at a specific time after, successive bouts of running at a specific speed for a specific time (or any other identical bouts of exercise intensity and duration), either: (1) the intensity of the training program should be increased if further cardiopulmonary fitness is wanted, or (2) the horse has reached its maximum level of cardiopulmonary fitness, and increasing the intensity of training is unlikely to be beneficial. To distinguish between these two possibilities, the intensity or duration of the training program should be increased. If the heart rate at a given speed does not continue to decrease, or if the speed at a given heart rate (or percent of maximum heart rate) does not continue to increase, it indicates the horse has reached its maximum level of cardiopulmonary fitness. Changes in plasma lactate concentration before and after exercise can also be used to measure physical fitness, but doing so is technically more difficult than measuring the heart rate.
The heart rate is increased not only by exercise but also by fatigue, apprehension, anxiety, discomfort, or pain from any cause, and by any respiratory dysfunction or difficulty. Because of this, any lameness, soreness, or respiratory difficulty generally, before it is sufficiently severe to be seen, can be detected by an increase from the previous day’s or exercise’s effect on the heart rate. In addition, the amount of increase in the heart rate is proportional to the severity of the pain or respiratory difficulty. However, because of the wide variation in each individual horse’s heart rate, these increases can be detected only by comparing each horse’s heart rate when not in pain or with a respiratory problem to its heart rate when affected. In addition, the effect these problems have on enhancing the exercise-induced increase on heart rate decreases rapidly following exercise, so that, although it may be evident at 1 minute, it may not be evident at 5 minutes following exercise.
Lameness-induced increases in heart rate during exercise are greater at trotting than at faster speeds, since pain has a greater psychological effect at lower-intensity exertion. However, following exercise, the greatest percentage increase in heart rate as a result of lameness occurs the faster the exercise speed was.
Monitoring the heart rate within the first minute and again at several intervals following a fast workout, and comparing it to that obtained previously on the same horse, provides the best opportunity of detecting any lameness or soreness the earliest. Less diagnostic but, perhaps helpful, is the recommendation that fit Thoroughbred horses’ heart rates after exercise should return to 45 bpm or less: (1) within 20 min after a trotting-induced increase in heart rate to greater than 100 bpm, (2) within 40 min after a galloping-induced increase in heart rate to greater than 180 bpm (2 mi, or 3200 m), or (3) within 60 min after a breezing-induced increase in heart rate to greater than 210 bpm (3 to 5 furlongs, or 600 to 1000 m). If a horse’s heart rate exceeds these values, or is higher than it was following the same workout done previously, the horse should be examined carefully for evidence of musculoskeletal or internal pain, or a respiratory problem. If no cause for the higher heart rate is found, yet it is elevated even more following the next workout, the training should be lessened or stopped to allow recovery. Doing so will quite likely prevent the problem from becoming more severe and result in less loss of training and competition time. This, as well as a measure of physical fitness and, therefore, appropriate training, rest, and competition, are valuable benefits of and reasons for always monitoring the horse’s heart rate response to exercise or recovery.
Plasma Lactate as an Indicator of Fitness
The amount that the plasma lactate concentration ([lac]p) increases with exercise, or conversely the speed or exertion that increases the horse’s [lac]p to a certain level is one of the best indications of the horse’s physical fitness or endurance capacity. The less the increase in the [lac]p with a specific degree of anaerobic exercise, or the greater the exercise speed or duration before a specific [lac]p is reached, the more physically fit the horse. Changes in peak lactate concentration after exhaustive exercise is also indicative of peak anaerobic power and endurance. However, fitness has no effect on the resting [lac]p or its rate of decline following exercise. These effects are well illustrated by the results of the study shown in Table 11-4. The rate of decline in the [lac]p following exercise, although not affected by physical fitness is hastened by physical activity as shown in Table 11-5.
The plasma lactate concentration ([lac]p) increases hyperbolically as a horse’s speed or degree of exertion increases. The speed at which the [lac]p first begins to increase is called the lactate or anaerobic threshold. This occurs in most horses at a heart rate above 140 to 150 bpm and at 50 to 60% of maximum oxygen consumption. At a heart rate of 170 to 220 bpm and greater than 90% of maximum oxygen consumption most horse’s [lac]p reaches a concentration of 4 mM/L and begins to increase sharply with small increases in speed or exertion. This is referred to as the onset of blood lactate accumulation (OBLA). Both are indicative of aerobic or endurance capacity and have been shown to be closely correlated to people’s performance in marathon races, but to have poorer correlation for middle-distance events which require more anaerobic capacity. The OBLA, which is more commonly used in horses than is the lactate threshold, has been shown to be well correlated to Standardbred trotter racing performance and to physical fitness. When the speed at which the OBLA occurs plateaus, the horse has probably reached its maximum endurance capacity fitness. The OBLA is obtained by three to four bouts of running the horse at different speeds for 2 to 3 minutes, or at the same speed for different lengths of time, and taking a blood sample after each run to determine at what speed or for what duration at a specific speed, the plasma lactate concentration first exceeds (4 mMl/L).
TABLE 11-4 Physical Fitness Effects on Quarter Horses’ Plasma Lactate Concentration (mM/L)
Unconditioned | Conditioned | |
Pre-exercise | 0.6 | 0.9 |
After 5 min of exercise | 3.0 | 1.1 |
Alter 10 min of exercise | 4.5 | 1.5 |
After 15 min of exercise | 20 | 10 |
TABLE 11-5 Postexercise Physical Activity Effect on the Horse’s Plasma Lactate Concentration (mM/L)
20 Min | 30 Min | |
Physical Activity | Following a 2-min run | |
Standing | 12 | 9 |
Walking | 9 | 5 |
Slow trot | 5 | 2 |
The exercise-induced increase in the [lac]p, like the exercise-induced increase in the submaximal heart rate, is increased not only by decreased physical fitness but also by the following factors:
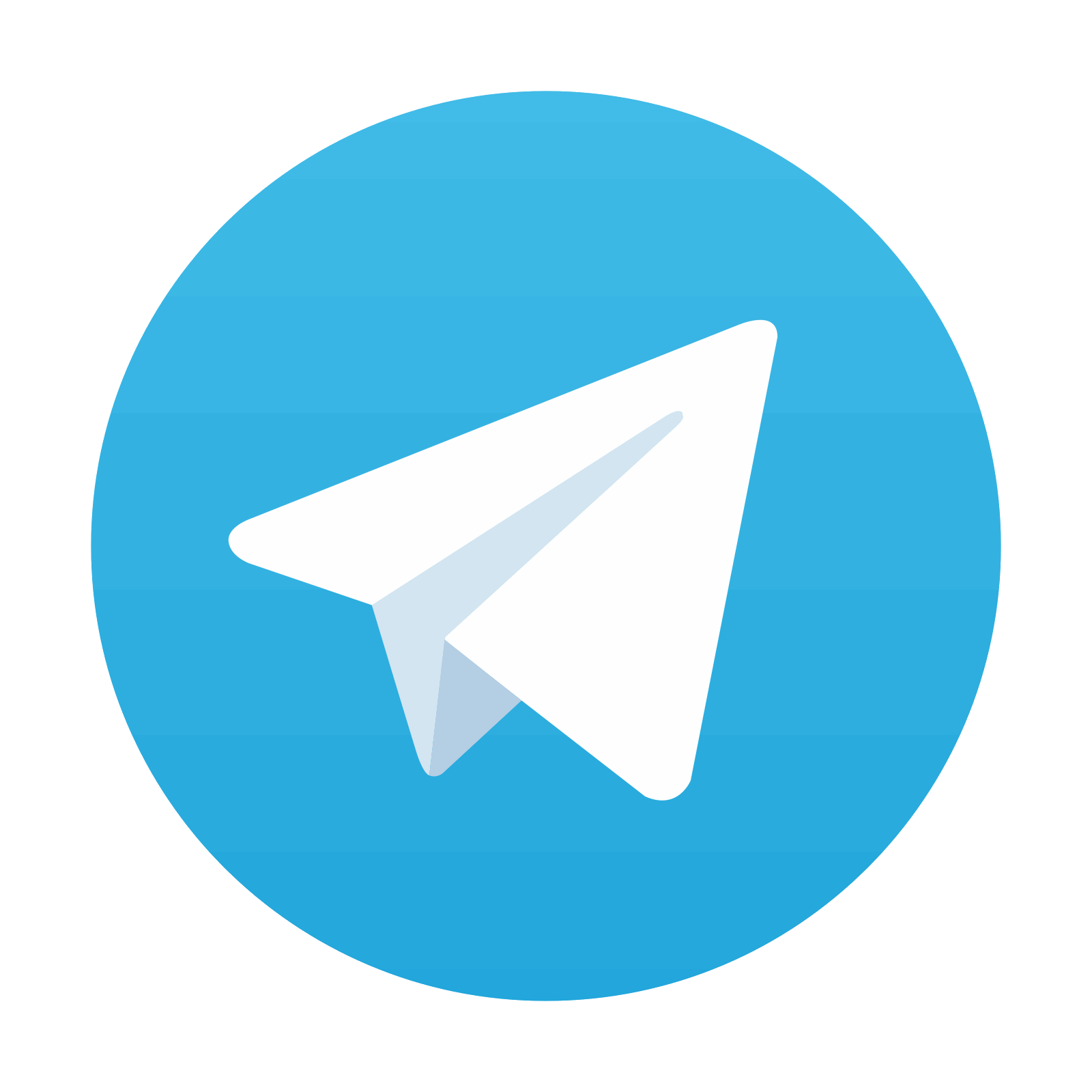
Stay updated, free articles. Join our Telegram channel

Full access? Get Clinical Tree
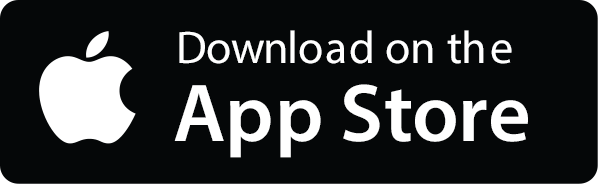
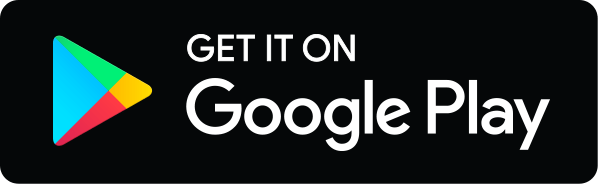