Fig. 13.1
Aneurysm elicitation in female Sprague Dawley rats by (1) Ligation of the bilateral posterior renal arteries for the induction of hypertension (according to the Hashimoto model) and (2) Ligation of the right common carotid artery for the induction of hemodynamic changes. The drinking water contained 1 % NaCl (saline). One month later the rats were subjected to bilateral oophorectomy (OVX). Aneurysm formation was assessed on vascular corrosion casts
In normotensive humans, factors such as lifting heavy objects, bending, and stress may result in a transient, recurrent blood pressure elevation that may injure arterial walls and result in the formation, enlargement, and even rupture of aneurysms. In vitro experiments of Austin et al. [6] showed that a high pulse rate and/or a sudden increase in the blood pressure can increase the turbulence of flow within saccular aneurysms. This pressure-jump phenomenon tends to result in sudden aneurysmal enlargement, bleb formation, or the rupture of saccular aneurysms. However, the reproducibility of their model was low (20–30 %) and, similar to humans, approximately 3 months were required for the development of aneurysms. In our experiments we chose to use this type of aneurysm model.
13.3.2 Hashimoto’s Model Plus Oophorectomy (Female Dominance in the Formation of CAs)
All experimental procedures and protocols were in accordance with the Japanese standards for the care and use of laboratory animals and were approved by the Care Committee of the University of Tokushima. Before any procedures, the rats were anesthetized by isofluorane (2–4 %) inhalation. Based on Hashimoto’s model [4], we ligated the unilateral posterior renal artery to induce hypertension and the right common carotid artery to induce hemodynamic stress in 7-week-old female Sprague-Dawley rats. On the following day we ligated the contralateral posterior renal artery. The drinking water contained 1 % NaCl (Fig. 13.1).
We determined the blood pressure with the tail-cuff method and confirmed that it was significantly elevated 1 month after ligation of the bilateral posterior renal arteries. Then the animals underwent bilateral oophorectomy (OVX) to reduce their estrogen level. Two months later we also confirmed the elevation of blood pressure (Fig. 13.2). Then we sacrificed the rats to assess the formation of cerebral aneurysms on vascular corrosion casts and to confirm the decrease in the estrogen level of HT/OVX+ rats (Fig. 13.2). Endothelial cells and the morphology of the inner lumen of the aneurysms were examined under a scanning electron microscope (SEM).
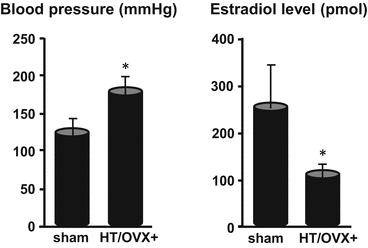
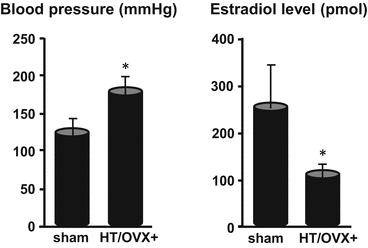
Fig. 13.2
Blood pressure and estradiol level in HT/OVX+ rats with experimentally produced renal hypertension and hemodynamic changes. * p<0.05 by the Student t-test
13.4 Assessment of the Cerebral Aneurysms
13.4.1 Vascular Corrosion Cast
Anesthetized rats were subjected to laparotomy/thoracotomy. The tip of a plastic cannula (19 G × 1¼″) inserted into the left ventricle was secured in the ascending aorta. After cutting the right atrium for drainage, the rats were perfused with 100 ml of heparinized (20 U/ml) phosphate-buffered saline using a perfusion pump (Fig. 13.3). The rate was 10 ml/min. We immediately prepared 10 ml of Batson’s No. 17 plastic resin (Polysciences, Inc., PA, USA) in a 20-ml syringe according to the protocol provided by the manufacturer. Then we manually injected 10 ml of Batson’s No. 17 plastic resin into the ascending aorta through the plastic cannula. After 24-h polymerization at room temperature the whole brain was removed and digested in 20 % KOH for 24–72 h to remove tissues surrounding vessels.
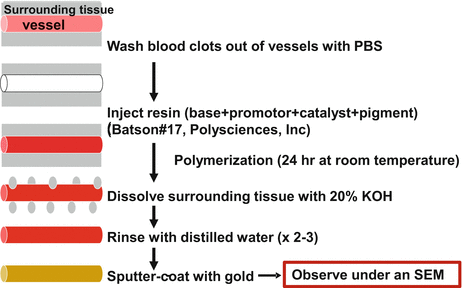
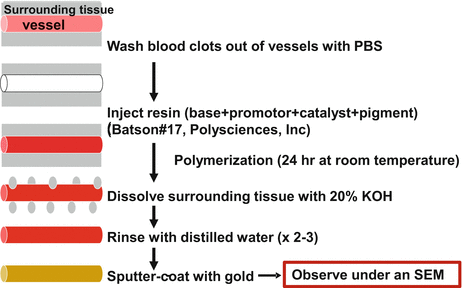
Fig. 13.3
Procedures for preparing vascular corrosion casts
Vascular casting is a powerful tool for studying arterial vascular systems; it avoids shrinkage artifacts and maintains a three-dimensional geometry. Even under a conventional microscope we saw that the blood flow on the side opposite the carotid artery ligation was increased (Fig. 13.4). After 2–3 water rinses the vascular cast was dried in a desiccator containing silica gel and sputtered with gold.
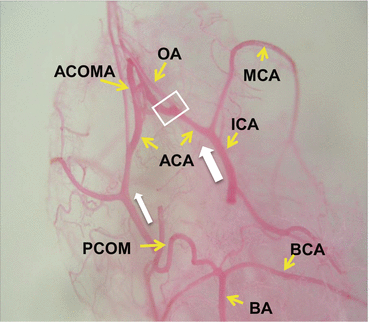
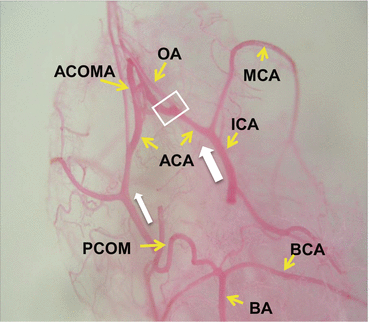
Fig. 13.4
Hemodynamic changes. The blood flow (white arrow) on the side opposite the carotid artery ligation (left side) was increased
The remaining vascular cast was mounted on an SEM stub using colloidal silver paste, sputter-coated with gold, and screened for the presence of aneurysmal changes under a Keyence VE-8800 (Osaka, Japan) SEM. The beam voltage was 3KV and the arterial bifurcations of major arteries were carefully examined. The corrosion cast sample of major arteries shown in Fig. 13.5 mirrors the intraluminal imprint seen under the SEM. Imprints of endothelial cell nuclei and endothelial cell borders are clearly visualized.
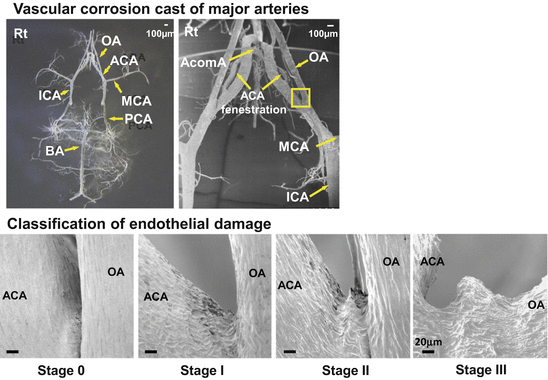
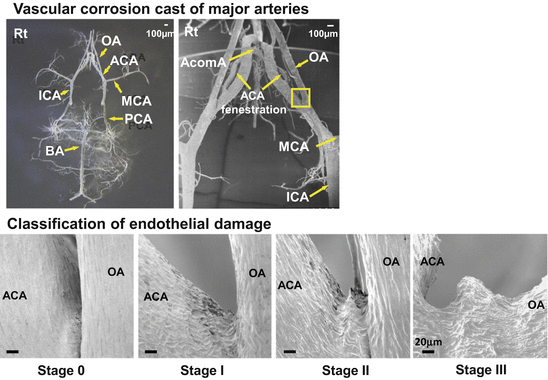
Fig. 13.5
Vascular corrosion casts of major arteries and classification of endothelial damage. Stage 0 = normal. Stage 1 = endothelial damage. Stage 2 = elevation of the intimal pad. Stage 3 = saccular aneurysm
As many aneurysms formed at the bifurcation of the left anterior cerebral artery (ACA) and the olfactory artery (OA), we focused on this area (ACA-OA bifurcation) (Fig. 13.5). In normal rats endothelial cells are arranged and oriented in the direction of flow (Stage 0). We classified anomalous endothelial cell imprints into 3 stages. Stage I indicated that the imprints were irregular, Stage II that there was a slight elevation of the intimal pad apparently affecting the apex of the bifurcation rather than the apical intimal pad, the site of earlier changes. We posit that intra-aneurysmal pressure and blood flow patterns control the expansion of aneurysmal dilation toward the bifurcation apex. The aneurysmal dome exhibited irregularly-shaped endothelial imprints and there were areas devoid of endothelial cell markings (Stage III). The absence of endothelial cell imprints may be more pronounced in larger aneurysms and may be related to their increased incidence of rupture. We were able to differentiate early aneurysmal dilation (Stage II) from junctional dilation, considered by some as a pre-aneurysmal lesion. Junctional dilation was present in some rats and differed from aneurysmal dilation in that the area of dilation was covered by normal endothelium. Some of the junctional dilations were on the right-, i.e., the low shear-stress side. Based on these observations we suggest that true junctional dilations do not progress to Stage III (saccular aneurysms) without undergoing the changes described as Stage I–II.
Earlier studies to investigate the pathology of CAs in clinical and experimental settings relied on the appearance of arterial wall dilation, identified by either angiography or dissecting microscopy, for the diagnosis of aneurysmal changes [7]. Our technique yields material to study the ultrastructural and 3D morphology of large areas of the vascular tree.
Under SEM images, we found that the incidence of cerebral aneurysm formation was 3 times higher in hypertensive HT/OVX+ than HT/OVX− rats (60 vs. 20 %). None of the control sham-operated rats developed aneurysms. Our aneurysm model was reliable and reproducible.
13.4.2 Immunohistochemical Findings
13.4.2.1 The Expression of Angiotensin II and the NADPH Oxidase Subunit
Compared to sham-operated rats, in HT/OVX+ rats there was obvious outward bulging of the arterial wall with disappearance of the internal elastic lamina. Note the strong immunoreactivity for angiotensin II (Ang II) antigen in the cerebral aneurysm (Fig. 13.6). In the cerebral aneurysmal wall and the parent artery adjacent to the lesion, there was immunoreactivity to a NADPH oxidase subunit, NOX4. Another subunit, p22phox was observed in the vascular intima and media of the cerebral aneurysm. These findings suggest that the renin-angiotensin system (RAS) was activated in association with hypertension and oxidative stress was increased in the aneurysmal wall (Fig. 13.6).
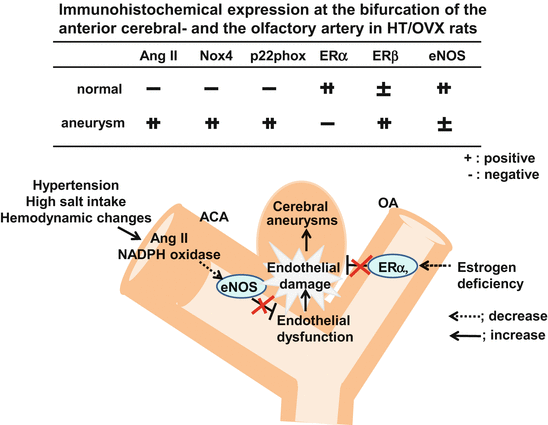
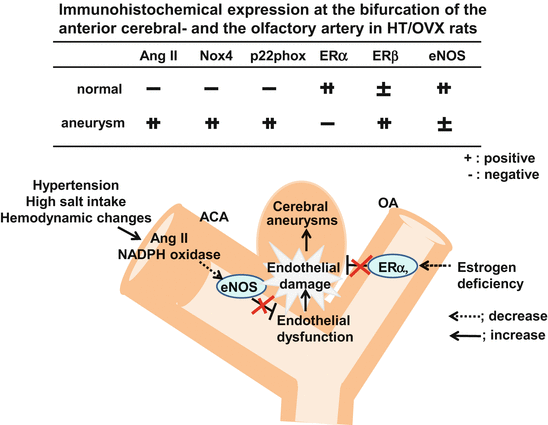
Fig. 13.6
Increased expression of angiotensin II (Ang II) and NADPH oxidase subunits (Nox4 and p22phox), decreased ERα and eNOS at the bifurcation of the anterior cerebral- and the olfactory artery in aneurysm model rats subjected to hypertension (HT), hemodynamic changes and oophorectomy (OVX + )
13.4.2.2 The Expression of Estrogen Receptors (ERs)
Estrogen predominantly binds to ERα and exerts vasoprotective effects. Although ERβ has a low affinity for estrogen, like ERα it plays a vasoprotective role. In the aneurysmal wall of our HT/OVX+ rats the expression of ERα was lower and the expression of ERβ was higher than those in sham-operated rats (Fig. 13.6), suggesting that under estrogen deficiency conditions ERβ may play a compensatory role.
13.4.3 Analysis of the mRNA Level in the Aneurysmal Wall
Each intracranial artery in HT-, OVX+-, and HT/OVX+ rats was isolated and total RNA was extracted. Transcribed cDNA was subjected to quantitative real-time polymerase chain reaction assay (qRT-PCR). The mRNA level of eNOS was normalized with GAPDH mRNA as the internal standard. In HT-, OVX+-, and HT/OVX+ rats, the mRNA level of eNOS was significantly reduced [8]. This reduction was more marked in HT- than OVX+ rats, suggesting a predominant vascular dysfunction due to hypertension. We confirmed this phenomenon using endothelial cells under the presence of angiotensin II and/or without 17-β estradiol in the in vitro study [8].
Taken together, these findings suggest that hypertension with hemodynamic change activates renin-angiotensin system (RAS), leading to the downregulation of eNOS and endothelial damage. On the other hand, estrogen deficiency decreases the expression of ERα, thereby decreasing antioxidative effects and increasing endothelial damage. These combined actions may facilitate the formation of CA (Fig. 13.6).
Furthermore, the upregulation of the mRNA level of inflammatory-related molecules including p-selectin, ICAM-1, VCAM-1, TNFα, and of RAS [9, 10] and the greater degree of extracellular matrix degradation in the aneurysmal wall of HT/OVX+ rats are suggestive of a relationship between CAs and vascular degradation due to inflammation.
13.5 The Acquired Degenerative Changes
The apical intimal pad of arterial bifurcations where aneurysms arose is the site of greatest hemodynamic stress which is transmitted along the arterial wall. The incidence of aneurysms increases at sites where the cerebral arteries may be exposed to greater wear and tear as evidenced by anomalies in the circle of Willis, e.g., arterial fenestration, ICA agenesis, persistence of a primitive artery.
In our rat model, the formation of cerebral aneurysms is associated with endothelial damage and vascular degradation due to inflammatory-related molecules and is increased at ACA-OA bifurcation where the cerebral arteries may be exposed to greater wear and tear. Under the hypothesis that the opening of tight junctions due to the loss of the tight junction proteins occludin and zona occludens-1 (ZO-1) in damaged endothelium allows macrophage migration and leads to cerebral aneurysm formation, we examined tight junction proteins in our aneurysm model HT/OVX+ rats. We also examined the morphology of the vascular wall in the aneurysms and performed the immunohistochemical and qRT-PCR studies. In the very early stage before aneurysm formation, the expression of occludin and ZO-1 was reduced in injured endothelial cell junctions exhibiting gaps [10]. In the course of aneurysmal progression, the reduction in these proteins was correlated with macrophage migration. Furthermore, in HT/OVX+ rats we observed an increase in the degradation molecules matrix metalloproteinase-9, nicotinamide-adenine dinucleotide phosphate (NADPH) oxidase, and monocyte chemoattractant protein-1 [10]. At sites prone to the formation of aneurysms the reduction in tight junction proteins was associated with the expression of degradation molecules and the migration of macrophages. These observations suggest that the destruction of tight junctions facilitates macrophage migration, thereby accelerating the formation of cerebral aneurysms in rats (Fig. 13.7).