Raquel M. Walton1 and Cheryl A. Lawson2 1 IDEXX Laboratories, Inc., Langhorne, PA, USA 2 Department of Veterinary Pathology, College of Veterinary Medicine, Iowa State University, Ames, IA, USA The complete blood count (CBC) provides information beyond the concentrations of blood cells. Insight into disease processes, their severity, and even diagnoses can be gleaned from a complete evaluation of the CBC, especially in conjunction with a peripheral blood film. A single CBC is merely a “snapshot” in time; therefore, serial CBCs are often beneficial in better understanding a progressing or improving disease process. Blood submitted for a CBC should be collected and immediately mixed with EDTA, which is the preferred anticoagulant for mammalian blood. Ideally, analysis of the sample should occur promptly to prevent the formation of cellular changes such as swelling and degeneration, which can affect both blood smear analysis and the evaluation of the blood sample with an automated hematology analyzer. It is not uncommon in equine medicine for delays in sample analysis up to 24 hours to occur as a result of restricted access to diagnostic laboratories. Characteristic changes in blood parameters associated with delayed analysis of equine blood samples using a common hematology analyzer (Advia 120, Bayer Corporation, Tarrytown, NY) include increased numbers of normocytic, hypochromic red blood cells (RBCs), increased numbers of macrocytic, hypochromic RBCs, misclassification of granulocytes as mononuclear cells using the basophil reagent method, and a pseudothrombocytosis due to the categorization of lyzed erythrocytes as platelets. These changes are mitigated by storage at 24 °C rather than at 4 °C [1]. In general, equine blood differential leukocyte counts obtained from the Advia 120 show less precision compared with classic impedance methods and it is recommended that these instrument‐derived counts should be verified with manual differentials [2]. It is also suggested that blood samples be warmed to 37 °C prior to analysis. Warming EDTA blood samples reduces pseudothrombocytopenia secondary to platelet clumping, a common preanalytical error for platelet counts [3]. In health, erythrocyte lifespan in horses appears to vary between breeds, but is approximately 140–160 days [4]. After a hemorrhagic event, erythrocyte lifespan is shortened to an average of approximately 139 days [5] and 144 days after a hemolytic event [6]. The erythrogram typically comprises the following elements: RBC count (×106/μL); hematocrit or packed cell volume (PCV) (%); hemoglobin (Hb) concentration (pg/dL); mean cell volume (MCV) (fL); mean cell Hb (MCH) (pg); mean cell Hb concentration (MCHC) (g/dL). Calculated indices: The indices that are measured by the hematology analyzer include RBC count, Hb, MCV, and PCV. Knowledge of which indices are calculated and which are measured will help to determine possible artifacts in the erythrogram. For example, a discrepancy between the hematocrit and PCV (>2% difference) will point to a spurious MCV or RBC measurement. When there is agglutination, the hematocrit may be spuriously low as a result of the measured RBC count being lower than the true RBC count due to the presence of RBC aggregates that are not detected by the hematology analyzer. However, agglutination also may spuriously increase the MCV measurement when RBC doublets are measured as individual RBCs. If the spuriously increased MCV is in proportion to the spuriously decreased RBC count, the hematocrit may not be significantly different from the PCV. Another example of artifact‐associated change that may not affect measured values would be erythrocyte swelling associated with lithium heparin anticoagulant. Lithium heparin anticoagulant may cause spuriously high hematocrits as a result of RBC swelling causing spuriously high MCV [7]. However, the increased MCV will similarly affect the centrifuged hematocrit so there may not be a mismatch between the calculated hematocrit and PCV. As a control for the accuracy of the analyzer hematocrit, a spun hematocrit (PCV) should always be run for comparison with the hematocrit. In the absence of a PCV, the universal relationship between the mammalian Hb concentration and hematocrit can be used to determine the accuracy of the hematocrit; for mammals other than camelids, the hemoglobin should be one‐third of the hematocrit. For example, if the Hb concentration is 11 pg/dL, the hematocrit should be approximately 33%. Erythrocytosis is defined as an increased hematocrit and may be relative or absolute. Transient, absolute erythrocytosis in horses may occur as a result of splenic contraction (see Section 2.1.1.6). Hemoconcentration produces a relative erythrocytosis secondary to dehydration. Erythrocytosis due to hemoconcentration will produce concomitant increases in both the PCV and plasma protein concentration, whereas erythrocytosis from splenic contraction is not accompanied by alterations in plasma protein concentration [8]. Paraneoplastic erythrocytosis is rare in horses and has been reported with lymphoma, hepatoblastoma, hepatocellular carcinoma, and a carcinoma of unknown origin as a result of autonomous erythropoietin excretion by the neoplastic cells [9–12]. Neoplastic erythrocytosis (primary erythrocytosis or polycythemia vera) is very rare in equids with only a single confirmed case report in a 2‐year old Arabian gelding [34]. Similar to other species, equids release erythropoietin in response to hypoxemia caused by decreased erythrocyte circulating mass due to loss or hemolysis. Thus, regenerative anemias in horses occur as a result of hemorrhage or hemolysis from immune‐mediated damage, toxins, or oxidative damage. During immune‐mediated hemolytic anemia (IMHA), antibodies with or without complement accelerate erythrocyte destruction [13]. Immune‐mediated hemolytic anemia may be idiopathic or secondary to medications, neoplasia, or infectious disease. Oxidative damage in horses has been associated with red maple leaf toxicity, glucose‐6‐phosphate dehydrogenase deficiency, erythrocyte flavin adenine dinucleotide deficiency, onion ingestion, phenothiazine, and equine infectious anemia viral infection (EIAV). In horses, nonregenerative anemias are most often attributable to decreased erythropoiesis associated with inflammation or disease due to inflammatory cytokine effects, often referred to as “anemia of chronic disease.” This type of anemia is now more appropriately termed “anemia of inflammation.” The net effects of inflammatory cytokines result in decreased iron availability for erythropoiesis and direct suppression of erythropoiesis (Figure 2.1) [14]. Figure 2.1 Simplified overview of the mechanisms in anemia of inflammation that result in erythroid hypoplasia and nonregenerative anemia. Decreased plasma iron concentration was shown to have good sensitivity as a marker of systemic inflammation in horses [15]. The decrease in iron is a relative rather than absolute deficiency due to sequestration in macrophages. Absolute iron deficiency in adult horses is very rare. Inflammation can be associated with neoplasia, infectious disease, and immune‐mediated disease, resulting in nonregenerative anemia. However, these diseases can also cause hemolysis or hemorrhage, so some neoplasms, infectious agents, or immune‐mediated disease may be associated with regenerative anemia depending upon the net effect of stimulatory and inhibitory forces. Impaired renal function may result in anemia due to decreased production of erythropoietin, but also as a result of inflammation [14, 16]. The response to increased erythropoietin from most mammalian species is to release marrow reticulocytes into circulation, which can primarily affect MCV, MCH, and MCHC. The classic change in RBC parameters in regenerative anemia is therefore macrocytic and hypochromic in most species. In contrast, the typical regenerative response to anemia in horses is macrocytic and normochromic. Horses are unique amongst domestic mammalian species with respect to the lack of release of reticulocytes following mild to moderate anemia. While reticulocytes are produced within the marrow and increases in marrow reticulocytes are associated with regenerative erythroid responses, too few reticulocytes are released into circulation to be useful as an indicator of regeneration. Until the advent of automated reticulocyte enumeration methods that evaluate more than 40 times the number of erythrocytes evaluated by manual methods, reticulocytosis was not thought to occur in equine blood. Using laser methodology (Advia 120), small numbers of circulating reticulocytes (0.5–85 × 103/μL) can be detected in health [2]. Reticulocyte numbers vary slightly depending on breed and age, with cold‐blooded horses having approximately 20% fewer reticulocytes compared to other breeds and Thoroughbred foals with approximately 50% more reticulocytes compared to adults. Nonblood diseases such as colic or dysproteinemia resulted in approximately 36% greater numbers of reticulocytes while marked anemia resulted in 120% greater numbers of reticulocytes [17]. However, since there are only low circulating numbers of reticulocytes overall, the clinical use of reticulocyte numbers is limited to severe anemias in select situations such as anemia associated with immune‐mediated hemolysis [18] or with high‐dose erythropoietin administration [19]. A regenerative response to blood loss anemia in horses is reported to take about four days from the onset of RBC loss, with a maximal response seen at nine days [20]. Recovery to normal values after a hemolytic event takes about 1–2 months [6], whereas recovery from hemorrhagic anemia is of the order of 2–3 months [5]. Historically, the best indicator of a regenerative response in horses prior to increasing hematocrit is evaluation of bone marrow. However, erythrocyte indices can show characteristic changes indicative of a regenerative response, especially in severe hemorrhagic or hemolytic anemias. Macrocytosis, characterized by the release of macrocytes that are roughly twice normal size, is part of the maximal erythrocyte regenerative response. This macrocytosis is not strictly related to reticulocytosis as regenerative macrocytosis in horses and other species does not correlate with reticulocytosis [19]. Macrocytosis is one of the first and most consistent parameters to show change following anemia in horses and is a more sensitive indicator of regeneration than hematocrit. However, horses with effective regenerative responses do not always have macrocytosis as defined by increases above reference values, especially with mild blood loss or hemolytic anemias. In these cases, serial evaluation of individual MCVs was more sensitive in detecting macrocytosis than comparison with a population‐based reference interval [21]. Widening in the red cell distribution width (RDW) (discussed later) can also identify macrocytic subpopulations before the MCV increases above reference values. In horses, macrocytosis subsequent to anemia is associated with a decrease in the number of normocytes, which suggests that macrocytes remain large and do not contribute to the normocyte population [22]. Macrocytes persist after hematocrit and RBC counts have returned to preanemia levels, so macrocytosis in the presence of other normal erythrocyte values in horses may be an indicator of a recent regenerative response [21, 22]. Microcytosis is typically associated with absolute or functional iron deficiency or portosystemic shunting in many species. In horses, the most common cause of microcytosis is physiological and age associated, necessitating separate reference values for MCV in horses less than 9 months of age. In horses, microcytosis associated with absolute iron deficiency has not been reported. Documented iron deficiency anemia in a foal was characterized as normocytic and normochromic [23]. Functional iron deficiency attributable to iron sequestration (i.e., anemia of inflammation) may result in microcytosis and does appear to occur in horses. Reported cases of larval cyathostominosis associated with microcytosis attributed the finding to systemic inflammation and/or protein exudation associated with intestinal parasitism [24]. Most hematology analyzers will report the RDW with the erythrocyte indices. The RDW is a calculated value assessing the coefficient of variance of the erythrocyte volumes. In other words, it evaluates the amount of variation in the erythrocyte volumes and reflects the degree of anisocytosis. Increases in RDW are associated with blood loss and hemolytic anemias, as well as with erythropoietin administration [19, 21]. Similar to the MCV, increases in RDW due to macrocytosis are detectable in serial comparisons of individuals, but may not exceed population‐based reference intervals. Because the RDW can increase as a result of the emergence of smaller and/or larger erythrocyte populations, the distribution histogram itself can better identify the cause of increases in RDW. The impedance method (see Chapter 1) generates a histogram depicting the distribution of erythrocyte volumes (Figure 2.2). The RBC histogram is valuable in detecting the emergence of macrocytic and microcytic erythrocyte subpopulations. This is best accomplished by comparing serial histograms from a patient at weekly intervals. In horses, the histogram is especially useful because macrocytic subpopulations representing a regenerative response to anemia can be detected before the MCV rises above the reference interval [22]. Moreover, as discussed previously, not all horses with regenerative responses show changes in MCV above the reference interval, but macrocytic subpopulations are detectable on the histogram. Figure 2.2 Red blood cell (RBC) histograms from the Advia 120 (Bayer Corporation) hematology analyzer. (a) Histogram from a hematologically normal horse. The red line shows the mean cell volume (MCV) in femtoliters; the black lines represent the instrument’s preset range of equine RBC volume. (b) Histogram from a horse with a macrocytic anemia. Note the widening of the histogram to include a right shoulder. The MCV is still within the reference limits established for this instrument (38–55 fL), but there is an emerging population of macrocytes suggesting a regenerative response. (c) Histogram from a horse with a microcytic anemia. The whole population of RBCs is microcytic, resulting in a shift of the entire histogram to the left. This horse had an anemia of chronic disease. The mean cell hemoglobin (MCH) and mean cell hemoglobin concentration (MCHC) represent the quantity and the concentration of Hb, respectively, per average erythrocyte. Any increase in MCH and/or MCHC indicates artifact since it is not physiologically possible for these indices to increase outside the upper reference limit because Hb synthesis halts when the optimal amount of Hb is present within the erythrocyte cytoplasm. Mean cell hemoglobin and MCHC are indices calculated from RBC and Hb concentrations, thus increases are associated with spurious RBC or Hb measurements. RBC agglutination may cause increases in MCH or MCHC due to a spuriously low RBC count. However, as discussed previously, decreases in RBC count due to agglutination may be countered by spuriously increased MCV measurements, resulting in minimal impact on the MCH and/or MCHC. Another common cause of increased MCH and/or MCHC is the presence of lipemia, which results in spurious increases in the Hb measurement. Heinz bodies will also falsely increase MCH and MCHC when determined by laser hematology analyzers and will spuriously increase the Hb measurement with spectrophotometric methods. In vitro hemolysis will also increase the MCH and MCHC because the number of intact RBCs is disproportionately low for the amount of Hb measured. Decreases in MCH and/or MCHC are typically associated with regenerative responses to anemia in species that release reticulocytes in large numbers. Since horses do not usually release substantial numbers of reticulocytes into circulation in a regenerative response, the regenerative response is often normochromic. In other species, decreased hemoglobin concentration as a result of iron deficiency causes hypochromic (and microcytic) anemia, but in horses iron deficiency anemia is reported to be normochromic [23]. Relative to adults, erythrocyte number, Hb, and hematocrit (Hct) are increased at birth, decline sharply within 12–24 hours, and then show a gradual decline over the subsequent two weeks to levels at the lower end of adult reference intervals. This change is suspected to be due to the transfusion of placental blood to the foal with subsequent catecholamine release and fluid balance adjustment due to osmotic effects from absorption of immunoglobulins in colostrum [25]. Continued decline is thought to be due to factors such as decreased erythrocyte circulating lifespan, decreased iron delivery to the bone marrow, and reduced stimulation for erythropoietin production from higher hemoglobin saturation. The MCV is high at birth and decreases to reach a nadir at 3–5 months of age; values are microcytic relative to adult reference intervals until 9 months to 1 year of age [26]. The microcytosis is thought to be due to a relative iron deficiency from limited storage of body iron or low concentration of iron in the dam’s milk [23]. Breed effects on erythrocyte indices are reflected in higher Hct, Hb, and RBC counts in “hot‐blooded” breeds (Arabians and Thoroughbreds) compared with the “cold‐blooded” draught horse and pony breeds. In addition, Thoroughbreds have a smaller reported MCV compared to draught horses [26]. The use of breed‐appropriate and age‐specific reference values is therefore very important. The equine spleen can store up to a third of the RBC mass and rapidly transfer large numbers of erythrocytes into the systemic circulation following epinephrine‐induced splenic contraction [25, 27]. Epinephrine‐induced splenic contraction is associated with excitement or strenuous exercise. Depending upon the baseline PCV, splenic contraction may result in erythrocytosis or a normal PCV. The time taken for the PCV to return to baseline following contraction may be 40–60 minutes to up to several hours, depending upon the magnitude of the stimulus. In contrast, splenic RBC sequestration and congestion following barbiturate, alpha‐2 agonist, or halothane anesthesia may drop the PCV below baseline values [28]. Thus, the spleen’s large storage capacity may impact significantly on the circulating RBC mass. Anemia could potentially be masked following splenic contraction or simulated secondary to anesthetic‐induced splenic congestion and RBC sequestration. The leukogram includes the numeric and morphological data pertaining to white blood cells. The leukogram, like erythrocyte indices, can provide information regarding the presence of a pathological or pathophysiological process, but rarely leads to a specific diagnosis. There are distinct leukogram profiles associated with inflammation, corticosteroids, and epinephrine. Acute inflammation results in the release of mature neutrophils and bands from the marrow storage and maturation pools, so neutrophilia with a left shift is characteristic of an active need for neutrophils. The marrow responds to inflammatory cytokines released into the blood by replenishing the storage and maturation pools from the stem cell and proliferation pools, resulting in a chronic or compensated inflammatory leukogram characterized by a mature neutrophilia. Inflammatory mediators stimulate neutropoiesis and subsequent granulocytic hyperplasia in the bone marrow. The total 7–9 days neutrophil transit time in health decreases to 3–4 days with inflammatory cytokine stimulation. When there is peracute, severe inflammation, neutropenia may occur as marked tissue demand depletes the storage pool before enhanced neutropoiesis can replenish the storage and maturation pools. A simplified depiction of the growth factors responsible for stimulation of neutrophil production and release is presented in Figure 2.3. Figure 2.3 Schematic diagram of bone marrow and blood neutrophil pools. Inflammatory mediators released into the blood stimulate the marrow to produce neutrophils via an increase in growth factors and cytokines, mainly colony‐stimulating factors (CSF) and interleukins (IL). G‐CSF, GM‐CSF, and IL‐1, IL‐3, and IL‐6 are the most prominent in neutropoiesis. Inflammatory mediators and cytokines such as tumor necrosis factors (TNF), IL‐1, and CSF increase neutrophil release from marrow sinuses and migration from blood into tissue. The most mature neutrophil forms preferentially leave the marrow; these forms also preferentially migrate into tissue. The equine neutrophil storage pool is intermediate in size compared to the canine and bovine pools, which have the largest and smallest pools, respectively. Horses may have little to no neutrophilia or left shift during inflammation. Inflammatory neutrophilias in horses only occasionally exceed 20,000/μL and it is uncommon to see neutrophilias greater than 30,000/μL. Monocytosis may be a feature of both acute and compensated (chronic) inflammation and generally reflects a need for macrophages. Thus, inflammatory processes that elicit histiocytic responses are associated with monocytosis. Endogenous and exogenous glucocorticoids produce a characteristic leukogram pattern consisting of a mature neutrophilia and lymphopenia. Unlike dogs and cats, horses do not have monocytosis as part of the glucocorticoid response. The neutrophilia is caused by release of marginated neutrophils into circulation. The ratio of marginated to circulating neutrophils in horses is 1:1, thus the maximum increase in neutrophil concentration due to demargination does not exceed twofold. Lymphopenia is considered the hallmark of the glucocorticoid response and is attributed to margination and emigration of lymphocytes to tissues and lymph nodes; chronic glucocorticoid effects include lymphoid hypoplasia, which contributes to the lymphopenia.
2
Equine Hematology
2.1 CBC Interpretation
2.1.1 The Erythrogram
2.1.1.1 Erythrocytosis
2.1.1.2 Anemia
2.1.1.2.1 Regenerative Anemia
2.1.1.2.2 Nonregenerative Anemia
2.1.1.3 Changes in Erythrocyte Indices in Response to Anemia
cssStyle=”font-weight:bold;font-style:italic;” 2.1.1.3.1 Mean Cell Volume
cssStyle=”font-weight:bold;font-style:italic;” 2.1.1.3.2 RDW and the Distribution Histogram
cssStyle=”font-weight:bold;font-style:italic;” 2.1.1.3.3 MCH and MCHC
2.1.1.4 Age and Breed Effects on RBC Parameters
2.1.1.5 Splenic Effects
2.1.2 The Leukogram
2.1.2.1 Leukogram Patterns
cssStyle=”font-weight:bold;font-style:italic;” 2.1.2.1.1 Inflammation
cssStyle=”font-weight:bold;font-style:italic;” 2.1.2.1.2 Corticosteroid Response
cssStyle=”font-weight:bold;font-style:italic;” 2.1.2.1.3 Physiological (Catecholamine) Response
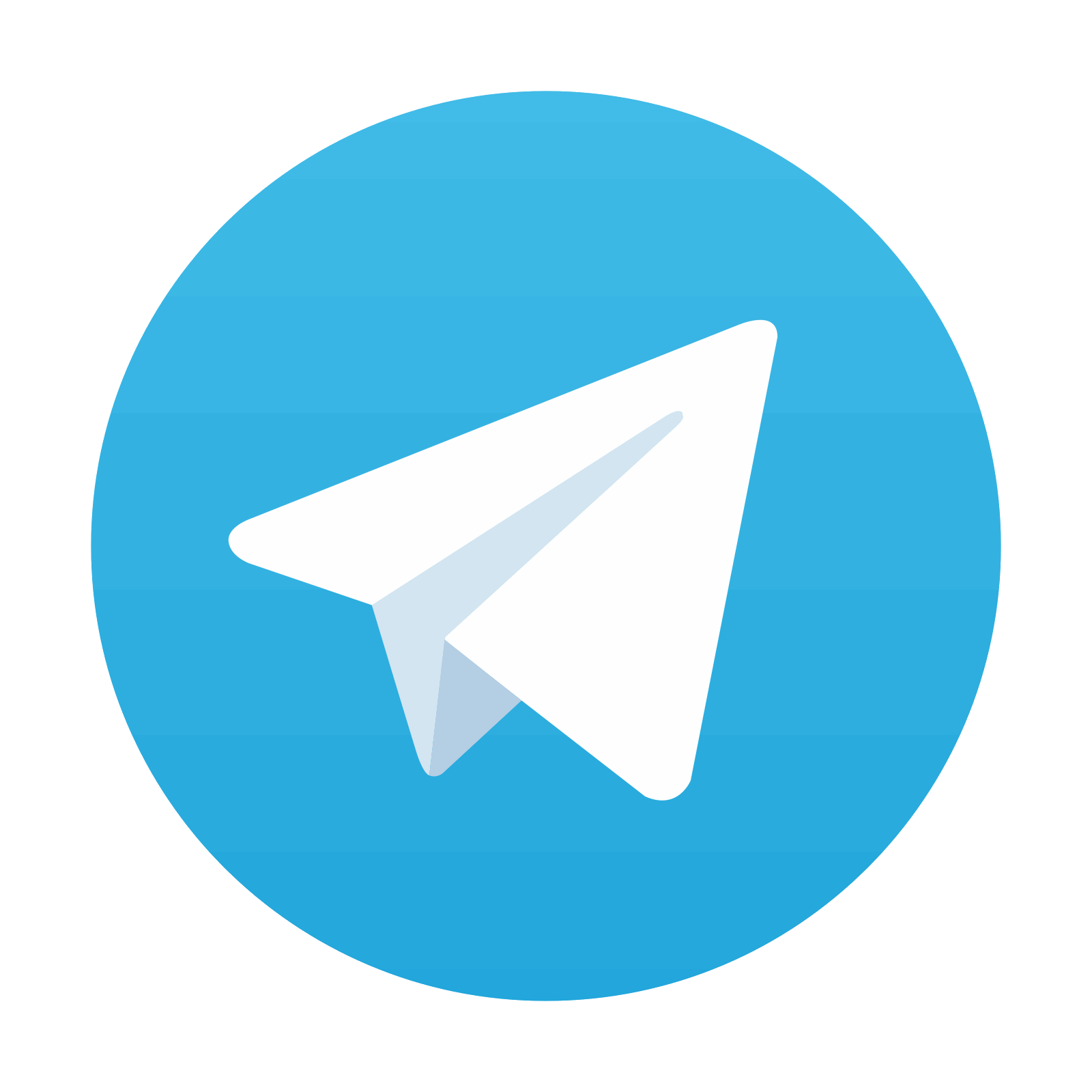
Stay updated, free articles. Join our Telegram channel

Full access? Get Clinical Tree
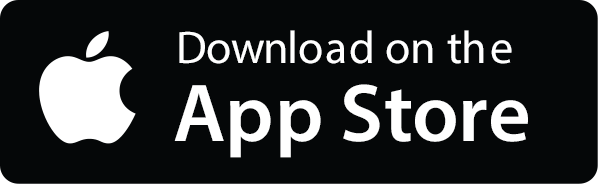
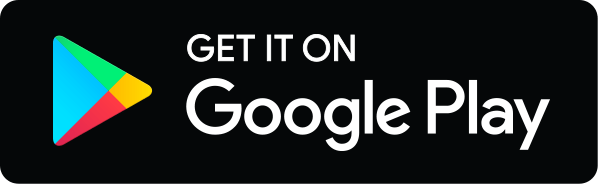