CHAPTER 60 Equine Piroplasmosis
Equine piroplasmosis (EP) is a tick-borne protozoal disease of horses, mules, donkeys, and zebras that is characterized by acute hemolytic anemia.1–6 The etiologic agents are two hemoprotozoan parasites, Babesia equi (Laveran, 1901)7 and Babesia caballi (Nutall and Strickland, 1910),8 that are transmitted primarily by ixodid ticks. Equine piroplasmosis is found globally where tick vectors are present and is endemic in tropical, subtropical, and some temperate regions.1–3,5,9–11 Horses infected with B. equi remain seropositive for life; horses infected with B. caballi are seropositive for several years to life.3,5,10–12
Economic losses associated with EP are significant and include the cost of treatment, especially in acutely infected horses; abortions; loss of performance; death; and restrictions in meeting international requirements related to exportation or participation in equestrian sporting events.13 Equine babesiosis–free countries, including the United States, limit the entrance of Babesia-seropositive horses into their countries. Equine piroplasmosis is synonymous with equine malaria,14,15 equine biliary fever,14–16 equine babesiasis,15 Oyns,16 horse tick fever,15 equine babesiosis, and equine theileriosis.17
ETIOLOGY
The genera Babesia and Theileria belong to the family Piroplasmidae. The term piroplasm is derived from the pear-shaped appearance of intraerythrocytic replicative forms. The piroplasms are members of the phylum Apicomplexa, which also includes the genera Plasmodium, Cryptosporidium, and Toxoplasma. B. caballi is regarded as a true Babesia because it exclusively replicates within erythrocytes in the vertebrate host (Fig. 60-1). Currently, there is uncertainty as to the appropriate taxonomic classification for B. equi. Although considered a “small Babesia,” B. equi has several characteristics that distinguish it from other species within the genus, including apparent initial development in lymphocytes before the erythrocytic stage18,19 (as occurs with Theileria spp.), division into four merozoites within the erythrocytes (forming the “Maltese cross,” Fig. 60-2), only transtadial transmission by ticks (not transovarial), and resistance to babesicidal drugs.11,20,21 Phylogenetic studies using small-subunit ribosomal ribonucleic acid (rRNA) gene analysis suggest B. equi belongs to a distinct paraphyletic group, different from both the Babesia and the Theileria genus.22 Currently, both nomenclatures may be found interchangeably in the literature. Because both Babesia and Theileria are piroplasms, the term equine piroplasmosis conforms with either classification.
Babesia caballi and B. equi share many of the same tick-vectors, are usually present in the same geographic regions, and frequently co-infect horses. B. caballi and B. equi are transmitted by more than 15 species of the tick genera Dermacentor (Fig. 60-3), Hyalomma, and Rhipicephalus.2,23,24 B. equi can also be transmitted by Boophilus microplus.25–27 Ticks serve as a reservoir for B. caballi because the organism persists in ticks throughout several generations, with transtadial and transovarial transmission (although not in all tick species). In contrast, horses are the only known reservoir for B. equi, and only transtadial (not transovarial) transmission occurs within the tick vector. Because of their longevity and mobility, male ticks can transmit B. equi to multiple horses.5 Importantly, Boophilus microplus may acquire and transmit B. equi even when feeding on inapparent carrier horses with a low level of parasitemia.28 Adult stages of Hyalomma anatolicum and Rhipicephalus turanicus transmit B. equi to horses after acquisition of the organism in nymph stages from infected horses under experimental conditions.5
Parasitemia (percentage of erythrocytes infected) typically does not exceed 1% in B. caballi infections and may be as low as 0.1% even in clinical cases.3 Maximum reported parasitemia is 10%.9 B. equi parasitemia usually ranges from 1% to 7%,5 with a maximum of 95%.9 Once infected with B. equi, horses remain carriers for life, regardless of whether clinical signs resolve naturally or with drug treatment.10 Infection with B. caballi has been said to be self-limiting, lasting up to 4 years after infection.9 However, many horses that recover from B. caballi infection later relapse, suggesting temporary nondetection of organisms with possible lifelong infection. Carrier horses represent a potential reservoir for maintenance and dissemination of parasites to ticks and horses.23 Because new tick species capable of transmitting equine piroplasms are being recognized and reliable control methods do not currently exist, it is important to prevent the introduction of both infected horses and ticks into equine piroplasm-free areas.
No evidences exists for transmission of EP by hematophagous dipterans such as tabanids (horsefly) or Stomoxys calcitrans (stable fly).23,29 B. equi can be transmitted iatrogenically by contaminated needles or surgical instruments, administration of contaminated blood transfusions, or failure to properly sterilize equipment that contacts equine blood (e.g., stomach tubes, dental instruments).5,20 Chronically infected pregnant mares are at risk of transplacental transmission, resulting in abortion, stillbirth, or birth of a sick foal that typically succumbs to the disease. B. equi is most frequently involved in these cases.3,30,31 Transmission of parasites in semen has not been documented; however, this may be possible if blood contamination of semen occurs.32
Life Cycle of Babesia caballi
The life cycle of B. caballi is typical of most Babesia species in that only erythrocytes are targeted in the mammalian host (Fig. 60-4). Infection is initiated by feeding of infected ticks, most often Dermacentor nitens, on a naive equine host. Sporozoites immediately invade erythrocytes. Within the erythrocyte, the parasite develops from a small anaplasmoid body (trophozoites), consisting predominantly of nuclear material, into a larger ameboid sphere that divides into two large pyriform bodies (merozoites) measuring approximately 2 to 5 μm long and 1.3 to 3 μm in diameter. When an uninfected tick subsequently feeds on the infected horse and ingests parasitized erythrocytes, most parasites are destroyed within the tick’s midgut. However, some merozoites survive and form small, round bodies floating free within the gut contents of the tick. These spherical bodies give rise to large, clavate (club-shaped) bodies that penetrate the epithelial cells of the midgut and undergo multiple fissions. This is followed by infection of a variety of tick tissues, where a secondary cycle of multiple fissions occurs. Infected tissues in the female tick include all tissues and eggs except for the salivary glands. Small pyriform bodies produced in the salivary glands of the larvae, nymphs, and adults of the next tick generation are infective to the horse if these tick forms are feeding.33 The large, paired merozoites joined at the posterior ends in the equine erythrocytes are a diagnostic feature of B. caballi infection5 (see Fig. 60-1).
Life Cycle of Babesia equi
The details of the life cycle of B. equi may vary depending on the tick species involved.34–37 Infected ticks feed on horses and inject B. equi sporozoites into the new mammalian host with their saliva. It has been reported that sporozoites initially are able to penetrate lymphocytes and form large microschizonts and macroschizonts (schizogony). These parasite forms ultimate give rise to approximately 200 merozoites per infected cell, each measuring approximately 1.5 to 2 μm in length. Merozoites invade erythrocytes and reproduce by binary fission (merogony), forming pyriform stages measuring approximately 2 to 3 μm. In erythrocytes, asexual division gives rise to four pear-shaped stages measuring approximately 2 μm and appearing as a “Maltese cross” form (see Fig. 60-2). After rupture of infected erythrocytes, merozoites enter new erythrocytes and continue to replicate. Some merozoites eventually become spherical in shape, forming ring forms, believed to be gamonts. When a tick ingests gamonts, gamonts grow in the tick’s midgut, start nuclear reproduction, and initiate formation of “ray bodies” (protrusions). By 4 to 6 days after ingestion, ray bodies divide and form microgamonts and macrogamonts, which will fuse, forming zygotes (sexual replication). Inside the zygote a kinete is formed, which will later penetrate through the midgut of the tick into the hemolymph. Kinetes are observed 5 to 7 days after tick feeding and usually last 6 to 9 days. Kinetes penetrate into salivary gland type III cells 7 to 8 days after attachment. In these cells, sporonts, sporoblasts, and then sporozoites are formed (sporogony). Sporozoite development is typically complete between day 6 and 24 after completion of tick feeding.17
EPIDEMIOLOGY
Few countries in the world are free from autochthonus (native) infections with EP, and it is estimated that only 10% of horses globally inhabit regions that are free of EP. The countries currently recognized as nonendemic by the World Organization for Animal Health (OIE) include Australia, Canada, United States, England, Ireland, and Japan; the OIE website, http://www.oie.int, is available to confirm national information. Many areas currently free of EP are climatically suitable for appropriate tick vectors or already possess competent tick vectors.3 Thus, there is the continual possibility of introducing B. equi and B. caballi into Babesia-free areas either by infected ticks or horses.
The worldwide prevalence of EP is consistent with worldwide distribution of competent tick vectors. Because B. equi and B. caballi share the same vectors in a given region, they are closely associated and are endemic in tropical and subtropical climates. In most regions, however, infections with B. equi are more common than infections with B. caballi.2,3,20 Outbreaks of overt clinical disease are uncommon in endemic areas, with the exception of India, despite the endemic status of implicated parasites.38 Outbreaks in Germany, Switzerland, and Australia in the past were attributed to the use of contaminated needles or instruments rather than tick transmission.2 Acute clinical disease is most often observed when naive horses are moved into endemic areas.39
Babesia equi and B. caballi are endemic in almost all of Latin America, except for the southern parts of Chile and Argentina. Almost all horses in Brazil,13,40–42 Colombia,43 Puerto Rico, and Mexico2,5 are seropositive for B. equi and B. caballi. Despite the widespread distribution of infection and the intense tick parasitism of the horse population in many parts of Latin America (see Fig. 60-3), data are limited regarding which ticks are responsible for transmission of EP in these countries. B. caballi is transmitted by Dermacentor (Anocentor) nitens, the “tropical horse tick.”40 Boophilus microplus is believed to be one of the ticks involved in the transmission of B. equi.26,37 In a study in Brazil using nested polymerase chain reaction (PCR), both B. equi and B. caballi were identified in B. microplus ticks.27 The significance of this finding is uncertain because these ticks are not generally considered to be a vector for B. caballi. Boophilus microplus primarily feeds on cattle, although it will occasionally feed on horses, especially if horses are kept in pasture with cattle. As a result, the seroprevalence of B. equi is higher in horses in close contact with cattle.26,37,41 The most common species of tick found on horses in Brazil are Dermacentor (Anocentor) nitens (see Fig. 60-3) and Amblyomma cajennense.26,40,41 Intense parasitism by these ticks is observed in horses at an early age in many regions of the country. In 1995, Barbosa et al.40 found that 100% of foals in southeastern Brazil seroconvert to B. equi by 127 days of age and to B. caballi by 150 days. Yet, tick surveys using microscopy40 or PCR41 and experimental transmission studies fail to support A. cajennense as a natural tick vector for equine piroplasmosis.2
Babesia equi and B. caballi are widely present in Portugal, Spain, France, and Italy. Piroplasmosis is also endemic in the Balkan Peninsula, Hungary, Romania, several countries of the Commonwealth of Independent States (CIS, the southern parts of Russia), and the states of the Caucasus region. In central Russia, B. caballi infections have a common distribution with the tick vector Dermacentor reticulatus.2,5 Most infections of horses in nonendemic European countries have been traced back to Spain, France, Italy, or the CIS. Belgium, Switzerland, Austria, the Czech Republic, and Poland are considered infection free, although autochthonous infections occasionally occur.2,3,5 Potential vectors of the genus Dermacentor are present in most Northern European regions. Hyalomma and Rhipicephalus ticks predominate in southern Europe, the southern regions of Russia, and the CIS.2
Morocco,45 Republic of South Africa, Madagascar, and nearly all other parts of the African continent are considered endemic for B. equi and B. caballi.2,12,46–48 Virtually all horses and zebras are infected, except in a few regions. The seroprevalence in these countries is generally greater than 50% and 60% for B. equi and B. caballi, respectively. Ticks involved in transmission include Rhipicephalus evertsi evertsi, which can transmit both B. equi and B. caballi,46 and Hyalomma truncatum, which transmits B. caballi.49 An association between gender and the occurrence of antibodies against B. caballi has been observed in South Africa, with colts more likely to have antibodies than fillies.50 This may be linked to tick gender predilection, because male horses in some studies have a higher burden of certain tick species than females.50
Babesia equi and B. caballi are widespread in the horse populations of Mongolia, China, and many parts of Southeast Asia and Asia.27,51–53 In both China and Mongolia, B. equi is more prevalent and is primarily transmitted by Dermacentor nuttallii.54,55 High infection rates are similarly reported in Korea.5 Historically considered free of infection, recent reports demonstrate both B. equi–seropositive and B. caballi–seropositive horses in Japan.56 The tick Haemaphysalis longicornis has been suggested as a potential vector for EP in Japan and was shown to acquire B. caballi from experimentally infected mice with severe combined immunodeficiency (SCID).57 Further studies are necessary to verify if acquisition and transmission occurs in horses under natural conditions.56 The “brown dog tick,” Rhipicephalus sanguineus, listed as a potential B. equi vector, is also present in Japan. (This tick has been shown to transmit B. caballi, although it is not believed to be involved in the natural transmission of the disease because of its habit of feeding almost exclusively on dogs.1,23)
High infection rates have also been reported in the Middle East, including Kuwait, Oman, and India, where Babesia equi is reported to have higher seroprevalence. Ticks involved are of the Hyalomma and Rhipicephalus species.2,5,58,59 Jordan recently has reported fatalities in strenuously exercised horses with high-level B. equi parasitemia on blood smears and consistent necropsy findings.60
Although B. equi was introduced into Australia in the 1950s and 1960s with Quarter Horses imported from Texas and in 1976 with the importation of Andalusian horses from Spain, the organism did not become established in Australia. As in other nonendemic countries, transmission occurred by contaminated needles and instruments.2,3,5,20 Boophilus microplus is present in parts of Australia, and other tick vectors capable of transmitting EP could potentially be introduced by horses or other hosts.61,62
Babesia caballi and B. equi were introduced into the United States (U.S.) in 1959 when Cuban horses were imported. Limited B. caballi infections became established in Florida, southern Georgia, Texas, and some adjacent states in which the tick vector Dermacentor (Anocentor) nitens was present.9,15 Since then, a few new cases and epizootics have occurred in Florida, but with minor exceptions, further spread was prevented by intensive control measures.15,29,63–65 Despite sporadic occurrence of EP in the U.S.,66 it is considered free of EP. Dermacentor nitens, D. albipictus, and D. variabilis are potential tick vectors for B. caballi in the U.S. Boophilus microplus and D. variabilis are native to the U.S. and potential vectors of B. equi.24 B. microplus has been eradicated from the U.S. but remains endemic in Mexico. The development of acaricide resistance in the U.S. at the border with Mexico increases the potential for reemergence of this tick in the U.S.67
PATHOGENESIS
In piroplasmosis-endemic areas, most horses become infected within the first year of life. Mortality in native horses may range from 5% to 10%, depending on the strain of protozoa, the general health of the animals, and the availability of treatment. Mortality rates exceeding 50% may occur when previously unexposed mature horses are introduced into endemic areas. Importation of infected animals into southern France resulted in 69% mortality among untreated horses.1 The incubation period varies from 12 to 19 days for B. equi and 10 to 30 days for B. caballi and coincides with the peak in fever and erythrolysis.3
Alterations in erythrocyte membrane protein and lipid content and increases in plasma malondialdehyde during severe parasitemia suggest that accumulation of oxidative ions resulting from lipid peroxidation alters the erythrocytes’ biochemical composition, leading to hemolysis.68 These alterations may result in increased erythrocyte rigidity and decreased deformability, contributing to microvascular stasis.68 The packed cell volume (PCV) typically decreases to 20% but may fall to 10% or lower. Extreme anemia is more common in B. equi infections.29 Hemoglobinuria of varying severity, secondary to severe hemolysis, is observed in B. equi infection. Icterus, also caused by hemolysis, is the result of an increase in the indirect (unconjugated) bilirubin, which is deposited in mucosal surfaces and elsewhere, imparting a yellow color to those tissues. In the case of B. caballi, clumping of parasitized erythrocytes can lead to microvascular occlusion. Simultaneous thrombocytopenia and the systemic inflammatory response result in endothelial damage, increased vascular permeability, and in severe cases, disseminated intravascular coagulation (DIC). Severely affected horses experience edema, hemorrhage, ischemia, and anoxia, culminating in organ dysfunction.1
Thrombocytopenia has been described in association with EP and other protozoal infections. Although various theories as to its etiology have been proposed, the exact pathogenesis remains uncertain. Thrombocytopenia may be a result of low-grade DIC, as proposed in dogs with Babesia canis infections.69
Passage of infected erythrocytes across the placental barrier has been implicated as the probable mode of in utero transmission of disease and may result from damage to placental blood vessels, leading to mixing of maternal and fetal blood near or at the time of foaling.16 Not all foals from an infected mare are born infected, and the factors that determine whether prenatal infection occurs have not been identified.16
Protective immunity is present as long as a horse harbors the Babesia organism, which in most cases is for life. There is no cross-immunity between B. equi and B. caballi.1,29 The resistance to clinical disease acquired by horses after Babesia infection is a result of continued stimulation of immunity by the persisting parasite, but the exact mechanisms involved are unknown. Innate immunity likely plays a central role in control of Babesia parasites,70,71 although the precise role of neutrophils, macrophages, and natural killer (NK) cells in parasite control is not defined.
The spleen is important in eliminating piroplasms. Horses with intact spleens often control infection and survive, whereas splenectomized horses develop severe parasitemia and succumb to the infection.4,10,72,73 Despite its important role, the spleen and innate immunity are insufficient for protection against EP in the absence of adaptive immunity. In 1994, Knowles et al.4 demonstrated that foals with SCID become severely anemic with a high level of parasitemia and die after experimental infection with B. equi. SCID foals lack mature T and B lymphocytes and are incapable of antigen-specific immune responses, but they have intact spleens and a competent innate immune system. This demonstrates that the spleen is unable to control B. equi infection in the absence of parasite-specific immune responses, and that erythrolysis caused by B. equi does not require a specific immune response.
High antibody titers correlate with parasite control in horses.74,75 Immunoglobulin Ga (IgGa) and IgGb correlate with control of B. equi during the acute stage of infection, and IgG(T) increases during chronic infection.76 Specific antibodies are first detected 7 to 11 days after experimental infection in ponies and reach a peak 30 to 45 days after infection.77 B. equi–infected horses preferentially produce antibodies against highly conserved merozoite surface proteins, specifically equi merozoite antigens 1 and 2 (EMA-1 and EMA-2).25,78 Passively transferred antibodies to B. equi and B. caballi may persist in the foal for 4 to 5 months.79 However, foals born from B. equi–seropositive mares may be born infected without clinical disease and remain seropositive for life. Foals from endemic areas are particularly resistant to clinical disease.41
Despite the vigorous humoral immune response, in vivo and in vitro studies with serum of recovered animals failed to prove a full protective effect of antibodies,80 suggesting that humoral antibodies are also insufficient for protection against EP.75,80 Considering the importance of cell-mediated immune responses to other protozoan parasites, such as Theileria parva and Babesia bovis, it is expected that cell-mediated immunity plays a key role in immunity against B. equi and B. caballi.4,81 The cell-mediated response to Theileria spp. schizont-infected cells is thought to be responsible for protective immunity.81 Although this life-cycle stage may occur in B. equi, it does not seem to be as predominant as it is for Theileria species.
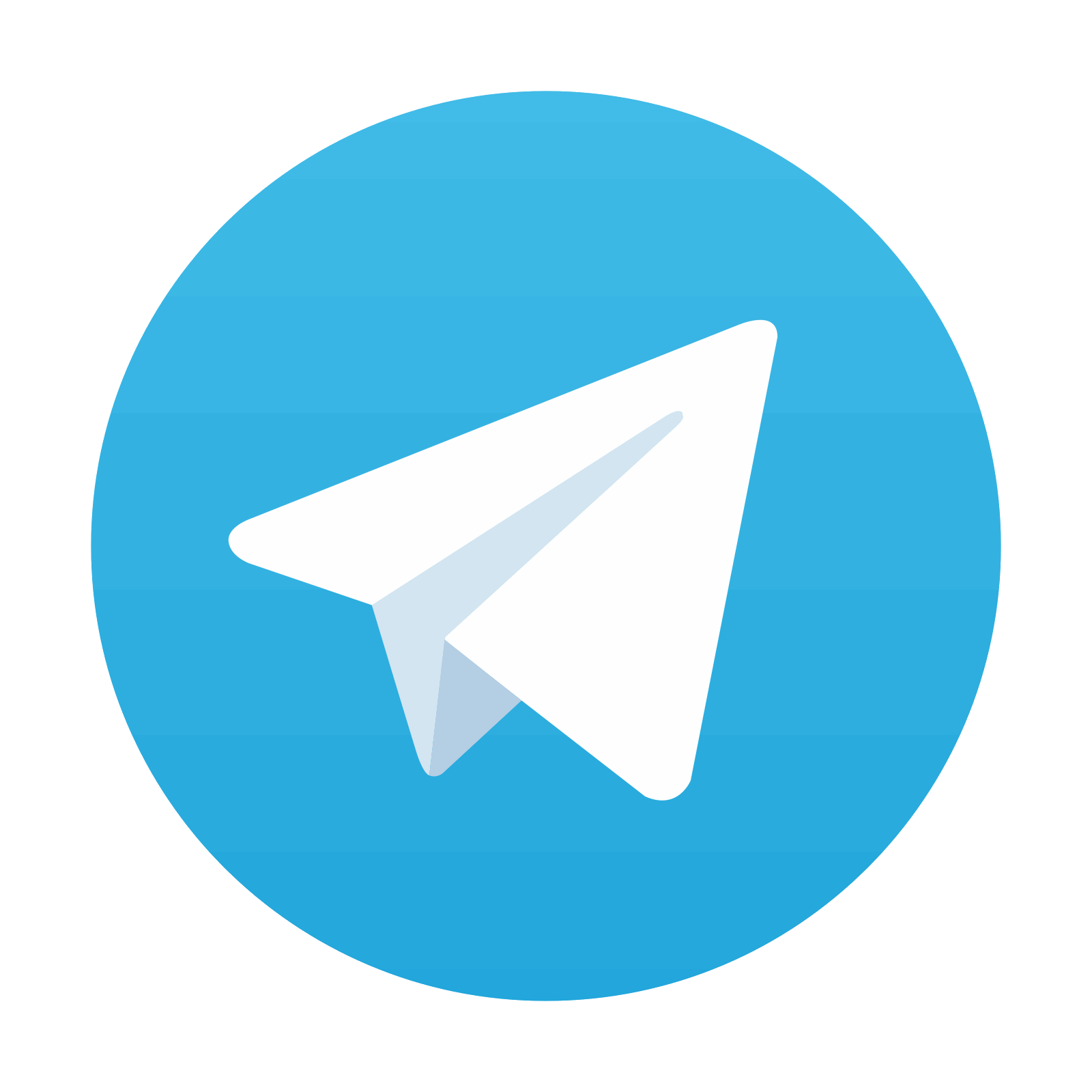
Stay updated, free articles. Join our Telegram channel

Full access? Get Clinical Tree
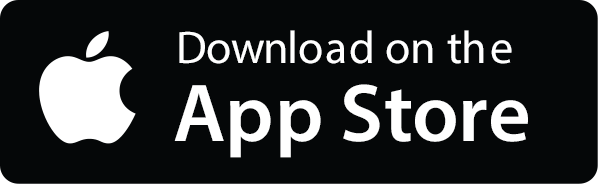
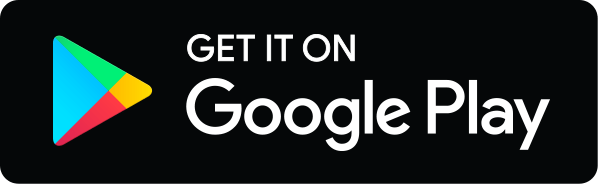