6 Evaluation of Semen in the Andrology Laboratory Steven P. Lorton* Bartlett (1978) formulated success in artificial breeding using an ‘Equation of Reproduction’. According to this formula, success as measured by the percentage of animals born is dependent upon four factors: A, % herd detected in oestrus and inseminated; B, % fertility of the herd; C, % fertility of semen; and D, % efficiency of the inseminator/technician. Therefore: % born = A × B × C × D Because the end result is the cumulative product of the factors, and not their arithmetic average, it is extremely important that each factor remains as high as biologically and practically possible in order to achieve optimal results. In artificial insemination centres, veterinary clinics and/or in semen collection centres, the quality and quantity of the semen collected must be assessed in order to achieve the optimal level of factor C in Bartlett’s Equation of Reproduction. This chapter will discuss the techniques that are currently used to achieve this goal. The significance of these techniques in predicting ‘fertility’ will also be discussed. Ejaculates vary significantly in volume among species. Table 6.1 lists typical volume characteristics for several species. As a result of this variation in volume, during collection and subsequent evaluation of ejaculates, suitable devices are used to estimate ejaculate volume. For very small volumes, e.g. for the ram, specialized collection tubes are available (Fig. 6.1). Centrifuge tubes of 15 ml capacity are often used for bull and dog ejaculates, while collection bottles or insulated cups are typically used for the higher volume stallion and boar ejaculates. In many cases, these collection devices have volumetric calibrations, although manufacturers do not often provide accuracy data for these calibrations. Many laboratories use a standard laboratory scale to measure the weight of the ejaculate, after making an allowance for the weight of the collection tube or bottle. Volume is equated with the weight in grams. Kaproth (1988) reported a variation to this gravimetric procedure that employed a regression formula to correct for an increase in specific gravity with concentration. Table 6.1. Ejaculate characteristics. Adapted from Setchell, 1991.
Reproduction Resources, Walworth, Wisconsin, USA
Introduction
Measurement of Ejaculate Volume
Species | Ejaculate volume (ml) | Sperm concentration (× 10-6/ml) |
Bull | 2–10 | 300–2000 |
Boar | 150–200 | 25–350 |
Dog | 2–15 | 60–300 |
Ram | 0.5–2 | 2000–5000 |
Stallion | 20–300 | 30–800 |
Measurement of Spermatozoal Concentration
Measurement of the concentration of spermatozoa in ejaculates is a fundamental and extremely important step in the evaluation and then in the processing of ejaculates for artificial insemination.
The simplest and most inaccurate method to estimate spermatozoal concentration is by means of visual inspection. This conservative processing technique is used effectively when, for example, a minimum number of insemination doses must be produced and when semen availability is not limited. In the case of liquid boar semen, for example, a fresh ejaculate that has the appearance similar to whole milk, i.e. creamy white, might be extended to yield 6–8 insemination doses; this would compare with 20–24 doses if actual concentration measurements were performed. In contrast, a semiclear, non-fat or skimmed milk-like appearance of an ejaculate might be extended to yield only 2–3 doses.
The Karras Spermiodensimeter has been used for the determination of sperm concentration in boar and bull ejaculates (see Fig. 6.2). In this case, the measurement is based upon the turbidity of a sperm suspension prepared in normal saline. The concentration is visually estimated by interpretation of the instrument’s scale through the turbid solution. Clarity of the scale is an indirect indication of spermatozoal concentration. Vianna et al. (2007) compared data obtained using this densimeter with data obtained using the Neubauer haemocytometer. These authors noted that data obtained using the densimeter was consistently an overestimation, which was due especially to the variability in volume of seminal fluids. The authors developed a corrected concentration table for use with the densimeter to provide data more consistent with those obtained with the haemocytometer.
The haemocytometer counting chamber has classically been the ‘gold standard’ for sperm concentration evaluation. Although variations exist depending upon the manufacturer, this chamber usually consists of a glass slide with two etched grids of precise dimensions. A special coverslip is applied on to support ‘rails’; thus creating a chamber of a specific volume over each grid. After filling each chamber with a diluted neat or extended semen sample, the user observes the chamber using a standard microscope and manually counts the sperm within the limits of the grid. (See detailed instructions below.)
Various manufacturers and types of counting chambers are available, which include the Petroff-Hauser, the Improved Neubauer and the Makler® chamber (see Fig. 6.3); however, the Improved Neubauer Haemocytometer is used widely in animal andrology laboratories. Several authors (Belding, 1934; Salisbury et al., 1943; Bane, 1952; Freund and Carol, 1964; Lorton et al., 1984; Seaman et al., 1996; Brazil et al., 2004; Christensen et al., 2005; Payne and Clark, 2005) have described causes of variation of the data obtained with counting chambers. The variation can be attributed to: type of chamber, technician, number of dilutions and the number of chambers counted. Therefore, it is recommended that a minimum of four counts, i.e. both sides of two chambers, are made for each diluted subsample. Additional dilutions, chambers and technicians will reduce variation. Counts of the two sides of each chamber should be within 10% of each other. A standardized method of counting should also be used. The evaluation of samples using haemocytometers is timeconsuming – approximately 15–20 min/chamber.
Fig. 6.2. The Karras densimeter. Photo courtesy of Minitüb GmbH Tiefenbach, Germany.
Suggested method for the determination of the concentration of spermatozoa in semen using an Improved Neubauer Haemocytometer
1. Prepare suitable diluted subsamples of the semen. The dilution used is somewhat dependent upon the species being studied. For example, for rams or bulls, ejaculates are typically very concentrated, so a 1:201 dilution could be used. Boar ejaculates are less concentrated, so a 1:101 dilution could be used.
Notes:
(i) It is important that dilutions are properly prepared. The outside surface of sample pipette tips should be carefully wiped to remove adherent cells. Tips should be wiped from the top towards the open end. The open end of the tip should not be touched, as this will result in sample loss by wicking action from inside the tip.
(ii) It is important that dilutions are properly prepared. For example, 0.01 µl (10 µl) sample plus 1.0 µl diluent results in a 1:101 dilution; 0.01ml (10 µl) sample plus 2.0 µl diluent results in a 1:201 dilution.
(iii) For accurate counts, a diluent that results in immotile spermatozoa should be used, e.g. 10% formaldehyde in saline (10 µl of 40% formaldehyde in saline).
(iv) Prepare a dilution that results in a count of approximately 150 spermatozoa in 5 large squares of the counting chamber.
2. Properly position the haemocytometer coverslip on the haemocytometer. After thoroughly mixing the diluted subsample, a drop of semen is placed on the filling ‘V’ groove (if present) of the haemocytometer – or it is placed at the intersection of the cover slip and top of the haemocytometer. The liquid should be drawn up to just cover/fill completely the one ‘side’ of the chamber. This procedure is repeated for the other side of the haemocytometer.
Note: Overfilling or underfilling of the chamber will result in inaccuracy of concentration measurements.
3. Wait approximately 5–10 min for the sperm to settle to the bottom of the haemocytometer. Do not allow the haemocytometer to dry out before counting. In order to be sure that the haemocytometer does not dry out, the following procedure can be followed. Place a paper towel large enough to hold the haemocytometer in the bottom of a dish and wet the towel. Then place two microscope slides on the wet paper towel as a base, positioned so that the haemocytometer can be placed on the slides above the wet towel. The slides prevent the bottom of the haemocytometer from getting wet, and avoid any need to manually dry the chamber, as doing so may dislodge the haemocytometer coverslip. Cover the dish and allow the necessary time for the cells to settle.
4. The haemocytometer consists of a grid system. Five large squares should be counted – either each of the corners and the centre square, or from corner to corner diagonally (see Figs 6.4 and 6.5).
Counting suggestions:
(i) Count the sperm heads only, ignore the sperm tails.
(ii) Establish a pattern to use every time.
(iii) Count the sperm heads touching two of the sides of the large squares, e.g. left and bottom. Do not count the sperm heads touching the remaining two sides, e.g. right and top.
(iv) Total counts for the two sides of each individual chamber should be within 10% of each other. If not, these counts should be discarded and another chamber prepared and counted.
5. Add the counts from all five squares of one side of the haemocytometer as one total count, and then count the other side. It is recommended to count at a minimum four sides, using two haemocytometers for each semen sample under evaluation.
6. Calculate the total number and concentration of spermatozoa as follows (steps A–D):
A. Average the haemocytometer counts.
B. Multiply the average of the counts by the dilution factor.
C. Multiply the result of step ‘B’ by 50,000 (5 × 104). This result is the number of cells/ml ejaculate. (Note: the factor 50,000 is used only if five large squares of the chamber are counted.)
D. Multiply the result of step ‘C’ by the volume of the ejaculate. This result is the total number of sperm in the ejaculate.
Example:
Ejaculate volume = 10 µl
Total counts in each of the five large squares are: 161, 168, 164, 167 after a dilution of 101 (counts from two chambers, two sides each).
A. (161 + 168 + 164 + 167) ÷ 4 = 660 ÷ 4 = 165
B. 165 × 101 = 16665 = 16.7 × 103
C. (16.7 × 103) × 50,000 = (16.7 × 103) × (5 × 104) = 8.4 × 108 = no. cells/ml
D. 8.4 × 108 × 10 µl = 8.4 × 109 = 8.4 billion sperm total in the ejaculate.
7. Clean the haemocytometer and coverslip carefully.
Note: These are coverslips unique to the haemocytometer and cannot be replaced with an ‘ordinary’ microscope coverslip.
Improved Neubauer Haemocytometer maths
• Counting chamber depth: 0.100 mm.
• Ruling pattern: 1/400 square mm.
• Rulings cover 9 mm2 (9 squares of 1 × 1mm).
• The centre square is subdivided into 25 large squares separated by triple lines.
• Each large square is subdivided into 16 small squares of 1/400 mm2.
• The chamber height is 1/10mm, so the volume over each small square is 1/4000 mm3 or 0.00025 mm3.
• If ‘N’ is the number of cells counted in five large (80 small) squares:
• Then: N = no. of cells in 80/4000 mm3.
• So in 1 mm3, there are 4000 (N)/80 cells.
• Therefore, in 1 µl, there are 4,000,000 (N)/80 cells.
• If ‘D’ is the dilution factor, then the number of cells/ml is: 4,000,000 (N) (D)/80 or (5 × 104) (N) (D).
Lorton (1986) described a technique for evaluating initially extended semen using a spectrophotometer. Photometric estimations of sperm concentration typically are simple and relatively quick (30–60 s). Photometers/spectrophotometers must be calibrated, i.e. to give OD versus sperm concentration, when purchased, as well as by periodic checks and after changing the light source. Calibrations change over time, especially in machines equipped with incandescent light sources, although they vary to a lesser degree in machines with light emitting diodes (LED) as the light source. Foote et al. (1978) reviewed calibration techniques when using haemocytometer counts. Calibrations must be made for each individual species of sperm under study. The NucleoCounter® SP-100™ (see below) provides more repeatable and more accurate data than haemocytometers and can be used for calibrations instead. The electronic function of photometers/spectrophotometers should be evaluated frequently (daily, weekly) with turbidity standards and/or filters.
Evaluation of concentrations can be made using the flow cytometer (Evenson et al., 1993). Within these machines, individual cells pass in a liquid fluid stream through a laser and are electronically counted. ‘Gates’ based upon cell size can be established in order to reduce error from debris and cells other than sperm (COTS), and extremely accurate data can be obtained. However, flow cytometers are expensive and relatively difficult to maintain and operate. Few semen production laboratories utilize them for concentration measurements alone, though they are used for more complex morphological and functional analyses (see below).
The NucleoCounter® SP-100™ (ChemoMetec A/S, Allerod, Denmark) is a relatively new device for the measurement of sperm concentration in raw (neat) ejaculates and/or extended semen. After a simple dilution with a reagent that results in disintegration of the sperm cell membranes, the sample (50 µl) is loaded into a special cassette (Fig. 6.6), which is manufactured with the fluorescent staining agent propidium iodide (PI) immobilized within it. Due to the absence/disruption of the cell membranes, the PI can be incorporated into the spermatozoa and selectively binds to the DNA of each cell. The microscope of the SP-100™ utilizes a green light source that causes the PI-DNA complexes to fluoresce red (Fig. 6.7), thus allowing the machine’s processing unit to count each complex. Algorithms eliminate COTS, invalid data due to dilution error and/or cassette loading error.
Due to the specificity of the PI-DNA binding and fluorescence, extender, seminal gel, COTS and other debris do not interfere with data analyses. Hansen et al. (2006) compared the use of the SP-100™ with the haemocytometer, the spectrophotometer, computer assisted semen analysis (CASA) machines and the flow cytometer (FACS). Repeatability for the SP-100™ and the FACS were similar, at 3.1% and 2.7%, respectively, while it was 7.1%, 10.4%, 8.1% and 5.4% for the haemocytometer, the Corning 254 photometer, and two models of CASA machines. Correlations between the FACS and the SP-100™ were highest (as was the repeatability of these instruments). Figure 6.8 presents examples of regressions of SP-100™ data versus flow cytometer data. Further validations of the SP-100™ instrument for measurement of sperm concentration were done by Comerford et al. (2008) and by Comerford (2009).
Anzar et al. (2009) concluded that the Nucleocounter® SP-100™ and the flow cytometer could be used with equal confidence for the measurement of sperm concentration. These authors also provided evidence that 13% additional bull artificial insemination doses could be produced when using the SP-100™ rather than haemocytometers for the calibration of spectrophotometers. The SP-100™ has become the instrument of choice for evaluating sperm concentration in neat and extended semen from several species, including stallions (Comerford et al., 2008; Morrell et al., 2010), bulls (DeJarnette and Lefevre, 2008; S. Lorton, unpublished), pigs, dogs, sheep, deer, wolves (S. Lorton, unpublished) and trout (Nynca and Ciereszko, 2009). Owing to the accuracy and repeatability of the Nucleocounter® SP-100™, it should be considered as the ‘new gold standard’ for the measurement of sperm concentration.
A later study (S.P. Lorton and M.M. Pace, unpublished) involving approximately 20,000 first inseminations also verified the model and provided additional evidence that the asymptotic value for individual bulls differed significantly. However, it was shown that, across bulls, approximately 10–12 × 106 motile spermatozoa per insemination dose resulted in the asymptotic level of fertility (non-return rate). Den Daas et al. (1998) provided similar results and also discussed the variability between bulls. Foote and Kaproth (1997) presented evidence that the total sperm number per artificial insemination dose could be reduced to 10 × 106 with only a one percentage point reduction in non-return rate. Further, Chenoweth et al. (2010) described data showing a strong correlation between the number of progressively motile spermatozoa per insemination dose (1.5, 3.5 and 7.0 × 106) and palpated pregnancy rate. A specialized process developed in New Zealand using Caprogen extender and sperm storage at ambient (15–23°C) temperatures results in optimal fertility at levels with approximately 1.5 × 106 total (1.0–1.2 × 106 motile) spermatozoa per insemination (Shannon et al., 1984). The number of motile spermatozoa inseminated is, therefore, a compensable semen trait. (See Saacke et al., 1991 for a review of compensable and noncompensable semen traits.)
There have been similar, but less extensive, sperm number titration studies in the pig. Baker et al. (1968) demonstrated an interaction between the number of sperm inseminated and the volume inseminated. By examining the number of spermatozoa recovered from oviducts of gilts and the number of spermatozoa bound to the zonae pellucidae of ova, these authors concluded that 5–10 × 109 total spermatozoa in a volume of approximately 100 µl resulted in optimum fertility. Johnson et al. (1981, 1982) demonstrated significant differences among boars in farrowing rate after insemination with 3 × 109 total sperm. In contrast, Xu et al. (1998) were unable to demonstrate a difference in farrowing rate, while there was a significant difference in total live-born, when the insemination dose was reduced from 3 × 109 to 2 × 109 total sperm. Flowers (2002) reported a linear relationship of litter size among some boars (32 of 200 boars) versus an asymptotic relationship among most boars (133 of 200 boars) when the number of sperm inseminated was increased from 1 × 109 to 9 × 109. The authors commented that if additional motile spermatozoa were inseminated, a plateau effect may also have been detected for the animals showing the linear relationship. In contrast, Alm et al. (2006) reported a significant decrease in 60-day non-return rate and litter size when the insemination dose decreased from 3 × 109 to 2 × 109 total sperm. Reicks et al. (2008) reported a decrease in litter size when sperm number inseminated was reduced from 4 × 109 to 2.5 × 109 total sperm, while farrowing rate did not differ. These studies were all performed using ‘conventional’ cervical artificial insemination (AI) with chilled semen.
More recently, post-cervical and deep uterine surgical insemination studies in pigs have demonstrated that both insemination volume and number of sperm can be reduced without a reduction in pregnancy rate or litter size, e.g. from 3–3.5 × 109 total sperm in 75–80 µl to approximately 1–1.5 × 109 total sperm in a volume of 35–40 µl (post-cervical insemination), or even 0.5 µl (deep uterine insemination, using fresh or frozen–thawed semen) (Krueger and Rath, 2000; Rozeboom et al., 2004; Wongtawan et al., 2006; Vazquez et al., 2008 (review); Sonderman et al., 2011).
Evaluation of Spermatozoal Morphology
Our brains, via the eyes, visualize objects and images using colours and/or variations of the grey scale to establish contrast. Using the microscope, we can visualize extremely small objects, many of which have no distinguishable colour or, therefore, contrast. For example, when viewing spermatozoa with the routine bright microscope, the cells appear white with a white/grey background. A wide variety of biological stains and probes are often used to increase the contrast (see Table 6.2), or to identify particular regions or cell structures, often using fluorescent techniques. Many of these stains or probes have recently been used with the flow cytometer to evaluate sperm morphology, cell structures and/or membrane integrity (see Plates 4 and 5). Use of the flow cytometer has the distinct advantage of quickly analysing thousands of cells, compared with only 100–200 cells with the microscope.
The optics of a phase microscope modify the light waves hitting and surrounding the viewed objects, e.g. spermatozoa, to create additional specimen contrast, without the use of biological stains. The result is increased ability by the user to see the objects and differentiate detail. (See Abramowitz (1987) for a detailed discussion of the physics of phase contrast microscopy.) The optics of the phase microscope must be properly set up (aligned) in order to establish the phase image. A description is given below of the suggested method for aligning a phase microscope. Note that this procedure will vary slightly by microscope manufacturer and model.
Assembly, set-up and use of the phase microscope system
The primary components of the phase optical system are the phase turret condenser, the phase objective lenses and the centring telescope (see Fig. 6.9). Note that some microscope manufacturers incorporate the centring device into the body of the microscope and therefore eliminate the use of a separate centring telescope.
Assembly and set up procedure
1. Rotate the coarse focusing knob to move the stage platform to its lowest position.
2. Remove the objective dust caps from the underside of the revolving nosepiece.
3. Screw individual objective lenses into the nosepiece. For convenience, mount the objectives in magnification order i.e. 10×, 20×, 40×, 100×.
Table 6.2. Biological stains and probes used to evaluate spermatozoa (see also Appendix at end of this chapter).
Function | Stain/probe | Reference |
General morphology | Alkaline methyl-violet | Hackett and Macpherson, 1965 |
| Anti-ubiquitin–FITC (fluorescein isothiocyanate)/TRITC (thiol-reactive tetramethylrhodamine-5-(and-6)- isothiocyanate) | Kuster et al., 2004a; Odhiambo et al., 2011 |
| Belling’s iron aceto-carmine | Hackett and Macpherson, 1965 |
| Casarett stain | Casarett, 1953 |
| Diff-Quik/Dip Quick® | Root Kustritz et al., 1998; Boersma et al., 2001; Pozor et al., 2012 |
| Eosin–nigrosin | Hackett and Macpherson, 1965; Brito, 2007; Freneau et al., 2010 |
| Farelly | Paufler, 1974; Boersma et al., 1999, 2001 |
| Giemsa | Hancock, 1952; Hackett and Macpherson, 1965 |
| Harris’ haematoxylin | Boersma et al., 2001 |
| Heidenhain’s iron haematoxylin | Hancock, 1952; Hackett and Macpherson, 1965 |
| India ink | Hackett and Macpherson, 1965 |
| Papanicolaou | Boersma et al., 1999, 2001 |
| SpermBlue® | Van der Horst and Maree, 2009 |
| Williams stain (carbolfuchsin–alkaline methylene blue) | Williams, 1920 |
General morphology and acrosome | Spermac® | Oettle, 1986; Boersma et al., 2001; Baran et al., 2004 |
Acrosome | Anti-acrosin–FITC | Garner et al., 1975; Thomas et al., 1997 |
| Chicago sky blue–Giemsa | Kútvölgyi et al., 2006 |
| Chlortetracycline (CTC) | Gillan et al., 1997; Maxwell and Johnson, 1997; Green and Watson, 2001; Rathi et al., 2001 |
| Fast Green FCF–eosin B | Wells and Awa, 1970 |
| Giemsa | Watson, 1975 |
| Lectin conjugates – PSA (Pisum sativum agglutinin), PNA (peanut agglutinin) | Garner et al., 1986; Graham et al., 1990; Cheng et al., 1996; Gillan et al., 1997; Rathi et al., 2001; Herrera et al., 2002; Nagy et al., 2003; Waterhouse et al., 2006; Celeghini et al., 2007; de Graaf et al., 2007; Garcia-Macias et al., 2007; Petrunkina and Harrison, 2010; Odhiambo et al., 2011; Waberski et al., 2011; Cheuquemán et al., 2012 |
| Lysotracker Green DND-26 (LYSO-G) | Thomas et al., 1997, 1998 |
| Merocyanine 540 | Rathi et al., 2001; Gadella and Harrison, 2002; Kavak et al., 2003; Peña et al., 2004b; Garcia-Macias et al., 2007 |
| Naphthol yellow S–aniline blue | Christensen et al., 1994 |
| Naphthol yellow S–erythrosin b | Christensen et al., 1994 |
| Nigrosin-eosin-Giemsa | Tamuli and Watson, 1994 |
Fluo-3 | Parrish et al., 1999; Green and Watson, 2001; Petrunkina et al., 2001; Peña et al., 2004a; Okazaki et al., 2011 | |
| Fura-2 | Zhou et al., 1990 |
DNA | Fuelgen | Hackett and Macpherson, 1965; Ball and Mohammed, 1995 |
DNA integrity (Comet) | Electrophoresis assay/ethidium bromide/SYBR® Green | Linfor and Meyers, 2002; Fraser and Strzezek, 2004, 2005; Boe-Hansen et al., 2005a |
DNA integrity (FISH) | Cy3 probe, DAPI (4′,6-diamidino-2-phenylindole) | Fernández et al., 2003 |
DNA integrity (SCD – Sperm Chromatin Dispersion test) | DAPI/propidium iodide/Wright’s stain | Fernández et al., 2003; Garcia-Macias et al., 2007 |
DNA integrity (SCSA® – Sperm Chromatin Structure Assay) | Acridine orange | Ballachey et al., 1987, 1988; Evenson et al., 1994; Gadella and Harrison, 2002; Boe-Hansen et al., 2005b, 2008; Love et al., 2005; Waterhouse et al., 2006; Didion et al., 2009; Teague et al., 2010; Taskmakidis et al., 2010; Waberski et al., 2011 |
DNA integrity (TUNEL) | dUTP nick end labelling/fluorescein/FITC-streptavidin/propidium iodide | Gadella and Harrison, 2002; Waterhouse et al., 2006; Teague et al., 2010 |
Mitochondrial function | JC-1 | Shaffer and Almquist, 1948; Gravance et al., 2000; Celeghini et al., 2007; Garcia-Macias et al., 2007; Brinsko et al., 2011b; Cheuquemán et al., 2012 |
| MitoTracker Red® | Gadella and Harrison, 2002; Waterhouse et al., 2006; Celeghini et al., 2007 |
| MitoTracker Green FA Shaffer and Almquist, 1948 Rhodamine 123 | Shaffer and Almquist, 1948; Celeghini et al., 2007; de Graaf et al., 2007 |
Viability/membrane integrity | Annexin-V–FITC | Anzar et al., 2002; Gadella and Harrison, 2002; Peña et al., 2003; Cheuquemán et al., 2012 |
| Bromophenol blue | Boguth, 1951 |
| Calcein acetylmethyl ester (CAM)– ethidium homodimer-1 (EH) | Althouse and Hopkins, 1995; Juonala et al., 1999 |
| Carboxy(methyl) fluorescein diacetate (CFDA) | Garner et al., 1986; Harrison and Vickers, 1990; Donoghue et al., 1995 |
| Chicago sky blue–Giemsa | Kútvölgyi et al., 2006 |
| Congo red–nigrosin | Hackett and Macpherson, 1965 |
| Eosin B–aniline | blue Hackett and Macpherson, 1965; Garner et al., 1997 |
| Eosin–nigrosin | Hackett and Macpherson, 1965; Graham et al., 1990 |
| Erythrosin–nigrosin | Hackett and Macpherson, 1965 |
| Ethidium homodimer-1 (EH) | de Graaf et al., 2007 |
| Fast Green FCF–eosin B | Hackett and Macpherson, 1965; Wells and Awa, 1970; Aalseth and Saacke, 1986 |
| Garner et al., 1986 | |
| Hoechst 33258 | Garner et al., 1986; De Leeuw et al., 1991; Casey et al., 1993; Juonala et al., 1999; Herrera et al., 2002 |
| Hoechst 33342 | Keeler et al., 1983; Celeghini et al., 2007 |
| Nigrosin–eosin | Hancock, 1952 |
| Nigrosin–eosin–Giemsa | Tamuli and Watson, 1994 |
| Propidium iodide (PI) | Graham el al., 1990; Harrison and Vickers, 1990; Papaioannou et al., 1997; Celeghini el al., 2007 |
| Propidium iodide (PI)–CFDA | Garner et al., 1986; Almid and Johnson, 1988; Tamuli and Watson, 1994; Magistrini et al., 1997 |
| SNARF-1–PI–FITC-PSA | Peña et al., 1999; Kavak et al., 2003 |
| SYBR-14/Propidium iodide (PI) | Garner et al., 1994; Garner and Johnson, 1995; Magistrini et al., 1997; Nagy et al., 2003; Garcia-Macias et al., 2007; Boe-Hansen et al., 2008; Petrunkina and Harrison, 2010; Brinsko et al., 2011b; Cheuquemán et al., 2012 |
| SYTO® 17 | Thomas et al., 1997 |
| Trypan blue–Giemsa | Hackett and Macpherson, 1965; Didion and Graves, 1988; Kovács and Foote, 1992 |
YoPro-1 | Rathi et al., 2001; Gadella and Harrison, 2002; Kavak et al., 2003; Peña et al., 2004b; Waterhouse et al., 2006 |
5. Loosen the knurled condenser locking screw on the side of the condenser mounting ring (see Figs 6.10 and 6.11).
6. Insert the phase condenser into its mounting ring. The top sleeve of the condenser should be inserted completely ‘up’ into the mounting ring.
7. Tighten the knurled locking screw to secure the condenser in position.
8. Place a specimen drop on a microscope slide and cover with a coverslip.
9. Place the slide on the microscope stage and adjust the stage so that the edge of cover glass is under the 10× lens.
10. Rotate the phase adjustment control of the condenser so that the bright-field (BF) setting is seen at the front.
11. Close the condenser diaphragm using the control lever on the bottom side of the condenser (see Fig. 6.12).
12. Focus on the edge of the coverslip.
13. Move the slide so that the coverslip is approximately centred under the objective.
14. Remove one eyepiece (ocular) and insert the phase centring telescope.
15. Rotate the condenser adjustment control so that the ‘10’ position is in the forward position.
16. Loosen the knurled locking screw on the side of the centring telescope.
18. While looking into the centring telescope, push inwards with each hand the two condenser centring screws that extend outwards from each side of the phase condenser. When completely pushed in, these screws engage centring screws within the condenser assembly.
19. Continue to look into the centring telescope and turn one of the condenser centring screws clockwise while turning the other centring screw counterclockwise. Small adjustments should be made. Note the movement of the white phase ring of the condenser (the black phase ring is actually located in the objective and is not moveable). Continue to turn both centring screws in small increments until the white ring is centred directly over the black ring (see Figs 6.13 and 6.14).
Fig. 6.14. Aligning the phase rings of the phase microscope.
20. Disengage the two condenser centring screws by allowing/pulling both to retract to their ‘out’ position. Do not attempt to rotate the condenser adjustment control while the condenser centring screws are in their ‘in’ position.
21. Rotate the condenser control to position ‘20‘ and then rotate the 20× objective to the ‘use‘ position. Repeat the phase rings centring procedure (steps 18–20), if necessary.
22. Repeat the centring steps for the 40× (condenser position ‘40‘) and 100× (condenser position ‘100‘) objectives, if necessary.
23. Remove the centring telescope and replace with the eyepiece. The microscope is now ready for use.
Note: When the condenser is in the phase positions (10, 20, 40 or 100), the condenser diaphragm should be in the completely open position. When using bright-field microscopy, e.g. with stained specimens, rotate the condenser control to position ‘BF’ and adjust the diaphragm as desired.
Specimen observation
1. Prepare a slide of the semen sample with a coverslip and place on the microscope stage.
2. Position the slide such that the edge of the coverslip is centred under the 10× objective, with the condenser control positioned at ‘10’.
3. Focus on the edge of the coverslip.
4. Using the stage movement controls, move the slide so that the centre of the coverslip is approximately under the objective lens.
5. Focus the image, primarily using the ‘fine’ focus knob.
6. Change the magnification by turning the nosepiece to the desired objective. Set the condenser control to the setting matching the objective.
7. When using phase contrast lenses, the green filter may be fitted on to the recess of the light housing or in a filter holder under the condenser. A blue filter is often employed when using bright-field microscopy, but this may also be used for alternative effects during phase microscopy.
Differential interference contrast (DIC) microscopy is another technique used to enhance contrast of objects using a light microscope. DIC provides an appearance of the 3D structure of viewed objects. The physics of this technique has also been reviewed by Abramowitz (1987). The techniques to set up DIC differ by microscope design and model, are quite complicated, and therefore will not be summarized here. The reader is referred to documentation accompanying individual microscopes.
Wet smears using phase or DIC microscopy may be performed on spermatozoa that are unfixed (Saacke and Marshall, 1968) or fixed with gluteraldehyde or formaldehyde (Hancock, 1957; Pursel and Johnson, 1974; Johnson et al., 1976; Barth and Oko, 1989; Richardson et al., 1992), or on spermatozoa that have had their motility inhibited with sodium fluoride (Pursel et al., 1972, 1974). Potential artefacts associated with the staining and preparation of smears are avoided by the use of wet mounts.
Morphological evaluations are performed at 400–1000× magnification, e.g. 40× or 100× (oil) objectives and 10× oculars. Several morphological classification systems have been used. In some laboratories, morphological abnormalities are classified as being ‘primary’ (originating in the testes), ‘secondary’ (originating during epidydimal passage) and ‘tertiary’ (occurring post ejaculation). Blom (1972), as referenced by Barth and Oko (1989), suggested a ‘major’ and ‘minor’ classification scheme, according to the importance of the defect with respect to fertility. With these schemes, however, it is often difficult or impossible to classify a particular defect. Often, the origin of the defect is unknown, its importance is unclear, and/or spermatozoa may exhibit more than one defect. A simpler classification may then be used, as follows: abnormal head, abnormal acrosome, abnormal tail, proximal cytoplasmic droplets, distal cytoplasmic droplets and, of course, ‘normal’. COTS, debris, etc., should also be noted.
SEM and TEM can also be used to identify and study specific sperm abnormalities. These techniques require specific and meticulous sample preparation. Individual microscopes are expensive. Samples are often transferred to specific laboratories specializing in these techniques.
Irrespective of the classification scheme used, in general a maximum level of 15–20% total abnormal sperm is often considered acceptable. However, whenever performing analyses, it is critical to understand that some abnormalities, e.g. nuclear vacuoles, are extremely significant with respect to fertility. Also, observed abnormalities may be indicative of abnormalities that cannot be observed, e.g. DNA defects. In these cases, the general consideration of 15–20% as acceptable must be reconsidered. In essence, this issue is the basis of the ‘major’ and ‘minor’ classification scheme proposed by Blom (1972).
It must also be realized that a morphological evaluation is merely a snapshot of the ability of the male to produce ‘normal’ spermatozoa. The analysis is performed on a sample collected on a particular day and is indicative only for that particular collection. If the male is young, has been ill, or has been exposed to environmental conditions detrimental to sperm production, a satisfactory semen analysis may indicate adequate/acceptable semen production. Likewise, an unsatisfactory analysis only indicates that on that particular day, the collection consisted of abnormal cells. In both cases, repeated analyses must be done to clearly elucidate the seminal status.
After several satisfactory analyses, repeated in-depth analyses must be done on a periodic basis, depending upon the frequency of semen collection. In between in-depth analyses, laboratory personnel should be aware of abnormal sperm during routine microscopic semen evaluations, e.g. for motility. Salisbury and Mercier (1945) provided evidence that data obtained by examination of 100 spermatozoa on one slide was as reliable as the examination of multiple slides and the examination of up to 500 cells, although differences between technicians may account for greater variation (Root Kustritz et al., 1998; Brito et al., 2011). The proportion of cells examined is, then, extremely low (100–500) compared with the total cells (billions) in the ejaculate. Kuster et al. (2004b) discussed the statistical implications of sample size when performing morphological analyses.
Biological stains, DIC, SEM and TEM have all been used to demonstrate various sperm abnormaties: in bulls – Blom (1948), Saacke and Marshall (1968), Saacke (1970), Lorton et al. (1983), Barth and Oko (1989), and Foote (1999); dogs – Root Kustritz et al. (1998); stallions – Blom (1948) and Brito (2007); and boars – Kojima (1977), Tamuli and Watson (1994) and Briz et al. (1996). See also Chapter 3 (Kaya et al.) and Chapter 7 (Chenoweth and McPherson) for reviews of environmental and genetically induced abnormalities, respectively. Phase and DIC microscopy of wet smears has been used extensively to characterize acrosomal changes due to sperm ageing in vitro (Pursel et al., 1972, 1974; Saacke and White, 1972; Johnson et al., 1976; Aalseth and Saacke, 1986) and after freezing-thawing of ejaculates (Mitchell et al., 1978).
Sperm membrane integrity
Sperm membrane integrity has been used extensively to evaluate sperm quality using three methods: (i) stains – in bulls (Garner et al., 1994; Brito et al., 2003), in boars (Fraser et al., 2001) and in stallions (Brinsko et al., 2011b); (ii) incubation in hyperosmotic media – in rams (Curry and Watson, 1994) and in stallions (de la Cueva et al., 1997); and (iii) incubation in hypo-osmotic media – in bulls (Bredderman and Foote, 1969; Rota et al., 2000; Quintero-Moreno et al., 2008; Rubio et al., 2008; Sahin et al., 2008), in dogs (Hishinuma and Sekine, 2003; Pinto and Kozink, 2008), in donkeys (Rota et al., 2010), in rams (Curry and Watson, 1994), in stallions (Neild et al., 1999; Almin et al., 2010), and in turkeys (Donoghue et al., 1996). The SYBR-14 and PI stain combination is commercially available from Life Technologies (formerly Invitrogen) Molecular Probes® LIVE/DEAD® Sperm Viability Kit for use with the flow cytometer and fluorescence microscope. Johansson et al. (2008), Foster (2009), Morrell et al. (2010) and Foster et al. (2011a,b) have validated the use of the NucleoCounter® SP-100™ for measurement of membrane integrity. Because of its simplicity and assay speed (approx. 2 min for both sample preparation and the total cell and membrane integrity assays), the SP-100™ is easily adapted for use in the andrology laboratory. The flow cytometer has also been used to determine membrane integrity (Thomas et al., 1997; Nagy et al., 2003; Morrell et al., 2010; Foster et al., 2011a; see Plate 5). Flow cytometers are expensive and require specialized training, although Odhiambo et al. (2011) demonstrated the availability and accuracy of a relatively simple and easy-to-use instrument.
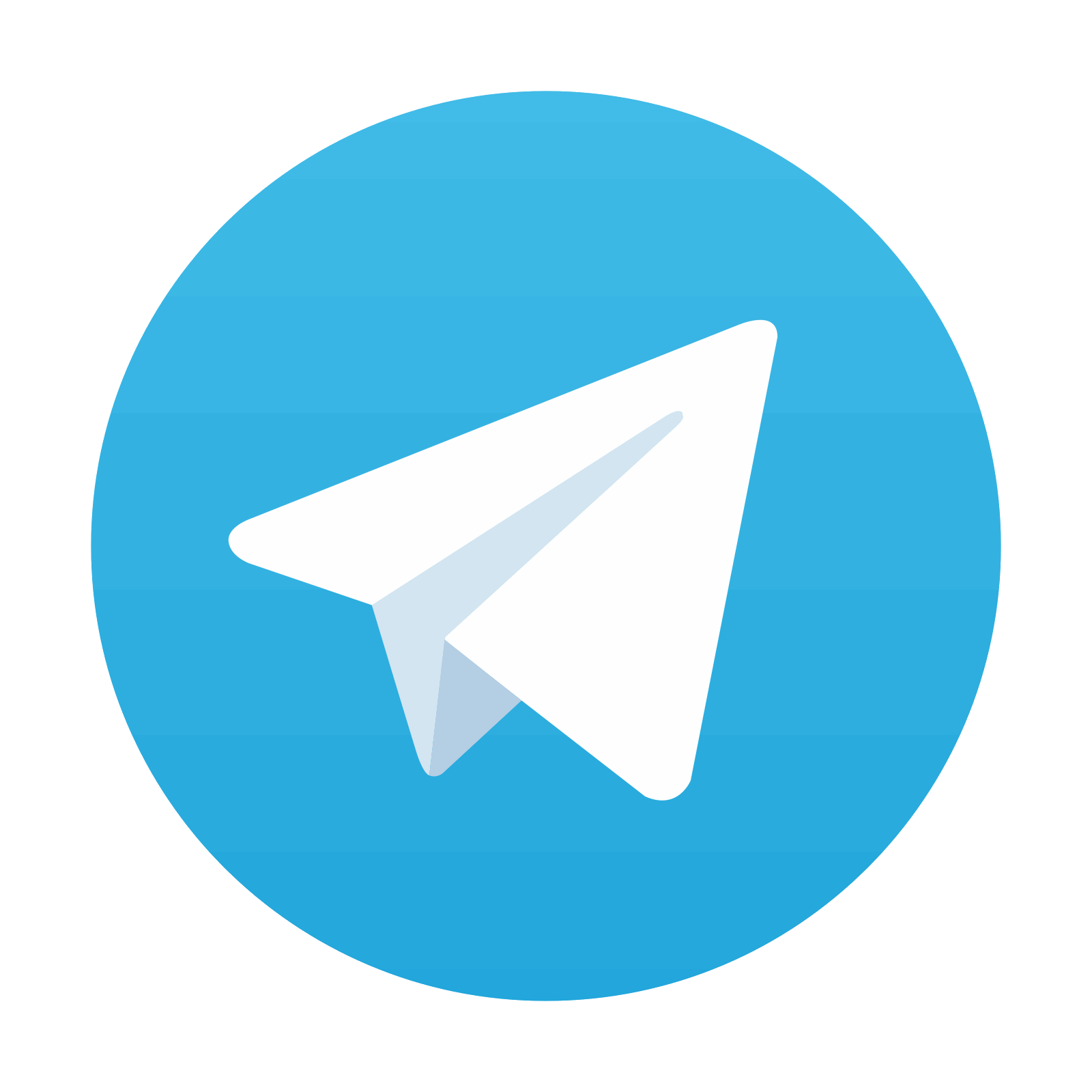
Stay updated, free articles. Join our Telegram channel

Full access? Get Clinical Tree
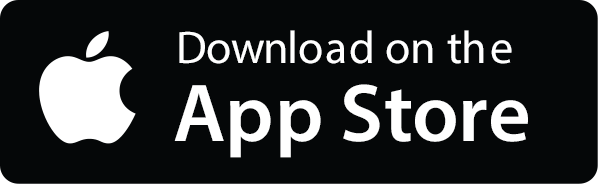
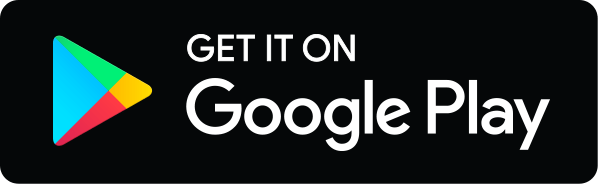