(1)
Indian Institute of Science Education and Research Pune (IISER-P), Pune, India
Abstract
The rapidly increasing epidemic of obesity has stimulated substantial research focused on fat metabolism. For the alternative model of T2D and related disorders that this book is developing, obesity is not as important and essential as the classical model presumes. By now the new argument is sufficiently clear on its stand on the relationship between obesity and T2D although a part of it is yet to be discussed. In the new emerging picture obesity is not causal to insulin resistance and T2D, but the behavioral factors that cause obesity and those that cause insulin resistance are largely overlapping. Therefore the association between obesity and insulin resistance leading to T2D is not surprising. But it is also not obligate. Physiologically it is possible (and also common in some populations) to be insulin resistant and eventually diabetic without ever being obese. Similarly it is also possible to be obese and still insulin sensitive. The two are independent but largely overlapping etho-physiological states. We will now examine this statement more elaborately starting for the basic functions of fat and why fat metabolism is linked with a diversity of other functions in the body.
The rapidly increasing epidemic of obesity has stimulated substantial research focused on fat metabolism. For the alternative model of T2D and related disorders that this book is developing, obesity is not as important and essential as the classical model presumes. By now the new argument is sufficiently clear on its stand on the relationship between obesity and T2D although a part of it is yet to be discussed. In the new emerging picture obesity is not causal to insulin resistance and T2D, but the behavioral factors that cause obesity and those that cause insulin resistance are largely overlapping. Therefore the association between obesity and insulin resistance leading to T2D is not surprising. But it is also not obligate. Physiologically it is possible (and also common in some populations) to be insulin resistant and eventually diabetic without ever being obese. Similarly it is also possible to be obese and still insulin sensitive. The two are independent but largely overlapping etho-physiological states. We will now examine this statement more elaborately starting from the basic functions of fat and why fat metabolism is linked with a diversity of other functions in the body.
It is quite well known that fats or lipids are storage molecules, and fat or adipose tissue serves as an energy storage tissue. Fortunately or unfortunately the word fat is used to denote both a kind of macromolecule and a kind of tissue that stores fat. I will make use of this ambiguity below while describing the seven functions of fat in our body. Some of the functions described below are of lipid molecules and some of adipose tissue. This distinction will be obvious as I elaborate on these functions. The important point to be emphasized here is that fat for the body is much more than storage of excess calories, and while talking about the causes and consequences of obesity, it is important to keep all of them in mind with due “weighting.” This is not intended to be an extensive and comprehensive review of the existing literature. Much comprehensive and scholarly work on obesity by several authors is available [1, 2]. I will avoid repeating what has already been said. Instead I will focus on aspects that these authors appear to have missed and those that are directly relevant to the synthesis in this book.
There is a very fundamental function of lipids, phospholipids in particular, in any living organism, and that is they form the outer and inner membranes of cells. Without this structural role of lipids, life would have been just impossible. But this is very basic for all forms of life, and it is taken for granted. I will not include this fundamental structural role of lipids while talking about the seven functions of fat. The seven functions of fat are:
1.
As an energy reserve
2.
As the most abundant macromolecular constituent of the brain
3.
As an insulator against cold temperatures
4.
As an impact buffer
5.
As a modulator of aggression, sex, and reproduction
6.
As an immune modulator
7.
As a social signal
Some of them are quite well known, others are less well known, and some are recent surprise findings. I will elaborate on each of them soon and show how each one is related in a different way to the four fundamental processes in fat dynamics, i.e., (1) lipogenesis, (2) lipolysis, (3) distribution of fat tissue in the body, and (4) effects of fat on other functions of the body.
1. Fat as an energy reserve: Excess energy left after meeting the current needs of the body is said to be stored, the common energy stores being fat. In this statement there is one fundamental assumption that appears to be uncritically accepted by everyone. That is, there is something like a fixed current requirement of the body above which it will be treated as excess and stored. However, the concept of current requirement of the body can be challenged. The current requirement is not a physiological constant but is a behavioral decision. An individual has a choice of what to do with energy. It is possible to spend an extra quantum of energy to go and chase more females, take another round of the territorial boundary to ensure there are no intruders, go and challenge one’s competitors, or just tease other group-mates. Storing as fat is only one of the many available options of what to do with energy. Which option is the best bet to increase one’s lifetime success is decided by ecological and social circumstances as well as by the personality of the decision maker. In terms of evolutionary optimization the available energy can be best utilized for the present if the situation is favorable to grow and reproduce and/or the future is relatively uncertain. On the other hand if the present circumstances are not so encouraging, there is a chance of the future being better and the probability for surviving till the next possible opportunity is fair; saving rather than spending is a better strategy. For example, if one’s reproductive opportunities are blocked by other more dominant individuals, it is no use spending time and energy in attempting to reproduce; it would rather pay to save energy and wait for a future opportunity. That is why lipogenesis is more adaptive under depressing or subordinate conditions. However, the most important condition necessary for storing for the future is that there is a good chance of surviving sufficiently long to make use of the stored energy. If predator or other environmental risks are large, it is better to spend as much energy as possible at the present and maximize immediate reproductive success. If the prospects for the future are to be counted, one needs to ensure survival before storing fat, and in order to do so, one should avoid all risk-taking behaviors till then. Therefore adiposity and risk-taking behavior have to develop an inverse association. Since physical aggression is necessarily coupled with risk of injury and perhaps death, obesity and aggression also need to be negatively related. Thus the very definition of excess energy on which the concept of energy storage is based is itself decided by ecology, social status, and behavior. Therefore believing in the popular notion that excess energy is stored as fat and ignoring the ecology and ethology of fat storage will not lead us to any insightful understanding of fat metabolism.
Leaving aside this consideration for the time being, let us return to the orthodox view and examine the concept of obesity as the result of net positive energy balance, i.e., calorie intake minus calorie burnt. Calorie burnt has two distinguishable components, the basic or resting metabolic rate and that due to physical activity or exercise. The former and even partly the latter are dependent on the total body mass. The greater the amount of respiring tissue, the greater the total fuel burnt. As a result, the body mass stabilizes at an equilibrium value of M. This is given by a simple baseline equation dM = I − aM − b, where I is energy intake, M is the body mass, a is the basal metabolic rate, and b the expenditure due to physical activity [3]. This equation can explain why at a given food intake and lifestyle, different people can have different body weights.
Unfortunately the reality is not as simple as the equation. What the equation does not incorporate is the influence of M on I. I is taken as an independent variable by this equation which is not true in reality. A large number of different mechanisms of the body regulate food intake and fat storage. There are two parallel lines of thinking and research which have failed to come together effectively. One is the energy balance equation given above which appears to neglect that a large number of mechanisms of intake regulation exist in the body and simply assumes that either a decrease in energy expenditure or increase in intake or both together are responsible for the obesity epidemic. If one or many of the intake regulation mechanisms were effective, neither of them could ever lead to obesity. And not only one but a series of mechanisms of food intake regulation exist which work at different levels (see Table 3.1). If there has to be a positive energy imbalance, not one but all the mechanisms would have to fail or be overridden, and as yet there is no suggestion as to how and why this is happening with increasing frequency only in the last couple of generations in a large and increasing fraction of the population.
We have seen earlier that a variety of at least partially independent mechanisms regulate food intake in the short [4–16] and long run [17, 18]. The body thus has adequate mechanisms to keep the short-term and long-term energy balance. Unless there is something wrong simultaneously with all these mechanisms, we will not eat excessively and will not accumulate fat. The short-term and long-term mechanisms of energy intake control appear to form a multilayered and robust system of regulation. It is difficult to perceive how such a system with a series of backups can fail to regulate food intake. If one system fails, others are there to compensate, and therefore, a single defect is most unlikely to cause an eating disorder. It is more likely that overeating represents an adaptive response. Being an evolved adaptive response, there would be mechanisms to modify the entire series of regulation mechanisms simultaneously. It is possible that the evolved mechanisms of overeating are facing supernormal stimuli today and therefore overexpressing now. In order to understand this we need to know under what conditions overeating would be adaptive in natural settings.
We have seen earlier that food is one of the major causes of aggression. This is certainly true for species having a patchy distribution of available food. For grass eaters, there need not be food-related aggression since the food is abundantly and widely distributed. For large carnivores a successful kill becomes a focus of intense competition and aggression. Frugivores also have a patchily distributed food although competition and aggression may be somewhat less intense than carnivores. On a food patch there is a complex interplay of aggression and food intake, and it is not a coincidence that common signal molecules such as serotonin are involved in regulating both the processes [11, 19]. We can visualize four possible scenarios on a food patch.
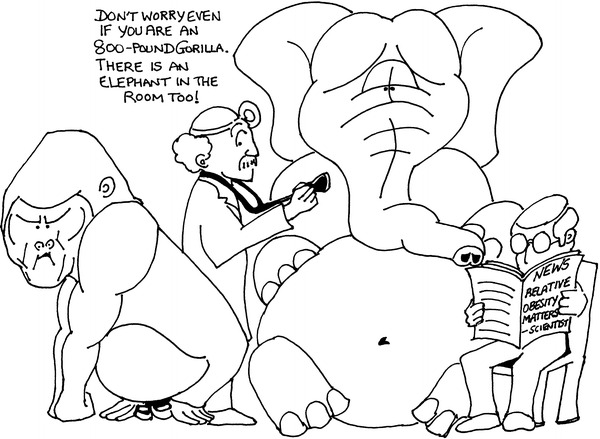
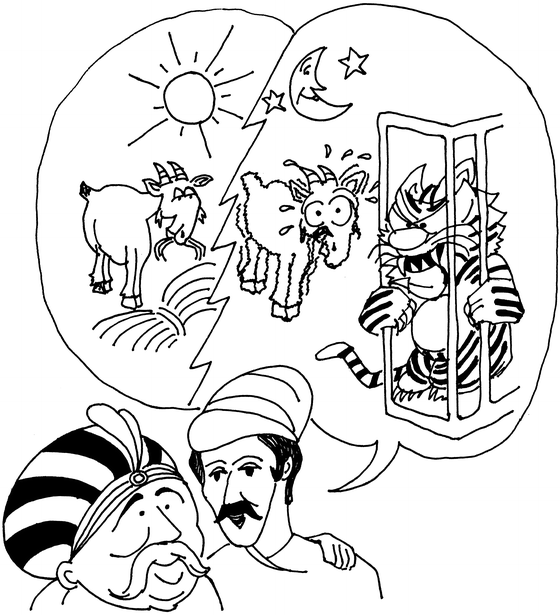
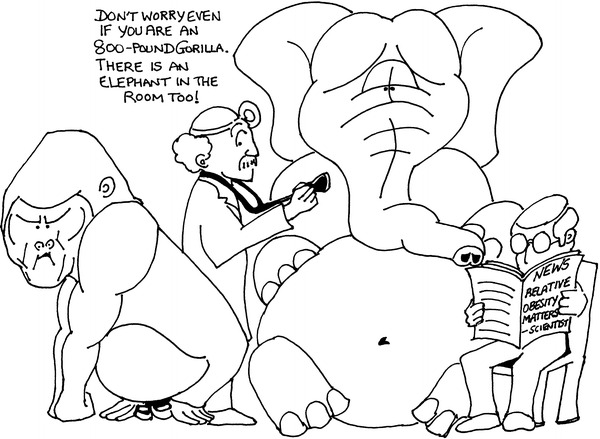
1.
There is competition and the opponents are weaker than you: Here there are clear advantages of being aggressive. You can drive away others and eat. Serotonin is expected to be low in this case. However, as you eat sufficiently, serotonin levels rise and aggression levels come down. At this stage, the competitors will become more active once again, and you retreat since your desperation for food decreases and you want to avoid risk of getting injured unnecessarily. Therefore you suppress aggression by increasing serotonin, and this suppresses hunger as well. This process ends up in moderate eating.
2.
There is competition and opponents are stronger: You need to suppress aggression by increasing serotonin and thereby partially suppressing hunger too. After waiting for variable time, you might get some share of the food, but you are unlikely to overeat.
3.
No competition: As a rare chance, you have no competitors on the food patch. This is a rare opportunity, and you should take maximum advantage of it. Since there is no competition, there is no need to suppress aggression. As a result serotonin remains low and satiety response is delayed. This is the only situation where overeating is possible and there is nothing wrong in overeating since such a situation is unlikely to come very frequently in a highly competitive environment. Therefore overeating response could be a natural adaptive response when food is abundant and there is no perceived chance of aggression related to food. As a result absence of aggressive competitors could have evolved a link with overeating response in species that normally have food-related aggression. It is unlikely to work the same way in species in which naturally there is no food-related aggression.
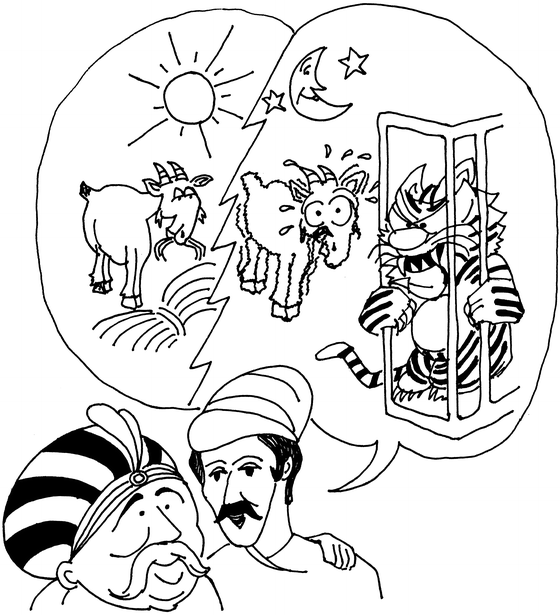
Another related factor is the risk of predators. In the wild, one has to forage for food. For species having predators, foraging often increases the risk of predators. Therefore there would be a trade-off between the nutritional benefits of foraging and the increasing risk of predation. The trade-off point is largely decided by the perceived risk of predation. If it is too high, then one should stop foraging after having the minimum intake needed for survival. Predators generally have a large home range and move over a large area. As a result, there is wide day-to-day variation in the risk of predation in any locality. When an animal perceives high risk, it is adaptive to arrest foraging efforts. When a predator is not perceived, it is equally adaptive to compensate by overeating. Thus eating behavior is intimately linked to perceived risk from predators and aggressive competitors. At a proximate level, this is mediated by aggression, anxiety, and fear signals in the brain. The list of regulators inhibiting food intake is considerably longer than that of appetite stimulators. Most of the peptides inhibiting food intake are also the ones mediating fear reactions, whereas the majority of the agents reducing anxiety responses stimulate appetite [20]. Of particular interest are the brain signal molecules, α melanocyte-stimulating hormone (α-MSH) [21–23], histamine [24–28], and cocaine- and amphetamine-regulated transcript (CART) [20, 29, 30], all the three being related to fear, anxiety, and risk avoidance on the one hand and decreased food intake on the other. Inhibition of histaminergic pathway is suspected to be important in hyperphagia and obesity [31]. It is very likely therefore that signaling by CART and histamine in the brain has evolved to fine-tune the trade-off between energy gain by foraging and predator or competitor aggression risk increasing with foraging.
A seventeenth-century Indian folktale has an interesting reference to body weight regulation. The emperor Akbar floated a riddle. He gave a goat to each village headman and ordered that the goat should be fed well, but after 6 months it should weigh exactly the same. Birbal, a famous wise man of his times, appears to have helped a village headman solve the riddle by an advice to tie the goat in front of a caged tiger at night and then feed the goat well during day time. He said the fear of the tiger will prevent the goat from being fat. We know today that fear induces peptides including CART, histamine, and alpha MSH which regulate food intake. Birbal obviously did not know the fear peptides but perhaps knew by observations and traditional wisdom that fear prevents one from overeating.
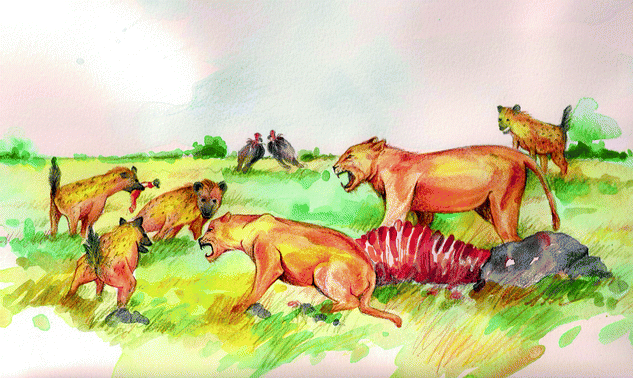
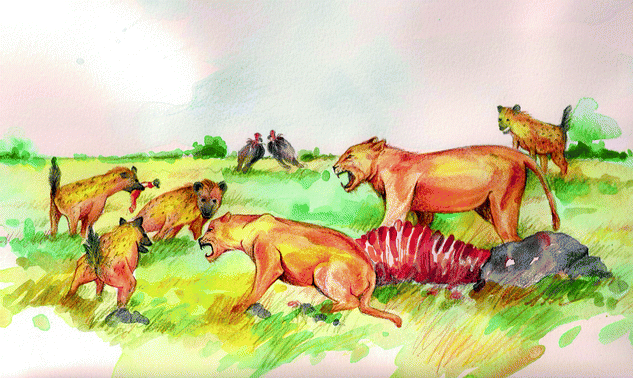
When a higher risk is perceived, higher levels of these signals are generated which will reduce foraging attempts and thereby food intake. Appetite control is a complex interplay between short-term metabolic signals such as insulin, long-term signals such as leptin, and behavioral signals such as CART and α MSH. The action of leptin is at least partially CART dependent, and CART levels are fine-tuned by perceived fear and aggression. It has been shown that the difference between diet-induced obese rats and diet-resistant rats (those that fail to become obese even when kept on a high-fat diet) lies in the CART and α MSH levels in the hypothalamus [32]. If CART and α MSH levels are low, food intake regulation fails and animals become obese. This may be the key to behavior-induced suppression of appetite. On the other hand if an animal is starving, or if leptin signaling is low indicating little stored energy, CART levels are depleted [33]. This impairs the fear response, and the animal is prepared to take greater risk for foraging. It is unlikely to be a coincidence that molecules involved in anxiety are also involved in appetite control. The leptin, CART, and α MSH interactions appear to have evolved in such a way to achieve an optimum trade-off between the metabolic demands of the body and the risk associated with foraging for food. In order to understand the interaction of these molecules in appetite regulation, it is necessary to know what is an optimum trade-off of food intake driven by perceived fear. It is necessary to make it clear at this point that this fear is necessarily physical. We have differentiated between soldier and diplomat anxieties in the last chapter whose physiological effects are expected to be different. Histamine, CART, and α MSH are shown to be driven by physical anxiety. So far, there is little data showing that diplomat anxiety triggers the same response, but this needs further investigation. We will continue with physical fear and anxiety in the discussions below.
The effects of predator fear and aggression anxiety can be incorporated in a formal model that gives good insights into what optimum food intake is from an integrated ecological and metabolic point of view. The model begins with the relationship of net energy intake per unit time such as a day and the evolutionary fitness contributed by this. In calculating net energy intake it is assumed that the energy expenditure in foraging, i.e., obtaining the food, is already subtracted. This relationship is most likely to go in a saturation curve with a maximum fitness that we will assume to be in unity. It is much like the famous Michaelis–Menten curve that every biochemistry student learns in the first year, with two differences. One being that it does not start from the origin. There is some basic energy needed for maintenance above which it starts contributing towards reproductive fitness. The other is that at very high intake the fitness may actually come down. Ignoring the latter for the time being, the curve will look as in Fig. 11.1. An equation for this curve can be written by adding a constant for maintenance energy I 0 to each of the terms in a Michaelis–Menten type of equation:
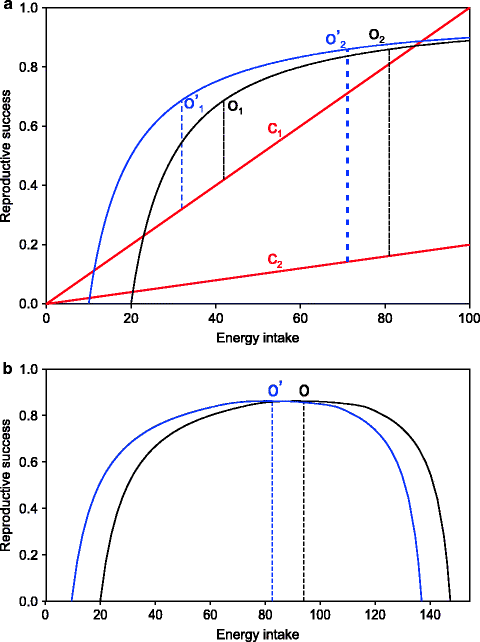
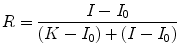
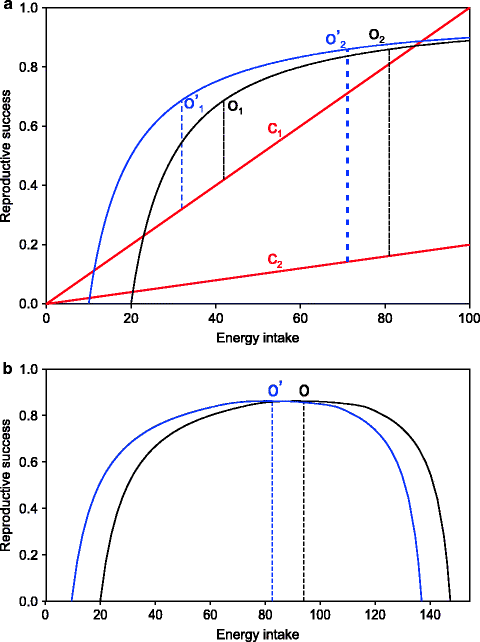
Fig. 11.1
(a) A foraging theory-based model of food intake optimization. The saturation curves represent the contribution of net energy intake to reproductive fitness. The straight lines with slope c denote risks associated with foraging. The optimum food intake O is one where the net benefit, i.e., the distance between the curve and the line, is maximum. This happens where the slope of the curve equals the slope of the line. It can be easily seen that when risk decreases (c1 to c2), the optimum food intake increases (O1 to O2). If the body has a preexisting energy reserve, the curve shifts left (blue line) and so does the optimum (O′1 to O′2). (b) Food intake optimum (O) without considering foraging risk: This is likely to develop for animal species like elephants that have neither predators nor food-related aggression. Here metabolic regulation is expected to prevail over behavioral regulation, and such species of animals are expected to be relatively resistant to developing obesity
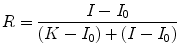
Since animals have to forage for food and the risk of predation or injuries from aggressive competitors increases with time spent in foraging, the risk will be positively correlated with energy intake. This is shown in the figure by the straight line with a slope c. The optimum food intake would be one at which the difference between the benefit and the risk is maximum. This happens at a point along the fitness curve where the slope becomes equal to c. Since the derivative of a curve is its slope, the optimum food intake I opt is obtained when


This is the optimum food intake per day that maximizes the net evolutionary fitness. It can be seen from Fig. 11.1 that c, the risk of foraging, is an important determinant of optimum foraging and thereby food intake. A small decrease in c can cause a disproportionately large increase in I opt. An assumption behind the model is that evolution will fine-tune the homeostatic mechanisms of the body to achieve this optimum. This is a calculation for a short-term optimum assuming that the animal has no reserve food. If there is a reserve biomass M, a part of M can be made available per day if the animal is undernourished on a given day. As a result the curve will shift to the left by a difference I m where I m is the reserve fat that can be mobilized in a day. This itself would be a saturating function of total body fat with an upper limit j. If the curve shifts to the left, the optimum food intake would also shift to the left. Thus there is a feedback from energy stores to food intake. Food intake I will in turn affect M by the well-known energy balance equation,
where a is the energy consumption per unit body mass for maintenance (basic resting metabolic rate) and b is energy expenditure related to activities other than foraging. The three equations above make an integrated dynamics, and it can be easily seen in this dynamics that a stable M is reached very soon and this steady state M is decided by the parameters of the equations namely, a, b, c, and j. Figure 11.2 shows the relative effects of changes in the four parameters. It is interesting to note that b, which represents physical activity or exercise, has the least effect on body mass. Fat oxidation rate is the most effective fine-tuner, whereas foraging risk and basic metabolic rate per unit body mass have almost the same effect.
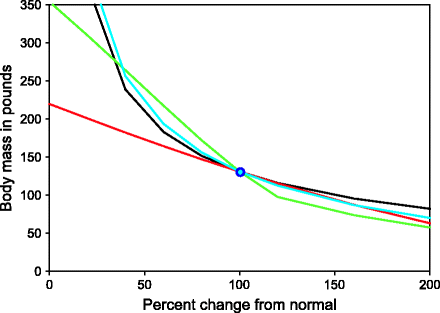
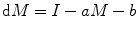
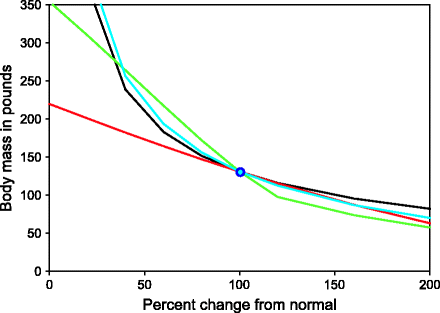
Fig. 11.2
The relative effects of four possible factors that can influence equilibrium body weight. The figure is based on simulation results from the model. An equilibrium point at moderate values of all parameters was set as standard. Percent change in each parameter and its effect on the equilibrium body weight are represented. Close to the normal body weight, exercise (red line) appears to have the minimum effect on body weight and the rate of fat oxidation (green line) has maximum. Risk of foraging (blue line) and basal metabolic rate (black line) also have large effect sizes
The difference between the classical energy balance equation and our model is that we have incorporated the feedback effect of M on I. It is also important to note that in our model c, the risk or the nonenergy cost of obtaining food is one of the most important determinants of food intake. In nature c is not constant and varies according to seasonal cyclic changes such as vegetation, visibility, migration, as well as purely stochastic factors. As a result individuals need to adjust the trade-off point between benefit and risk accordingly. They would eat more when predator risk is less and vice versa. Since long-term feedbacks in terms of effects of M are there in the system, the average c decides the mean M. However, what could we expect if the mean c changes? With decreasing nonenergy costs of food, there would be a dramatic rise in M. This is not a failure of homeostasis; instead, the desired set point of homeostatic regulation is shifted to the right, and the body has programs hardwired to attain the shifted desired level. This is one possible reason for the apparent “failure” of the homeostatic mechanisms in regulating obesity. This model suggests that this can happen when food-related risks disappear. This is the first level of ecological optimization of food intake that we will call risk optimization control. In the case of animals that naturally have high risk associated with foraging, removal of the risk will lead to obesity if food availability is not limiting.
What about species that naturally have a very low mean c? This may happen for animals such as elephant or rhino that are almost free of predators and food-related aggression. Many large ungulate species of the savanna that have predators but do not have a safe predator-free refuge will also have a small c since the difference in predator risk during foraging and non-foraging is not very large. In such animals c is unlikely to be important in the evolution of homeostatic mechanisms. Here we would expect that the deleterious effects of large M would shape the homoeostatic mechanisms to regulate food intake to keep an optimum M directly. This can happen by the short-term and long-term metabolic signals mentioned above. The short-term regulation works through gut and pancreatic signals including insulin, whereas long-term regulation works through leptin and other adipose-generated molecules. Let us call it metabolic regulation. In species where the behavioral regulation is frequently triggered by predation and aggression, metabolic control would not be needed most frequently. As a result metabolic control may not evolve to be strong. Moreover there is a reason why metabolic control will directionally evolve to be weaker. If predator risk is high, the behavioral optimum is always lower than the metabolic optimum. This will eventually lead to loss of weight. However predator risk is variable. Therefore whenever no risk of predator or aggressive competitor is perceived, an animal can make up by eating more. For such a compensatory hyperphagia to work, the metabolic regulation needs to be weaker and overridable. It appears necessary therefore that in species in which behavioral control of food intake prevails, metabolic control would evolve to be weaker and surmountable. The environmental cue for overriding the control mechanisms would be absence of fear and aggression. The mediator of this overriding is likely to be downregulation of CART, which can weaken the action of normal food intake regulators such as leptin.
In species where behavioral control never works in its ecological setting, metabolic control would be strong and almost infallible. A testable prediction of this would be that animal species that have neither predators nor food-related aggression will not show a tendency to become obese even when food availability is high. A possible example is elephants. So far, I have not been able to put together elephant morphometry data from various sources along with dietary and ecological data for each individual elephant. But such data do exist scattered over various sources, and therefore, the prediction is testable. Having spent a few years in the company of wild and captive Asiatic elephants, I have an impression that morphometric standards in elephants are highly reproducible and the variance around them is relatively small both in the wild as well as in captivity. Although a number of elephants in captivity are given food with high sucrose, other soluble carbohydrates, and oils, they do not drift substantially from the morphometric standards. There is no published data on obesity in elephants, but I have not seen rapid and uncontrolled weight gains in elephants, comparable to what happens to carnivores or primates in captivity. Obesity in carnivores and primates in captivity and occasionally even in the wild or semiwild state is not uncommon. Zookeepers know that the amount of food provided for carnivores needs to be carefully controlled, but excess of food does not matter so much for grass eaters. It does matter for primates that are predominantly fruit eaters. The distinction may not lie in diet since elephants in captivity are given fruits, sugarcane, and coconut which do not appear to lead to obesity.
The ancestral humans had a patchy distribution of food, whether scavenged, hunted, or gathered; therefore, a carnivore-like response is more likely to have evolved. Human ancestors had predators until recently, and the cultural development of death rituals is likely to have discouraged predators according to one theory [34]. Speakman also considers predators to be responsible to arresting obesity for a different reason [35]. Obese individuals are likely to be more susceptible as well as more attractive to predators, and therefore, evolution would strengthen energy regulation mechanisms for species with predators. This reasoning is compatible with our model with some differences. Speakman thinks that after being free of predators, a process of genetic drift increased obesity in humans. The above model says that being free of predation would reduce the risk of foraging c and thereby increase the optimum body weight. In human ancestors food-related aggression was also present to varying degrees almost throughout history. Food could have been one of the causes of wars and fights throughout human history. In hunter-gatherers foraging often involved driving away competing animals. Protection of crops, stored grain, and livestock from wild animals is a major aggressive activity even today in areas of residual wildlife. However, in modern society, an urban consumer is almost never exposed to food-related aggression. Therefore the c of the model in today’s society is almost zero. This makes the risk optimization-related food intake regulation driven by CART and histamine completely ineffective. We have seen above that in species where behavioral regulation is important, metabolic regulation would be partially degenerate. It is possible therefore that the human species is primarily a behavioral regulation species, not a metabolic regulation species. In modern lifestyle behavioral regulation factors have vanished, and metabolic regulation was always weak. Therefore energy intake regulation is failing in modern life.
Another result of the model is of considerable interest to us since it matches very well with reality. The parameter I m which reflects the maximum energy that can be made available for the body from the stored energy per unit time is an important determinant of obesity. The prediction of the model is that the basal metabolic rate or fat oxidation rate has similar effects on M but exercise has a much smaller impact on obesity (Fig. 11.2). For a long time a popular belief has been that obese and non-obese individuals differ in the basal metabolic rate, i.e., a of this model. Although the model supports this, evidence does not appear to give unanimous support to this idea [36, 37]. Exercise is always advocated as an antidote on obesity, but according to the model, exercises have limited efficiency in reducing body weight. Individuals differ dramatically in their tendencies, some rapidly gaining weight in spite of exercises and others remaining lean without any exercises. More robust data relate fat oxidation rate with obesity. Low rate of fat oxidation appears to be the main difference between people of obese versus lean tendencies [38–42]. The traditional models of energy balance equation or homeostasis have not been able to incorporate the rate of fat oxidation in their models which our model does effectively.
The rate of fat oxidation is also aggression dependent by a mechanism that is quite well worked out. One of the main mechanisms that cause rapid lipolysis is sympathetic stimulation [43–45]. Sympathetic stimulation is triggered in a flight-or-fight response. We have seen while discussing stress that flight or fight is typically a soldier response. Moreover the sympathetic activation is received by the adipose tissue by β adrenergic receptors [43], and the formation and distribution of these receptors is testosterone dependent [46]. Therefore testosterone arrests visceral fat accumulation [47–49]. Typical diplomat personalities have lower levels of testosterone [50], and therefore even if they happen to activate a sympathetic response, it does not lead to lipolysis as much as in a high-testosterone individual. Sympathetic nerves also appear to regulate the proliferation of preadipocytes since denervation of fat pad has been shown to increase the number of preadipocytes and weight of fat pad in rats [51–54]. This is another possible mechanism by which absence of aggression could facilitate obesity.
This also partially explains the distribution of fat in the body. The question as to why the distribution of fat is different in different persons remains largely unanswered although the differential effects of fat accumulation in different parts of the body are well known [55–61]. Since the same blood carrying the same concentration of fatty acids and hormones is circulated throughout the body, why does fat deposit in one adipose depot more than another? The answer certainly does not lie in hormones and metabolites since the same blood carrying these molecules circulates to all fat depots. A possible logical answer is nerve supply. Different adipose depots are innervated differently [62]. For example, abdominal visceral fat has an intricate sympathetic supply through the vagus nerve. Vagus does not innervate subcutaneous fat [63]. As a result, a combination of testosterone and sympathetic activation, both triggered by aggression, keeps on mobilizing visceral fat. This is how aggression changes the ratio of visceral to subcutaneous fat. There is no wonder then that sumo wrestlers have little visceral fat in spite of having large amount of total body fat. This is true as long as they practice wrestling. On retirement, their fat may go visceral rapidly, and they might become insulin resistant [64].
This is not the first time that a model is being used to explain obesity. However, the uniqueness of this model is that for the first time it brings together three different concepts, namely, energy balance equation, homeostatic control on energy intake, and foraging theory. Somehow, researchers had so far either not recognized the importance of bringing the three concepts together or had failed to do so, the former being the most likely case. But now we appear to be closer to the reality of how and why the homeostatic mechanisms evolved under ancestral conditions are failing in the modern lifestyle. There are a few more implications of the model. Both predation and aggression are population regulation mechanisms. Removal of predation increases prey population [65, 66], and reduced aggression also enables higher population densities [67]. Therefore if the predatory pressure as well as intraspecific aggression is suddenly removed in a species, there can be anticipation of crowding and thereby decreased food availability. Therefore it would be adaptive to increase thrift on removal of a fear signal. It is possible that because of this reason too, evolution might have built in a program to increase food intake on loss of predator fear and signs of population growth. For wild populations crowding is almost invariably associated with increased food competition, exerting a negative feedback control that would effectively arrest obesity. The problem with the current human condition is that the natural fear signals associated with foraging are absent, eating has lost its connection with foraging associated risks, there is increasing crowding which could be stimulating a thrift response, and there are no signs of reduction in food availability that naturally accompanies these conditions. If vanished risk of predation and food-related aggression anticipating crowding is at the root of these changes, it is logical then that reproductive strategies also change in this situation, and the reduction in fecundity with thrift is likely to be a response evolved for this reason.
Obese persons are often known to be lethargic. In the light of the model described above, it can be easily seen why lethargy is adaptive. Lethargy is not an inevitable result of storing fat. Extra energy makes one lethargic is paradoxical. Lethargy is more likely to have evolved as a feedback mechanism in energy homeostasis and risk minimization. If an individual has sufficient stored energy, it is unprofitable to keep on foraging and being exposed to risks. So lethargy increases the chances of survival in a wilderness environment by reducing foraging-related risks. Simultaneously lethargy decreases foraging and thereby food intake, contributing to effective feedback in energy homeostasis. If fat causes lethargy then the foraging feedback is almost infallible. It is only in the modern human society that feeding is detached from foraging. As a result, in spite of being lethargic, food intake may not be affected. In our hunter-gatherer ancestors the lethargy feedback must have been very effective.
There are other possible means by which evolved responses might be leading to overeating in modern life. There are reward centers in the brain that are important in regulating food intake [17, 68]. In the wilderness, the most common natural rewards activating reward centers are food, sex, and presumably occasional social rewards such as achieving dominance. The reward centers when activated by getting a sumptuous food reward initiate a satiety pathway and stop food intake [17]. The alternative rewards also trigger the same reward centers, and in most of these rewarding situations, temporary suppression of hunger is essential. For example, while having sex, hunger would be an interfering drive, and therefore, reward center activation by sex should also give satiety signals. Such an adaptive loop must have evolved. However, it is also important to put a limit on such nonfood reward suppression of hunger. So if the reward center is suppressed by nonfood rewards too often, there would be a temporary desensitization of the reward center. This would bring back the hunger response and, as a result of the partial desensitization, result into compensatory overeating.
It is very likely that this is happening in today’s monetary economics. In modern life money is perceived as a reward and is perhaps the most common nonfood reward of modern life. Money keeps on stimulating the reward center repeatedly. Neurobiological studies have shown that the brain areas activated by getting prize money and those activated by a sweet taste are the same [69–73]. Experimental psychologists have also demonstrated that there is a neuronal cross talk between food and money such that hunger affects food-related decisions and thinking about money affects food intake [74]. It is very likely that repeated activation of the reward centers by money over a long time results into chronic desensitization of the reward center resulting into habitual overeating. A downregulation of dopamine signaling is indeed demonstrated in obesity [75, 76]. There is a link with aggression here too. Aggression is linked to the dopamine reward pathway, and aggression increases dopamine activity [77]. This is perhaps one more mechanism by which aggression arrests obesity.
In an attempt to test the hypothesis that nonfood rewards affect obesity, we carried out a survey of people working as full-time cashiers in different types of organizations. Some of the cashiers were owners or partners in the endeavor, and others were only salaried cashiers. Since they were all doing physically very similar jobs, the levels of on-job physical activities could be assumed to be similar. There was an obvious difference between the reward values of the money being handled. For the owner cashiers the money had a strong reward value, whereas for the salaried cashiers, the reward value of the money they handled would have been at the most weak. The survey showed that owners had a greater BMI, waist circumference, and waist-to-hip ratio than salaried cashiers. Also, two other variables, namely, the amount of money handled per day and the duration of doing a cashier’s job, were also positively related with obesity, but the duration of doing a sedentary job and self-reported exercise or absence of it was not. The most important part of the study, in spite of its small sample size, was that this single behavioral factor explained about 20% of the population variance [16]. This is interesting on the background that genome-wide fishing for obesity-related genes has so far explained not more than 2% of population variance in obesity [78–83]. Yet researchers as well as laymen have a strong belief that obesity is genetic and are reluctant to believe that behavior could be a more important determinant of it.
The purpose of the above discussion was not to claim to have identified all factors and mechanisms responsible for overeating but to highlight that neurobehavioral processes are perhaps the most important contributors to it. Unless the neurobehavioral mechanisms that have evolved to face social and ecological conditions are clearly identified and addressed, all efforts to mitigate obesity are unlikely to work.
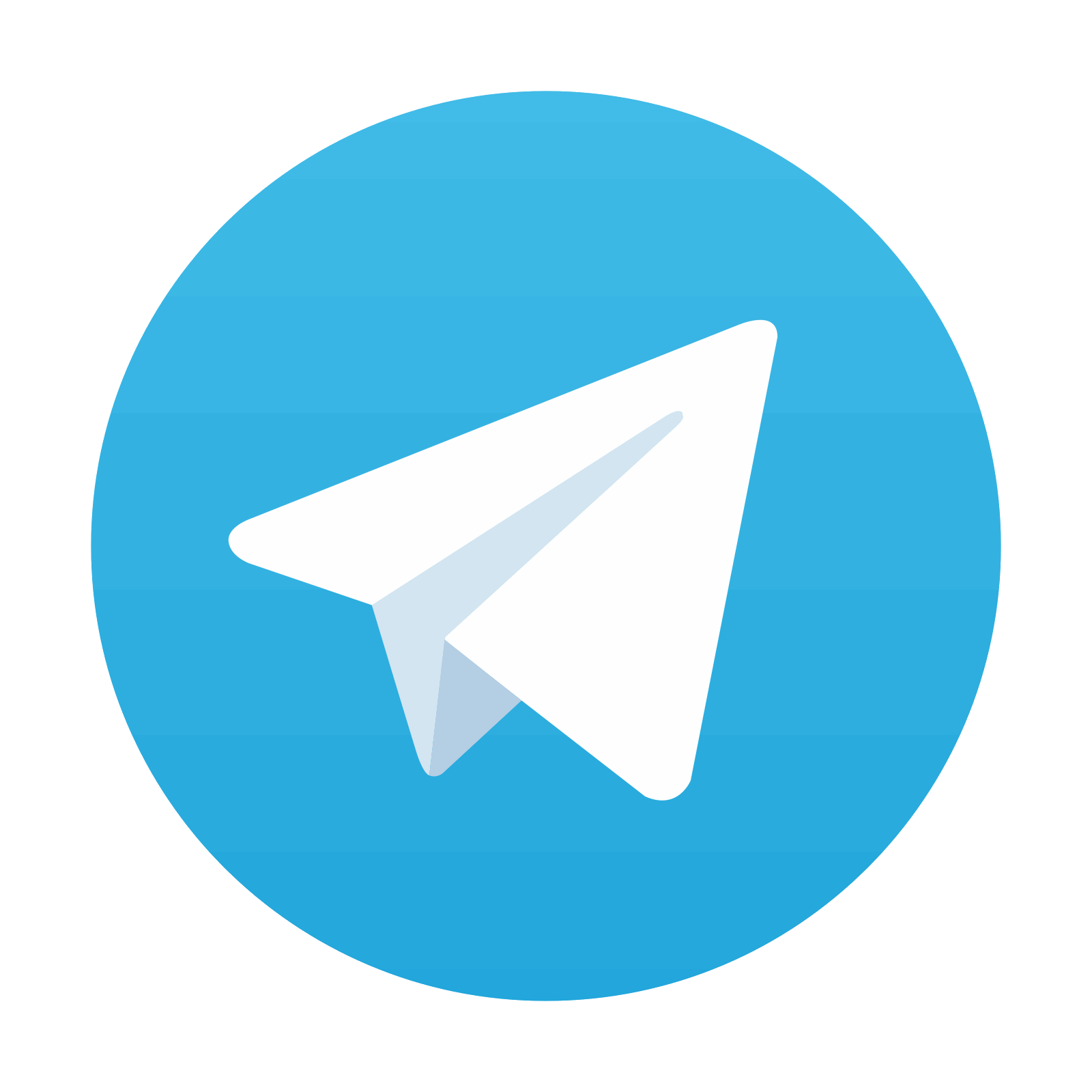
Stay updated, free articles. Join our Telegram channel

Full access? Get Clinical Tree
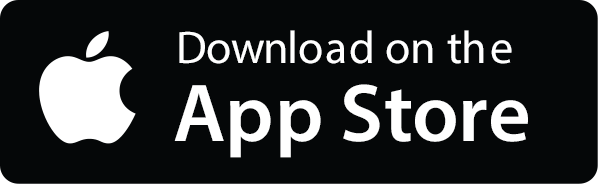
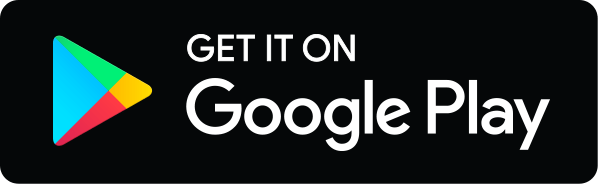