Revised from 6th edition of Veterinary Ophthalmology, Chapter 10: Part 10.1 – Ophthalmic Examination and Diagnostics, by Heidi I. Featherstone and Christine L. Heinrich; Part 10.2 – Ocular Imaging, by David Donoldson and Claudia Hartley; Part 10.3 – Diagnostic Ophthalmic Ultrasound, by Ellison Bentley, Stefana Pizzirani, and Kenneyh R. Waller; and Part 10.4 – Clinical Electrodiagnostic Evaluation of the Visual System, by Gil Ben‐Shiomo; and Chapter 11: Ophthalmic Genetics and DNA Testing, by Simon M. Peterson‐Jones An ophthalmic diagnosis is often made at the time of the examination, more often than in most systems, because most ocular structures can be visualized either directly or indirectly. Furthermore, the eye lends itself to numerous simple and efficient noninvasive diagnostic procedures, many of which can be performed during a routine examination. This chapter describes examination techniques and diagnostic procedures available to veterinarians, and those often employed by veterinary ophthalmologists. A thorough history, when combined with clinical findings, will support appropriate case management. In taking a history, the same considerations apply as to other body systems and include signalment, use of the animal, environment, diet, characterization of the primary complaint (onset, initial clinical signs, progression, duration), treatment (response and current treatment), and nonophthalmic disease (previous and concurrent). Additional information that may be helpful includes travel history, vaccination, and deworming history, and whether other animals in the vicinity are similarly affected. More specific information pertaining to the eye includes the owners’ interpretation of vision, pain, and whether one or both eyes are involved. Further information may be required depending on the primary presenting problem. Signalment can provide an important clue to the cause of many ophthalmic conditions; these clues include conditions that are specific to a particular species (e.g., corneal sequestrum in the cat), those that are associated with a particular coat color (e.g., multiple ocular anomalies in the homozygous merle dog), those with strong breed predispositions (e.g., keratoconjunctivitis sicca [] in the West Highland White Terrier), and others known to be hereditary (e.g., cataract in the Morgan horse). Primary complaints from the owner usually include ocular discharge, change in appearance of the eye (size, color, pupil), or reduced vision or blindness. The role of concurrent disease or secondary complaints should not be underestimated. Concurrent systemic disease may also affect treatment; for example, timolol, a topical β‐blocker, should be avoided in cats with both asthma and glaucoma. Intensive therapy with topical atropine can induce mydriasis for several days up to 14 days in the horse. Questions should be directed toward vision in different situations: photopic and scotopic conditions, i.e., hemeralopia (day blindness) versus nyctalopia (night blindness); and stationary and moving objects, i.e., central versus peripheral retinal disease. Owners are often poor judges of ocular pain. They may notice classic signs of ophthalmic pain – watery discharge (increased lacrimation), closed or small eye (blepharospasm), and photophobia, but do not interpret them as such. The ocular examination should proceed in a logical and systematic manner. The general order of steps is listed in Box 4.1, but the method will vary according to personal preference, available equipment, and the individual case. However, certain diagnostic tests and observations must precede others to avoid interference with results and interpretation. Important tests influenced by previous diagnostics include the Schirmer tear test (STT), the evaluation of pupillary light reflexes (PLRs), instillation of topical anesthetics, and tonometry before the administration of mydriatic agents. The place of the ophthalmic examination varies with the species, but the illumination must be dark enough to prevent undue corneal reflection but not too dark to cause risk to the examiner and assistants! Regardless of the species, it is essential to have a well‐lit area for the initial examination and the option to darken the environment for subsequent steps. The examination area should also be quiet and away from major distractions. An appropriate examination area minimizes animal and examiner stress, which in turn maximizes cooperation levels and helps to reduce examination time. Small animals can usually be examined on a table in a closed room; large animals require more specialized facilities, e.g., stocks or stalls for horses, and chutes or stanchions for cattle. The best method of restraint for any given situation varies with the species involved, the patient’s temperament, and the desired effect. Generally, however, the least amount of restraint possible is best for the ophthalmic examination. Most dogs and cats require only gentle manual restraint for an ophthalmic examination. Fractious animals may require additional restraint. Muzzles can be employed with aggressive or fearful dogs, but their use is limited in brachycephalic breeds. A large towel or blanket or a cat bag can “wrap up” a fractious cat and is a minimally invasive way to avoid claw injuries to the examiner or owner. When sedation or general anesthesia is necessary, there can be side effects. Many anesthetic agents and sedatives decrease tear production, or alter intraocular pressure (IOP) in most dogs; deep sedation and general anesthesia frequently make the ophthalmic examination more difficult because the eyes tend to roll downward (infraversion) and the nictitating membrane tends to protrude. Ketamine with or without diazepam in cats results in a loss of the blink reflex, which can lead to corneal drying and mydriasis, which alters the PLRs. Ketamine can cause nystagmus and, in some species, will elevate IOP. Acepromazine may cause nictitans protrusion, which obscures the globe during evaluation. Opioids may alter pupil size, reactivity, and IOP. Specifically, opioids tend to produce mydriasis in those species that exhibit central nervous system (CNS) excitation and miosis in those that become sedated after opioid administration. Although eye exams in horses can be accomplished without chemical restraint, these drugs markedly facilitate the complete eye examination without undue stress on the horse and with greater protection for the owner and examiner. Detomidine hydrochloride 0.02–0.04 mg/kg i.v. is the chemical agent of choice as it provides rapid onset of tranquilization without an excitatory phase, and a steady and low head position without movement. Xylazine, 0.5–1.0 mg/kg i.v., has also been recommended to facilitate equine ocular examination; it may be necessary to add butorphanol 0.01–0.02 mg/kg i.v. or acepromazine 0.02–0.04 mg/kg i.v. for painful procedures or additional restraint. Dairy cattle can usually be adequately restrained in a stanchion, with the head pulled laterally by an assistant using a nose lead. Beef cattle may be more difficult to restrain, and squeeze chutes with special head restraints are often necessary. Sheep and goats can usually be restrained manually with the help of an assistant. Goats can be examined standing, and sheep can be examined seated on their rumps to avoid excessive movements. Llamas and alpacas may need to be supported in a chute or a sling. Rodents, rabbits, birds, and small exotic species can usually be restrained manually. Rabbits are usually adequately restrained on a table with a handler supporting the hind limbs with one hand and the back or neck with the other. Most small‐ to medium‐sized birds can be examined safely when properly restrained with a hand about the neck and feet, with wings tucked in or wrapped within a towel. Leather working gloves facilitate handling of raptors and other large birds. Ferrets are usually simply and sufficiently restrained by suspension from the neck scruff with legs dangling free. The orbicularis oculi muscle closes the eyelids and thus can impair or even prevent ocular examination, especially in large animals. Akinesia of this muscle is routine in the horse, occasionally required in cattle, and only rarely performed in small animals. Most horses require an auriculopalpebral block for a thorough ocular examination, especially if the eye is painful or if the structural integrity of the globe (usually the cornea) is compromised. The palpebral nerve, which arises from the auriculopalpebral branch of the facial nerve (cranial nerve VII), innervates the orbicularis oculi muscle. These motor fibers may be blocked at any point between the origin of the auriculopalpebral nerve and the palpebral nerve branch, but blocks administered closer to the origin of the nerve are most effective. There are three main points at which the auriculopalpebral or palpebral nerves can be blocked in the horse (Figure 4.1). Figure 4.1 Horse skull depicting sites for the auriculopalpebral (a and b), palpebral (c), frontal/supraorbital (d), lacrimal (e), zygomatic (f), and infratrochlear (g) nerve blocks. Akinesia can be achieved in a similar fashion in cattle. The auriculopalpebral nerve can sometimes be palpated as it passes over a palpable notch in the zygomatic arch at the level of the temporomandibular joint. If the nerve cannot be palpated, anesthetic may be injected subcutaneously along the zygomatic arch, starting at the supraorbital process and continuing approximately 3.75 cm caudally. The auriculopalpebral nerve block is usually necessary with other retrobulbar nerve blocks in cattle for enucleations. In the dog, the auriculopalpebral nerve can be located and blocked along the most lateral part of the zygomatic arch, at the midpoint of the caudal third of the bone. Alternatively, terminal palpebral nerve fibers may be blocked as they pass over the orbital rim. The most commonly applied form of anesthesia for the eye is the application of topical anesthetic to the cornea, which is routinely used during ocular examination, specifically to facilitate the assessment of painful eyes, the measuring of IOP, and for obtaining of laboratory samples. In the dog, the application of a single drop of 0.5% proxymetacaine resulted in the loss of sensation for 55 min, with a maximal effect of 15 min, while the application of a second drop of the drug increased the maximal duration of corneal sensation loss to 25 min. The use of a 1% tetracaine and 0.4% oxybuprocaine preparation showed a fast onset of maximum corneal anesthesia within 1 min and a significant loss of sensation for a duration of 45 min; however, it can be irritating to some individuals. In the horse, maximal corneal sensation loss is noted 5 min following the application of a single drop of 0.5% proxymetacaine and the duration is approximately 25 min; however, full loss of corneal sensation is not achieved. Several different sensory blocks may be employed in large animals depending on the location of the eyelid lesion to be addressed (see Figure 4.1) (the anesthetic agents used are the same as those described earlier for induction of eyelid akinesia): The medial canthal region is innervated by the infratrochlear nerve and is desensitized by injecting anesthetic agent through the bony trochlear notch on the dorsal rim of the orbit near the medial canthus Retrobulbar anesthesia aims to block cranial nerves III, IV, V, and VI and the ciliary ganglion, and therefore provides loss of sensation to the globe and eyelids as well as extraocular muscle akinesis. In the horse, retrobulbar blocks are commonly used to allow surgical manipulation of the eye and adnexa in order to avoid general anesthesia. The use of combined sedation and retrobulbar block is well established for enucleation in the standing horse. The retrobulbar block has been shown to prevent heart rate decrease associated with the oculocardiac reflex during enucleation. Retrobulbar blocks are also used in equine patients undergoing general anesthesia to provide extraocular muscle akinesia during cataract surgery, thereby reducing the need for nondepolarizing neuromuscular blocking agents (NMBAs) and ventilation. In small animals, retrobulbar injections are most commonly used to augment analgesia for enucleation procedures, and to replace the need for NMBAs to provide immobilization and optimal exposure during intraocular surgery. Various techniques have been described for achieving retrobulbar anesthesia. In the dog, the most commonly employed approach is the inferior temporal technique in which an approximate 20° angle is created by bending a 2‐gauge, 1.5‐in. spinal needle at its midpoint. The needle is then positioned at the ventral orbital rim and inserted through the lower lid at the junction of its middle and lateral thirds. The needle is advanced until a slight “popping” sensation is detected, indicating that the orbital fascia has been pierced. The needle is then directed slightly dorsally and medially toward the apex of the orbit and advanced approximately 1–2 cm. Then, 1–2 ml of a suitable local anesthetic (e.g., lidocaine, bupivacaine, mepivacaine, or ropivacaine) is injected after gentle aspiration to check for blood to avoid intravascular injection. In the cat, a dorsomedial approach for retrobulbar anesthesia has been shown to be more reliable than the inferotemporal approach recommended for dogs. In cats, the available retrobulbar space for an intraorbital injection is very limited. With the cat in sternal recumbency, a 1.5‐in. (38‐mm) spinal needle is bent at the midpoint to achieve an angle of approximately 20°. The needle is then inserted through the superior eyelid at the medial region of the orbit (at the junction between the medial and middle third of the eyelid) and advanced approximately three‐quarters of its length (30 mm) toward the caudal pole of the globe, but in close proximity to the orbital wall. In the horse, several methods have been described: More recently, alternatives to retrobulbar anesthesia, including peribulbar injection and sub‐Tenon’s methods, have been explored for veterinary patients. In peribulbar anesthesia, the needle is introduced into the extraconal space (i.e., outside the extraocular muscle cone), thereby limiting risk of injury to intraconal structures, especially the optic nerve and major blood vessels supplying the globe. The injectate spreads throughout much of the orbit, including the intraconal space. Sub‐Tenon’s anesthesia in the dog and horse is described via the mediodorsal area of the bulbar conjunctiva, which is incised with ophthalmic scissors 5 mm from the limbus. Closed scissors are introduced through the created aperture and the sclera is exposed by blunt dissection through Tenon’s capsule. A human, curved, blunt 19‐gauge, 25‐mm sub‐Tenon’s cannula is inserted along the equator of the globe until it reaches the posterior sub‐Tenon’s space, where the local anesthetic is infused. Initial examination is performed in ambient lighting and without instruments. This is an important stage because it can be very informative. Examination is started from a distance, before handling the animal, and is followed by close examination. The animal’s attitude, general body condition, and ability to navigate in an unfamiliar environment are carefully evaluated to provide some information about its general health and vision. Conformation of the orbit and periocular region, and the size, position, and movement of the eyes should be assessed with particular note of asymmetry. Abnormalities in globe size (e.g., microphthalmia), buphthalmos from changes in globe position (e.g., enophthalmos and exophthalmos), and any type of ocular discharge should be noted. The cranial nerve examination is an inherent and essential part of any thorough ocular examination. Specifically, cranial nerves II–VIII are assessed via the menace response, dazzle, pupillary light, palpebral, corneal, and vestibulo‐ocular reflexes (VORs). Vision can be assessed by the menace response, tracking reflex (cotton ball test), visual placing reflex (for small animals), and maze test (in photopic and scotopic conditions). The menace response is part of a routine ophthalmic examination. A menacing movement toward the eye results in closure of the ipsilateral eyelids, and possibly globe retraction or an avoidance head movement. The menacing gesture should be directed toward different visual fields to gather information about partial visual deficits. The menace response is an acquired protective cortical response. It is absent in puppies and kittens, and should be acquired by approximately 12 weeks of age in these species. In foals, the menace response should be acquired by 2 weeks of age, although some reports have described a positive menace response between 5 days and 19.5 weeks, and by 9 days. The menace response is inconsistent in cats, birds, and some exotic species. The resting pupil size should first be observed in normal light and then in a darkened room. Pupil size and asymmetry can be accurately assessed by distant direct or indirect ophthalmoscopy; the PLR, tested by shining a bright focal light source at the eye, results in constriction of the ipsilateral pupil (direct PLR), and in some species, constriction of the contralateral pupil (indirect or consensual PLR). The PLR evaluates the retina, cranial nerve II, midbrain, and cranial nerve III (parasympathetic fibers). An animal with cortical blindness can have a normal PLR because the pupillomotor fibers branch from the optic tract before the visual fibers. The equine PLR is biphasic, i.e., an initial brisk but small reaction followed by a slower complete constriction. The direct PLR is difficult to assess in birds because intermittent dynamic anisocoria can be a normal feature; this results from (presumptive) voluntary control of the striated component of the iris musculature and the bird’s emotional state. The indirect PLR is only present in species with partial decussation of the optic nerve fibers at the optic chiasm (e.g., 33% in the cat, 75% in the dog, and 85% in the horse). In species with more than 50% decussation, the indirect PLR is weaker than the direct PLR because more efferent pupillomotor fibers return to the ipsilateral side of the brain (dynamic contraction anisocoria). The “swinging flashlight test” is a modification of the PLR. A focal light source is passed repeatedly from one eye to the other, causing the pupil under direct illumination to constrict slightly more than the contralateral pupil. A unilateral prechiasmal lesion (i.e., involving the retina and/or optic nerve) will result in a pupil constricting when the fellow eye is illuminated, but subsequently dilating under direct illumination (a Marcus–Gunn pupil). It is, however, important to note that pupillary escape, a slight dilation that follows constriction under direct light stimulation, is normal in many species. The dazzle reflex is a subcortical reflex in which a bright focal light source shone quickly into the eye results in closure of the ipsilateral and, to a lesser extent, contralateral eyelids. The complete anatomical pathway for the dazzle reflex is unknown, but the retina, cranial nerve II, rostral colliculus, and/or supraoptic nuclei of the hypothalamus, and cranial nerve VII (innervating the orbicularis oculi) are involved. Being a subcortical reflex, in contrast to the cortical menace response, the dazzle reflex is present from birth. Sufficient brightness of the light source is crucial for this test to be performed reliably. The dazzle reflex is especially helpful to evaluate retina and optic nerve function when the PLR cannot be assessed (e.g., hyphema and severe corneal edema). The palpebral reflex (blink reflex), tested by lightly touching the lateral and medial canthi, results in eyelid closure. The afferent arm of the palpebral reflex is the ophthalmic branch of cranial nerve V, and the efferent arm is cranial nerve VII. As for the menace response, the palpebral reflex should be performed before sedation or eyelid akinesia. The corneal reflex is one of the most sensitive reflexes in the body and its purpose is to protect the eye. The corneal reflex, tested by touching the peripheral cornea with a sterile swab tip or wisp of cotton wool, results in retraction of the globe and closure of the eyelids (Figure 4.2). The afferent arm of the corneal reflex is the ophthalmic branch of cranial nerve V, and the efferent arm is mediated by cranial nerves VI (globe retraction) and VII (eyelid closure). Veterinary ophthalmologists can quantify corneal sensitivity using the corneal esthesiometer. Figure 4.2 Corneal reflex. Corneal sensation is tested by means of a wisp of cotton wool contacting the peripheral cornea. The VOR assesses eye movement in relation to changes within the vestibular apparatus. It thereby indirectly assesses the three cranial nerves (III, IV, and VI) that innervate the extraocular muscles, and cranial nerve VIII and the medial longitudinal fasciculus that coordinate movement. The animal’s head is moved from side to side and up and down. During head movement, the eyes will attempt to maintain the last eye direction, i.e., where the animal was looking at the start of the test. This results in a slow movement of the eyes away from the direction of head movement. As the head continues to be moved, the extraocular muscles, influenced by the vestibular system, move the eyes quickly in the same direction as the head movement. The eyes should move in unison and should stop moving when head movement is stopped. The VOR is more difficult to perform in large animals. At this point in the examination, or even before some of the neuro‐ophthalmic tests, tear production should be measured using STT I. This is to avoid falsely high values from excessive manipulation of the eye and/or eyelids (inducing reflex tear production) and/or the administration of any topical agents (e.g., stains, anesthetic agents, and mydriatic agents). Following initial distance examination, the animal can be handled gently, with minimal manipulation of adnexal tissues. The orbit should be examined by palpation of the bony orbital rim and indirectly by retropulsion of the globe through the closed upper eyelid. A more detailed assessment of the orbit includes manipulation of the mandible and oral examination (this can be deferred to minimize stress in the early stages). As the coronoid process of the vertical ramus of the mandible is closely associated with the floor of the orbit, jaw opening may cause pain or resistance if orbital disease is present. A careful examination of the oral cavity should follow. Eyelids should be examined in more detail in terms of position, mobility and conformation, and skin lesions. In some animals, it is important to assess eyelid conformation with the head in different positions. Close inspection of the eyelid margins allows observation of the cilia (eyelashes) and meibomian gland openings. The eyelids differ greatly between species. The upper eyelid is more mobile in mammals, whereas the lower eyelid is more mobile in birds and many lower vertebrates. Birds have a tarsal plate in the lower eyelid and a well‐developed, almost transparent third eyelid. Complete fusion of the upper and lower eyelid forms the spectacle in snakes and geckos. The eyelids, conjunctiva, and third eyelid should be examined in one almost continuous movement: the upper eyelid is retracted to examine the dorsal bulbar conjunctiva; the eyelid margin is everted to examine the upper palpebral conjunctiva and the upper nasolacrimal punctum; and the third eyelid is protruded by applying gentle pressure through the upper eyelid to retropulse the globe and enable examination of the anterior surface, leading edge, and alignment of the third eyelid. Finally, the lower eyelid is retracted to aid visualization of the lower conjunctival fornix and nasolacrimal punctum. The leading edge of the third eyelid is usually pigmented. The lacrimal caruncle lies just inside the medial canthus; it appears as a small mound covered with conjunctiva and, in some animals, fine hairs. It is more prominent in ungulates than in carnivores. A preliminary assessment of the nasolacrimal system is made at this stage. Tear overflow (epiphora) is noted and can represent either increased tear production or decreased tear drainage. Increased tear production is typically caused by ocular surface irritation (e.g., ectopic cilia) or pain (e.g., anterior uveitis) and can be objectively quantified by STT I. Tear drainage may be reduced or absent as a result of partial or complete obstruction of the nasolacrimal drainage system. The drainage apparatus is evaluated by examining the proximal upper and lower lacrimal puncta, and the distal nasal punctum; the latter is only really practical in the horse because it is large and easy to identify on the ventrolateral floor of the nasal vestibule. The lacrimal puncta are slit‐like openings in most species, but are round in the cat. In the dog, the lacrimal puncta are 1 mm long and 0.3 mm wide and are located 2–5 mm lateral to the medial canthus, on the palpebral conjunctiva, where the line of meibomian glands ends. In the horse, the 2‐mm slit‐like lacrimal puncta are located 8–9 mm lateral to the medial canthus. The rabbit has a single lower lacrimal punctum and the pig has a single upper punctum Abnormalities in size, position, shape, and ocular discharge should be noted. The area ventral to the medial canthus should be evaluated for abnormalities (e.g., swelling) as the underlying lacrimal sac, the small dilation at the confluence of the upper and lower canaliculi, is a common site for foreign bodies. A more detailed assessment of the nasolacrimal system can be made with the application of fluorescein dye (Jones test), irrigation (see the “Nasolacrimal Flush” section), and imaging. Gross examination of the ocular surface includes assessment of the tear film and cornea. The specular reflection of the cornea (Purkinje image) appears as a focal reflection of light from a window or artificial light in the examination area. The specular reflection provides information about the optical smoothness of the ocular surface and thereby the quality and quantity of the tear film and corneal regularity. Gross examination of the limbus, cornea, anterior chamber, anterior iris, pupil, and anterior lens is performed at this stage. The cornea is slightly oval in most mammals and should appear as a transparent, smooth, convex structure. Evaluation of the resting pupil size, shape, and mobility of the pupil, and appearance of the anterior chamber structures should follow. In the horse, the insertion of the pectinate ligaments onto Descemet’s membrane (i.e., “gray line”) can be observed laterally and medially, enabling direct observation of the iridocorneal drainage angle. Direct observation of the drainage angle is also possible in the cat because of its deep anterior chamber and marked corneal curvature. The cornea is examined next. Most mammalian corneas are oval, with a greater horizontal than vertical diameter. The normal cornea is an avascular, nonpigmented, convex structure. The presence of surface irregularities, blood vessels, pigment, or other opacities indicates disease. Corneal integrity is most readily evaluated with fluorescein dye. Fluorescein dye is hydrophilic and binds readily to exposed corneal stroma if an ulcer is present. The dye will not bind to intact healthy corneal epithelium nor to Descemet’s membrane and the endothelium. Corneal sensation may be demonstrated by touching the cornea gently with a thin wisp of cotton while holding the eyelids open. When touched from the periphery to avoid a menace response, the normal corneal reflex is retraction of the globe, with protraction of the nictitating membrane. This corneal reflex tests cranial nerves V, VI, and VII. The degree of corneal sensation can be semi‐quantified in different areas of the cornea using an esthesiometer, discussed later in “Advanced Ophthalmic Diagnostics.” Slit‐lamp biomicroscopy by the veterinary ophthalmologist may be useful in characterizing the microscopic details and exact depth of corneal lesions. Additional tests for corneal disease include culture, cytology, tear tests, and use of other ophthalmic dyes, e.g., Rose Bengal. The anterior chamber is observed for transparency and depth. Anterior chamber depth shows more variation in exotic species than in domestic animals. The anterior chamber depth may decrease because of intumescent cataract, iris bombé, or a mass within or behind the iris. It may appear unusually deep in eyes without lens support in cases of aphakia or pseudophakia, reabsorbing cataract, or lens luxation. A slit beam, as may be found on many direct ophthalmoscopes and slit‐lamp biomicroscopes, may be useful in evaluating the depth of the anterior chamber. The aqueous humor of the anterior chamber should be transparent and therefore free of blood (i.e., hyphema), protein (i.e., aqueous flare), cells, fibrin, cysts, parasites, tumors, and foreign material. Aqueous flare can be detected with a slit beam. In a dark room, a slit or tiny circular beam is focused on the cornea and viewed from an angle perpendicular to the beam. As it contains only 10–50 mg/dl of protein, the normal aqueous humor is clear. With inflammation, however, this protein content can reach 5–7 g/dl, which is a condition called plasmoid aqueous. Under these conditions, both protein and cells suspended in the aqueous humor reflect the focused beam, creating the Tyndall effect. If the anterior chamber cannot be visualized because of corneal opacification, B‐scan ultrasonography may prove useful for evaluating chamber depth and consistency. Aqueous paracentesis, described under the “Advanced Ophthalmic Diagnostics” section, may provide useful information via culture, serology, or cytology. Laser cell fluorophotometry or flaremetry can measure protein and cellular debris levels in the aqueous humor and is extensively used in pharmacological testing. Because of the presence of the scleral shelf, the canine iridocorneal angle (ICA) and opening of the ciliary cleft (CC) cannot be visualized without special lenses. The direct goniolens and indirect gonioprism allow observation of the entrance into the ICA for congenital defects, abnormal narrowing, peripheral anterior synechiae, extension of iris or limbal neoplasms, and foreign bodies. Though still limited in use, ultrasonic biomicroscopy using probes from 40 to 60 MHz permits high‐resolution imaging of the aqueous outflow pathways. The iris is evaluated for color, consistency, pupillary membrane strands, pupil size and shape, and stability. Multiple colors within an iris may occur congenitally, as with heterochromia irides, or may be acquired, as with chronic inflammation or neoplasia. Blue irides occur in some lightly pigmented breeds, whereas albino animals have pink‐to‐white irides. Iris darkening or thickening (or both) may occur with chronic inflammation and neoplasia. Hypoplastic and atrophic irides are thin, and usually transilluminate; focal holes may be present as well, especially near the pupil margin. The major arterial circle of the iris is often visible (especially in iridal heterochromia) as an elevated, wavy line near the iris base. The pupil may be abnormally dilated with iris atrophy, iris coloboma, glaucoma, and retinal or optic nerve disease, or it may be abnormally small in microphthalmic eyes with congenital hypoplasia of the iris sphincter with Horner’s syndrome or with anterior uveitis. Abnormalities of pupil shape may occur with lens luxations, anterior and posterior synechiae, iris neoplasia, trauma, or iris atrophy. The pupil is round in dogs, a vertical slit in cats, and a horizontal ellipse in horses and cattle. Most ungulate species have nodular extensions of the posterior pigmented epithelium of the iris (i.e., granula iridica or corpora nigra), which protrude along the dorsal and ventral pupillary margins. In alpacas and llamas, the dorsal iris is extended in “radial folds” protruding above the pupil, similar to the granula iridica in the horse. Similar structures are not typically seen in small animals. Iris masses in small animals should be retroilluminated to determine whether they are hollow (i.e., cystic) or solid (i.e., neoplastic). Details of iris lesions may be seen through an otoscope head or, preferably, with the slit‐lamp biomicroscope. A slit beam can be used to determine whether an iris lesion is elevated or, less commonly, depressed. Further diagnostic procedures include transillumination, retroillumination, gonioscopy, ultrasonography, brush cytology, and biopsy. IOP is assessed in all patients presented for complete ophthalmic examination; the applanation or rebound tonometer is preferred by the veterinary ophthalmologist as these instruments are most accurate. IOP measurement should initially be always carried out prior to the application of mydriatics. In the absence of glaucoma and lens luxation, pupils are pharmacologically dilated. Tropicamide (1%) is preferred in most mammals because of its rapid onset (10–20 min) and relatively short duration (6–8 h in dogs and 8–12 h in horses). Complete mydriasis is essential for thorough lens evaluation, as well as for peripheral fundic examination. The lens is evaluated with both direct illumination and retroillumination for its position, size, shape, surface irregularities, and transparency. The size and shape of the lens in different species vary tremendously. A slit beam and the biomicroscope are preferred by the veterinary ophthalmologist to characterize cataracts. While directing the beam onto the lens and looking from an oblique angle, the examiner observes a cross section of the lens. Cataracts should be classified both anatomically and by stage of maturity. Nuclear sclerosis, which is an aging change that leads to a hazy, gray‐blue appearance in the center of the lens, must be distinguished from senile cataract. The lens may appear to be cataractous at a glance, but retroillumination and ophthalmoscopy demonstrate that nuclear sclerosis does not actually obstruct the passage of light or prevent visualization of the ocular fundus. Other methods of lens evaluation include both A‐ and B‐scan ultrasonography. The normal vitreous is a clear and homogeneous gel that fills the space between the posterior lens capsule and the retina. In prenatal animals, the hyaloid artery extends from the center of the optic disc to the axial posterior lens capsule. In very young animals, and especially ruminants, a persistent hyaloid artery may be visible postnatally for several weeks. Abnormalities of the anterior vitreous may be observed with direct illumination and preferably the slit‐lamp biomicroscope. The vitreous should be examined for extensions of uveal or retinal hemorrhage, neoplasia, parasites, retinal detachment, infection, and inflammation. Ultrasonic examination of the vitreous as well as vitreous paracentesis (i.e., hyalocentesis) is an additional diagnostic. Successful examination of the ocular fundus requires intimate knowledge of the normal variations within each species. Structures or areas to be evaluated include the optic nerve head (i.e., optic disc or optic papilla: shape, color, and topography), retinal vasculature (number and size), tapetal fundus in species with a tapetum (reflectivity, pigmentation, depigmentation, hemorrhage, exudates), and nontapetal fundus (pigment loss, hemorrhages, exudates). Diagnostic procedures to evaluate the ocular fundus include both direct and indirect ophthalmoscopy, slit‐lamp biomicroscopy with a condensing lens, fluorescein angiography (FA), electroretinography (ERG; i.e., flash and pattern), ultrasonography, biopsy, scanning laser tomography, and optical coherence tomography (OCT). Direct and indirect ophthalmoscopy are the primary means to visualize and evaluate the ocular fundus. The slit‐lamp biomicroscope is the most versatile and important diagnostic tool for the veterinary ophthalmologist, allowing magnified, three‐dimensional examination of the adnexa, cornea, anterior chamber, lens, and vitreous. With the help of specifically designed condensing lenses, even the ocular fundus can be inspected with the slit‐lamp biomicroscope. The first slit‐lamp biomicroscope, which combined a corneal microscope with a slit‐lamp biomicroscope that used a magnesium oxide light, was developed in 1911. Since then, numerous refinements and modifications have been made, and the slit‐lamp biomicroscope was introduced into veterinary medicine in 1953. With the introduction of the portable slit‐lamp biomicroscope in the 1960s (to accommodate bedridden and anesthetized human patients and children), veterinary ophthalmologists quickly changed from the cumbersome table units to the smaller portable scopes! The slit‐lamp biomicroscope is the combination of a binocular microscope with a highly versatile source of illumination. The microscope and light source are copivotal (i.e., they swing on the same axis), confocal (i.e., they focus on the same point), and isocentric (i.e., they center on the same plane) (Figure 4.3). The strong white focal halogen, xenon, or light‐emitting diode (LED) light can be modified by a series of diaphragms and filters that adjust the light shape (diffuse or focused beam), light intensity, and light‐beam width, length, orientation, and color (neutral density, cobalt blue, or red‐free). Returning light is focused through the objective lenses of the binocular microscope, and magnification can be adjusted from 5× to 40× (10–20× in portable units), depending on the model. Digital technology, and photographic and video adapters have also become available for some of the handheld slit‐lamp biomicroscope models. Portable slit‐lamp biomicroscopes are less expensive, and can be used in any position, and are thus are useful for laboratory, companion, and large animals. Figure 4.3 Use of a handheld slit‐lamp biomicroscope in a dog. Simplified handheld units or headpieces for the direct ophthalmoscope handle combine a bright light source that can be used with both a diffuse (approximately 3–5‐mm circle) or a slit (0.3–4 mm) setting with a magnifying lens of 6× magnification at a 25–35° fixed angle. These instruments have obvious limitations when compared to the more versatile handheld slit‐lamp biomicroscopes described earlier, but can still be useful for depth estimation of lesions in the anterior segment and lens, as well as with the detection of aqueous flare. The aim of slit‐lamp biomicroscope examination is to serially examine the eye under magnification with as many forms of illumination as possible, to identify any pathology and to create a mental, complete composite of the eye, including both detail and context. The light beam used during the slit‐lamp biomicroscopy can be diffuse or focal. Diffuse forms of lighting are useful to examine a large area for gross abnormalities and to obtain an initial overview of the adnexa, cornea, anterior chamber, and iris. However, to gain more detailed information and highlight subtle lesions, more focal forms of illumination are required. The concept of slit‐lamp biomicroscopy with a focal light is the creation of an optical section of the transparent tissues of the eye (cornea, anterior chamber, and lens). This is achieved by projecting a narrowly delineated, sharply focused, and bright slab of light onto the eye. The optical section of the structures visible to the examiner through the adjacent nonilluminated tissues can be likened to a histological section. Portable slit‐lamp biomicroscopes offer slit beam width options varying from 0.1 to 1.6 mm. With the use of a broad slit beam (>0.5 mm), transparent ocular structures such as the cornea become visible as a parallel‐piped “block of tissue.” The usually tall or long slit beam used for optical sectioning can be reduced to a minimum height or even a pinpoint (1–2 mm) to allow checking for the Tyndall effect, indicating any deviations of the composition of the normally optically clear AH.Both direct illumination and retroillumination are utilized during ocular slit‐lamp biomicroscopy. With direct illumination, the structure to be examined is illuminated by the light source itself, while in retroillumination, the structure of interest is illuminated by light reflected from a neighboring tissue. The same opacity within the clear ocular media may appear bright in direct illumination and dark on indirect illumination. For this purpose, corneal changes are mostly examined by retroillumination from the iris, while iris and lens changes are routinely examined with the help of light reflected from the fundus. Iris transillumination can be achieved when the fundus is illuminated through a small pupil only and will highlight defects in iris pigmentation or tissue. Ophthalmoscopy is a technique that requires extensive practice before it becomes a valuable and rapid diagnostic tool for the clinician. This is not only because of the actual difficulty of performing the procedure (with a moving eye), but also because of the huge variation in fundic appearance both between and within different species. The direct ophthalmoscope is the most fundamental diagnostic instrument for the ophthalmologist! As the veterinary otoscope and ophthalmoscope are often a combination set for the veterinarian, direct ophthalmoscopy is best first mastered before other types of ophthalmoscopes are attempted. Direct ophthalmoscopy is the classic form of ophthalmoscopy with which most clinicians are familiar because the direct ophthalmoscope is commonly available as part of a set with an otoscope (Box 4.2). The direct ophthalmoscope consists of a power source and a halogen or xenon coaxial optical system (Figure 4.4). Light is directed via a mirror or prism into the patient’s eye and is reflected back through a lens in the ophthalmoscope to the examiner. To focus clearly on the animal’s ocular fundus, the direct ophthalmoscope is influenced by the combined refractive power of the patient’s cornea and lens, as well as the refractive errors of the examiner. Refractive errors for most animals appear to be reasonably static with the exceptions of the nonhuman primate and the cat, in which some accommodation may occur. Most fundi are in focus at 0–2 D if the examiner is emmetropic. The resultant image is real, erect, and magnified several times depending on the species being evaluated (Table 4.1). The size of the eye being examined, and therefore the working distance between the examiner and the lesion of interest, can affect magnification. Figure 4.4 Optics of direct ophthalmoscopy. The direct ophthalmoscope allows alignment of the observer’s visual axis with a light beam, which is reflected via a mirror to illuminate the patient’s fundus. Light rays emanating from the patient’s eye form a real image on the observer’s retina. Table 4.1 Fundus magnification with direct ophthalmoscopy in animals.a a Change in millimeters with 1‐D change in focus by direct ophthalmoscopy. To perform direct ophthalmoscopy, animals must be either reasonably cooperative or adequately restrained. Most small animals can be gently restrained on the examination table by the owner or an assistant; some fractious animals or large animals require some form of sedation and/or an auriculopalpebral nerve block. Before examination, the pupils are usually dilated with a short‐acting mydriatic such as 0.5–1% tropicamide. To initiate direct ophthalmoscopy, the patient’s fundic reflex is identified approximately an arm’s length away from the patient’s eye. Ideally, the examiner’s right eye should be used to examine the patient’s right eye, and vice versa. Once the fundic reflex is identified, the examiner moves toward the patient to a point approximately 2–3 cm from the eye – the closer the examiner is to the patient’s eye, the wider the angle of view (Figure 4.5). In the dog and nonhuman primate, the patient’s eye is intermittently moving and often the desired area of the ocular fundus is only occasionally viewed. Once the fundus is visualized, ophthalmoscopy should proceed in a timely and orderly fashion. It is important for each examiner to establish an order or pattern for the fundic examination to ensure that no features are missed. The optic nerve is typically identified and examined first. Afterward, the remainder of the fundus is thoroughly examined in quadrants, making sure that the tapetal regions of the fundus, the atapetal regions of the fundus, the fundic periphery, and the retinal vasculature are closely examined. Figure 4.5 Close direct ophthalmoscopy. The examiner must be as close as possible to obtain an optimal image with direct ophthalmoscopy. To achieve this, the examiner ideally should use the right eye to examine the patient’s right eye, and vice versa. A circular dial on the direct ophthalmoscope holds a series of concave and convex lenses that can be rotated through the viewing aperture (Figure 4.6). Green or black numbers on the circular dial represent convex or converging lenses (positive), while red numbers represent concave or diverging lenses (negative). These lenses are selected so that the focus of the patient’s eye is obtained. A separate dial or switch adjusts the size, shape, and color of the light beam (Table 4.2). The direct ophthalmoscope can also be used to pick up any central opacities in the clear media anterior to the fundus by retroillumination. Once the fundic reflex is identified by the examiner looking through the ophthalmoscope at a distance (“distant direct ophthalmoscopy”), a dark spot detected against the fundus reflection indicates that an opacity is blocking the return of light to the observer. Figure 4.6 Direct ophthalmoscope head. (a) Observer’s side with viewing aperture, lens dial, quick lens switch, and diopter indicator. (b) Patient side with viewing hole and switches/dials allowing selection of beam size and shape, as well as light filters. Table 4.2 Light beam selections for direct ophthalmoscopy. Indirect ophthalmoscopy, in contrast to direct ophthalmoscopy, allows the clinician to view a larger portion of the fundus (larger field of view) at one time and to do so from a greater and safer working distance from the patient (Box 4.3 and Figure 4.7). Because much less magnification of the image is perceived with indirect ophthalmoscopy than with direct ophthalmoscopy, a better overview of the fundus can be achieved (Figure 4.8a–f). Direct and indirect methods complement each other, particularly if a lesion detected with the indirect method is then examined more closely, at greater magnification, with the direct method. The direct method is most useful when examining the optic disc and surrounding retinal vasculature. Figure 4.7 Binocular indirect ophthalmoscopy with the Keeler Vantage head‐mounted indirect ophthalmoscope in the dog. In this technique, the observer has not only stereopsis, but also one hand free to hold the patient’s head and eyelids. Figure 4.8 Comparison of direct (a and d), panoptic (b and e), and indirect ophthalmoscopy (c and f). These photographs illustrate the different appearances in terms of magnification and orientation of the canine and equine ocular fundus with each of the three methods of ophthalmoscopy. The necessary components of indirect ophthalmoscopy are a strong, focal light source and a converging lens. Indirect ophthalmoscopy has additional advantages over direct ophthalmoscopy when a binocular indirect ophthalmoscope is utilized, including stereopsis and the ability to use both hands for patient manipulation. Binocular indirect ophthalmoscopy uses a halogen, xenon, or LED light source, a mirror to direct light into the patient’s eye, a handheld converging lens to magnify the reflected image, and two prisms to split the reflected light so that it can be directed into both of the examiner’s eyes, thereby permitting stereopsis (Figure 4.9). Most binocular indirect ophthalmoscopes have interchangeable apertures and filters, as well as permanent or detachable teaching mirrors for students, clients, or others, and are also available with video cameras. Commercial indirect ophthalmoscopes cost in excess of US$1000. Monocular indirect ophthalmoscopy can also be performed using a bright, handheld light source (e.g., a transilluminator, direct ophthalmoscope or strong otoscope, fiberoptic bundle, and penlight) and a handheld lens (Figure 4.10). This method does not provide stereopsis and requires an assistant to restrain the patient and position the head, but nonetheless gives an overall view of the fundus. To perform the fundic examination following induction of mydriasis, the examiner grasps the patient’s muzzle with one hand and stabilizes the head. The other hand is used both to hold the eyelids open and to position the lens (with the strongest convex surface facing the examiner) 2–4 cm in front of the patient’s cornea. From a distance of approximately 0.50–0.75 m (at arm’s length), the examiner then directs the light into the patient’s eye and identifies the fundic reflex. Figure 4.9 The optics of binocular indirect ophthalmoscope allow the light from the indirect ophthalmoscope to illuminate the patient’s ocular fundus. The image is split with a prism into both examiner’s eyes, permitting stereopsis. The patient’s fundus image is virtual and inverted.
4
Eye Examination and Diagnostics
History
General Ocular Examination
Restraint
Eyelid Akinesia
Regional Anesthesia/Analgesia
Ophthalmic Examination in Ambient Lighting
Distance Examination
Vision Assessment and Neuro‐ophthalmic Examination
Menace Response
Pupillary Light Reflex
Dazzle Reflex
Palpebral Reflex
Corneal Reflex
Vestibulo‐ocular Reflex (Oculocephalic Reflex)
Tear Tests
Close Examination of the Adnexa and Globe
Intraocular Pressure Measurement and Pupil Dilation
Anterior Segment Examination After Pupil Dilation: Lens Examination
Posterior Segment Examination
Ophthalmic Examination Techniques
Slit‐Lamp Biomicroscopy
Direct Ophthalmoscopy
Animal
Fundus magnification
Millimeter equivalent per diopter change
Cat
19.5
0.22
Dog
17.2
0.28
Cow
10.6
0.74
Horse
7.9
1.33
Sheep
13.9
0.43
Rabbit
25.3
0.13
Small spot
Use with pinpoint pupil
Medium spot
Use with pupil in a darkened room
Large spot
Use in a pupil dilated by mydriatics
Red‐free light
To evaluate retinal vessels and the nerve fiber layer
Graticule
Grid (often of 16 squares) to estimate size of fundic lesions
Slit beam
Helps estimate fundic elevations/depressions
Blue light
Helps detect fluorescence
Indirect Ophthalmoscopy
Stay updated, free articles. Join our Telegram channel

Full access? Get Clinical Tree
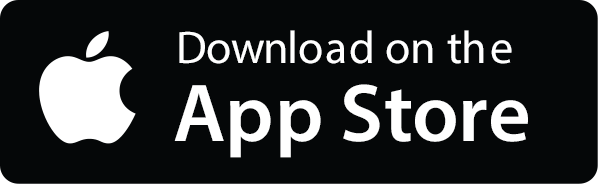
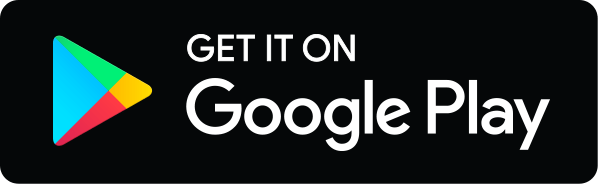