Chapter 24 The embryonic tissues which contribute to the formation of the eye include neural and surface ectoderm and neural crest‐derived mesenchyme. The primordia which initiate ocular development can first be identified as a pair of shallow grooves on either side of the folding prosencephalon (Fig 24.1). These structures can be recognised towards the end of neurulation prior to the closure of the rostral neuropore. Formation of these grooves is induced by factors from adjacent pharyngeal endoderm and mesoderm. Following closure of the rostral neuropore, the grooves form diverticula, the optic vesicles, the cavities of which are initially continuous with the cavity of the forebrain (Fig 24.2). As the optic vesicles grow laterally towards the surface ectoderm, the neuroepithelium of the optic vesicles induces the surface ectoderm to proliferate and form the lens placodes (Fig 24.3). Following formation of the lens placodes, the lateral walls of the optic vesicles begin to flatten and become concave. This results in the conversion of the optic vesicles into double‐walled optic cups. The inner and outer walls of each optic cup are initially separated by a space. The walls of the cup become apposed, resulting in the gradual disappearance of the intervening space. Narrowing of the stem of the optic vesicle leads to formation of the optic stalk. Because the invagination which shapes the optic cup occurs at the ventral margin of the vesicle, the rim of the optic cup is not continuous at its ventral aspect. This invagination results in the formation of a groove in the ventral rim of the cup which extends along the ventral surface of the optic stalk. This groove, referred to as the choroid fissure, provides access for the hyaloid blood vessels which supply the developing retina and lens (Fig 24.4). Associated with closure of the choroid fissure towards the end of the embryonic period, the hyaloid vessels become enclosed within the optic stalk (Fig 24.5) and the rim of the optic cup becomes a continuous structure enclosing a rounded space, the primordium of the pupil. With the development of the optic cup, the lens placode invaginates into the rim of the optic vesicle and forms the lens vesicle (Fig 24.6). The lens vesicle subsequently loses contact with the surface ectoderm, is surrounded by mesenchymal tissue and becomes positioned at the opening of the optic cup (Fig 24.7). Figure 24.1 Dorsal view of forebrain, A, prior to closure of the rostral neuropore showing the optic grooves, and a cross‐section, B, through the forebrain at the level of the optic grooves (X). Figure 24.2 Developing forebrain showing the optic vesicles. Figure 24.3 Cross‐section through forebrain at the level of the optic vesicles showing contact between the neuroepithelium of the optic vesicles and the surface ectoderm, A. Early stage in the formation of the optic cups and lens placodes, B. Figure 24.4 Ventro‐lateral view of the optic cup and optic stalk showing the ventral choroid fissure. Figure 24.5 Sequential stages in the closure of the choroid fissure in the optic stalk, showing the incorporation of the hyaloid vessels into the optic stalk and subsequent development of the optic nerve. Figure 24.6 Sequential stages in the development of the lens vesicle and its invagination into the optic cup. A. Initial stage in the formation of the lens vesicle. B. Invagination of the lens vesicle into the optic cup. C. Longitudinal section through the optic stalk showing the position of the developing lens, which has lost contact with the surface ectoderm, within the optic cup. Figure 24.7 Differentiation of the cells in the inner and outer walls of the optic cup. The position of the developing lens in relation to the optic cup and the early stages of eyelid formation are also shown. Initially, Pax‐6 is expressed in a region of the cranial neural ridge prior to neurulation and, for normal eye development, high and continuous levels of this transcription factor are required in cells which develop from the surface ectoderm and optic cup. In these cells, Pax‐6 up‐regulates the homeobox transcription factor Six‐3, genes encoding structural proteins such as crystallins, cell adhesion molecules and other factors which are essential for the morphogenesis of ectodermally‐derived ocular tissues. A low and transient expression of Pax‐6 is required for the differentiation of the epithelial layers of the cornea. Humans heterozygous for mutations in Pax‐6 develop defects of the iris, corneal opacity and cataracts. After retinal identity is determined in the eye primordium, the single centrally‐located eye field is converted into bilateral zones, a process which is determined by secretion of Tgf‐β, Fgf and Shh, which emanate from the underlying mesenchyme. In the absence of Shh, bilateral zones fail to develop resulting in a condition known as cyclopia, formation of a single eye in the midline cranial region. The toxic plant Veratrum californicum contains cyclopamine, an established Hedgehog (Hh) pathway inhibitor which, if ingested by ewes in early pregnancy, induces cyclopia in their lambs. A number of homeobox transcription factors, including Vax‐1, Vax‐2 and Pax‐2 further specify the fate of retinal tissue. The process of optic cup formation is regulated by interactive signals between the optic vesicle, the surrounding mesenchyme and the overlying lens placode. Although the anterior and posterior regions of the optic vesicles are similar morphologically, axial polarity of the expression of several trancription factors is evident. The expression of Brain factor 1 (BF‐1) and BF‐2 divides the optic vesicle into rostral (BF‐1) and caudal (BF‐2) expressing domains. Subsesquently, the transcription factors SOHo‐1 and Hmx1 are expressed in nested domains within the anterior retina. Fibroblast growth factors from the surface ectoderm support differentiation of the neural retina. Tgf‐β, secreted from the surrounding mesenchyme, directs formation of the outer retinal layer. These signals determine regional development of the inner and outer layers of the optic cup and up‐regulate transcription factors such as Mitf and Chx‐10 which, in turn, promote the differentiation of the pigmented and neural layers respectively. Experiments with avian embryos suggest that early differentiation of the mesenchymal cells in the cornea and associated structures depends on signals derived from the lens placode. Similar inductive influences may apply to the development of the mammalian eye. Mutations in a number of genes encoding transcription factors, including Maf, Foxe‐3 and Pitx‐e, which are expressed in the lens placode result in malformation of the lens and structures anterior to the lens. It is probable that the various transcription factors which control eye morphogenesis do so by modulating signalling molecules. These signalling molecules, which include Bmp‐4 and Tgf‐β2, are directly involved in the formation of the mesenchymally‐derived structures in the anterior region of the eye. Bmp‐4 is expressed in the iris, the ciliary body and retinal pigmented epithelium of both the embryonic and postnatal murine eye. Mice heterozygous for a null allele of Bmp‐4 show a variety of abnormalities in the iris, cornea and anterior chamber of the eye. Tgf‐β2 is expressed during both prenatal and postnatal ocular development in the anterior portion of the eye. In homozygous Tgf‐β2 knockout mice, the cornea is thinner than normal, the corneal endothelium is completely absent and the lens and iris are in direct contact with the corneal stroma. The retina is derived from the apposed walls of the optic cup. The outer wall of the optic cup forms the pigmented layer of the retina (Fig 24.7). It also contributes to the formation of the ciliary body and iris. Differentiation of the inner wall of the retina involves a series of developmental changes. Two distinct areas of differentiation develop in the inner layer of the retina. A narrow region close to the rim of the optic cup, the non‐neural area of the retina, remains thin walled and subsequently contributes to the formation of the ciliary body and iris. The remainder, referred to as the neural area of the retina, develops in a manner analogous to the stages of neural tube differentiation. The neuroepithelial cells of the inner wall of the cup proliferate and differentiate giving rise to the specialised layers of the neural retina which include the light‐sensitive rods and cones, the bipolar and ganglion cells and the supportive glial cells (Fig 24.8). As the optic vesicle invaginates and forms the optic cup, its light‐sensitive photoreceptors are positioned adjacent to the pigmented retinal layer. The cellular layers constituting the inner wall of the optic cup are designated according to their positions relative to the outer wall. Accordingly, the cells of the inner wall which are immediately adjacent to the pigmented retinal layer are called the outer visual layer of the retina and the retinal layer most distant from the pigmented layer is referred to as the inner layer. Thus, light passes through the inner layers of the retina before reaching the visual receptors. The impulses generated in the rods and cones are relayed to the overlying bipolar neurons and thence to the ganglion cells. The axons of the ganglion cells enter the optic stalk and signals are relayed via the optic nerve to the visual cortex of the brain. With the proliferation of nerve fibres, the structure of the optic stalk changes. The axons of the ganglion cells infiltrate the optic stalk and form the optic nerve which surrounds the remnants of the hyaloid artery within the optic stalk, termed the central artery of the retina. Prior to entering the brain, the optic nerves from each side meet and form an X‐shaped structure, the optic chiasma. At the optic chiasma, some fibres from each optic nerve cross to the opposite side and accompany the fibres from that side to the brain. Some optic nerve fibres terminate in the rostral colliculus while others synapse with neurons in the lateral geniculate body of the thalamus. From the geniculate body, information is conveyed to the visual cortex. Figure 24.8 Cross section through retina and associated structures showing cellular layers.
Eye and ear
Eye
Molecular aspects of development of the eye
Differentiation of the optic cup
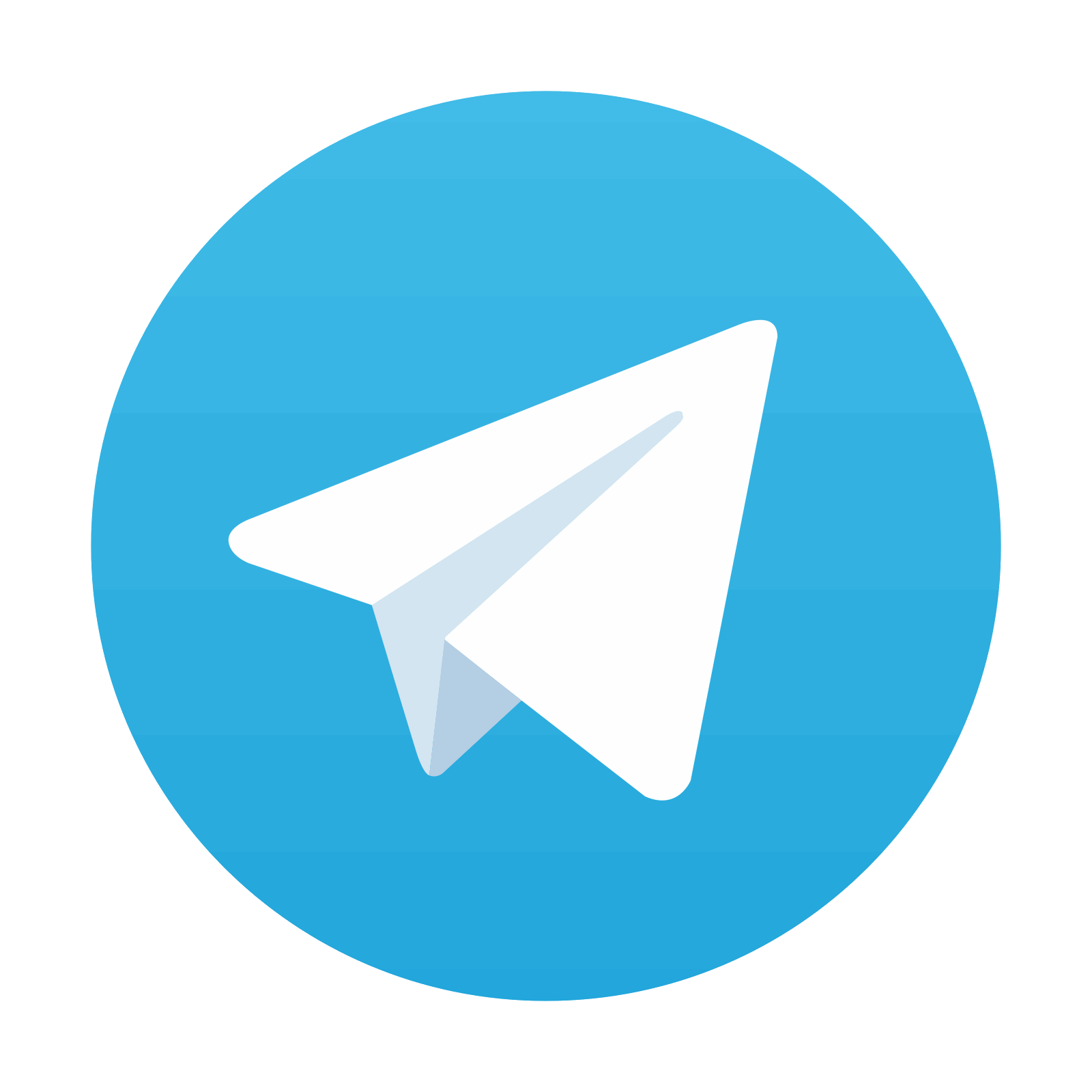
Stay updated, free articles. Join our Telegram channel

Full access? Get Clinical Tree
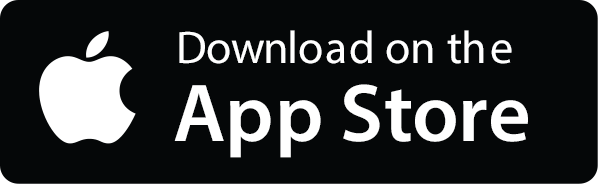
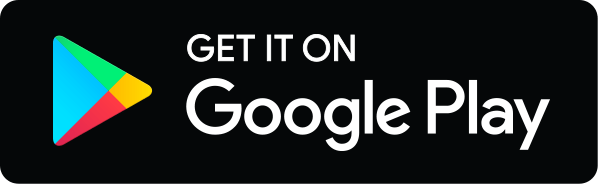