OURANIA M. ANDRISANI
The endocrine system is the ductless gland system (Greek endo, meaning “within”; Greek krino, meaning “to separate”), that is, the glands of internal secretion. Endocrine secretions are released into the intercellular compartment rather than onto a surface or into a duct leading to a surface (as is the case with exocrine glands). Most organs and tissues of the endocrine system are characterized by a dense epithelioid parenchyma and a sparse, delicate, interstitial stroma. The reticular fiber stroma supports individual parenchymal cells and conveys blood and lymph capillaries and the distal arborizations of neurons. Endocrine cells are typically nonpolar, releasing their secretory products, hormones, into the interstitial space from the entire cell surface. Hormones may diffuse locally in the interstitial fluid, binding to their specific cellular receptors, signaling adjacent cells in an autocrine or paracrine mode. Alternatively, they may enter the blood or lymph capillaries, signaling target cells located at a greater distance. Circulating hormones are diluted by interstitial fluid and plasma to relatively low concentrations (10–9 to 10–11M) and bind to their receptors with high affinity. The endocrine system plays a major role in the maintenance of homeostasis, the dynamic steady state of the body, ensuring minimum variability of the organism’s internal environment. Hormones modulate target cell function by up-regulating (stimulating) or down-regulating (inhibiting) processes in a dynamic fashion. Functions of the endocrine system are integrated and regulated by complex neural, endocrine, and immune molecular signaling and feedback mechanisms.
The endocrine system is composed of endocrine organs, as well as tissues and cells found in small numbers in nonendocrine organs. Classically, the endocrine organs include the hypophysis cerebri (pituitary gland), epiphysis cerebri (pineal gland), thyroid gland(s), parathyroid glands, and adrenal glands. Endocrine tissues typically have well-differentiated epithelial/epithelioid cells. The organelles and inclusions are indicative of secretory activity, and the cells have an intimate association with large, highly permeable blood capillaries (sinusoids) as well as lymphatics. In addition to dense vascularity, other characteristics of endocrine tissue include sparse stroma, high metabolic activity of the parenchyma, and a potent ability to stimulate angiogenesis. Interestingly, endocrine tissues are found in organs having additional, nonendocrine functions, for example, neurosecretory neurons of the hypothalamus; pancreatic islet cells; adipocytes; cardiac myocytes; juxtaglomerular cells of the kidney; internal theca cells, follicular epitheliocytes, interstitial cells, and corpus luteal cells of the ovary; hormone-producing cells of the placenta; interstitial and sustentacular cells of the testis; and paraganglia associated with blood vessels. Relatively isolated endocrine cells are dispersed in the epithelium of the digestive, urinary, reproductive, and respiratory systems; together these cells constitute the diffuse neuroendocrine system.
All embryonic germ layers contribute parenchyma to endocrine organs and tissues. For example, ectoderm gives rise to hypothalamic nuclei, the hypophysis cerebri, the epiphysis cerebri, and the neural crest, the latter providing precursor cells to multiple endocrine cells and tissues; differentiation of mesoderm forms the adrenal cortex and endocrine tissues of the ovary and testis; and endoderm contributes the parenchyma of the thyroid and parathyroid.
ENDOCRINE ORGANS
Hypothalamus
The hypothalamus is the most ventral part of the diencephalon and forms a significant portion of the expansive circumventricular region (Fig. 15-1). The hypothalamus contains neurons that integrate and regulate vital body functions (e.g., temperature, blood volume, blood osmolarity, and food intake). Clusters of neurons of the hypothalamus are referred to as nuclei, including the paired supraoptic, paraventricular, and arcuate nuclei. Afferent neural input to the hypothalamic neurons comes from many regions of the brain, spinal cord, and special senses. The hypothalamic neurons receive signals that reflect the status of the internal and external environments of the body, and respond with signals to the endocrine system and the autonomic portion of the nervous system. In the circumventricular region, the blood-brain barrier is reduced or absent, allowing hypothalamic neurons to easily respond to ionic and molecular signals (importantly, hormonal signals) in the blood. This combined input from the nervous system and the vascular system provides the basis for both a hormonal feedback system and neuroendocrine integration.
Peptidergic neurons of the hypothalamus (e.g., neurons of the supraoptic, suprachiasmatic, paraventricular, or arcuate nuclei, which have peptides as neurotransmitters) contribute axons to the neurohypophysis and secrete releasing hormones (releasing factors) to the adenohypophysis (Table 15-1). These axons projecting from the hypothalamus constitute the hypothalmo-neurohypophyseal tract described below. Releasing hormones are distributed to the hypophysis cerebri through a blood circulation system (hypothalamo-hypophyseal portal system) described below.
FIGURE 15-1 Schematic drawing of the hypothalamo-adenohypophysial and the hypothalmo-neurohypophysial systems. Optic chiasm (O); rostral commissure (RC). Axons from the parvicellular neurons (PN) of the hypothalamus terminate in contact with a primary capillary plexus (PCP) of the hypophysial portal system in the external zone of the median eminence, into which they secrete releasing hormones; in the case of prolactin, dopamine-producing neurons of the hypothalamus inhibit its release from the lactotrophs. These hormones are conveyed to the pars distalis (PD) through the hypophysial portal venules (HPV) and gain access to the cells of the pars distalis via a secondary capillary plexus (SCP). Pars intermedia (PI); pars tuberalis (PT). Axons originating from the magnocellular hypothalamic supraoptic (SO) and paraventricular (PV) nuclei course through the median eminence (ME) and the infundibular stalk (IS) to terminate in the neural lobe (NL), where oxytocin and vasopressin are stored and released.

Hypophysis Cerebri (Pituitary Gland)
Adenohypophysis
The hypophysis cerebri is composed of the adenohypophysis and neurohypophysis (in humans, the anterior lobe and posterior lobe, respectively). During development, the adenohypophysis forms from a midline epithelial evagination (Rathke’s pouch) of the roof of the stomodeum, eventually losing the stomodeal connection. Simultaneously, the neurohypophysis forms as a midline evagination of neural tissue from the floor of the hypothalamus (diencephalon). As the oral epithelial pouch advances, it surrounds the neural evagination, and forms a double-layered envelope. Together these primordia form the hypophysis cerebri, with the neurohypophysis remaining attached to the developing hypothalamus (Fig. 15-2A). This organogenesis brings the adenohypophysis near the hypothalamus, creating a structural proximity that is important for the hypothalamic regulation of hypophyseal function.
The adenohypophyseal (Latin adeno, meaning “gland”; Greek hypo, meaning “beneath”; Greek physis, meaning “growth”) parenchyma differentiates, forming three parts: pars distalis, pars intermedia, and pars tuberalis. The relative size and orientation of each part depends upon the species (Fig. 15-3). As the pouch contacts and envelops all but the distal aspect of the developing neurohypophysis, those cells contacting the neural tissue form the pars intermedia (Fig. 15-2B and D). The pars distalis forms the most diverse cell population and largest part of the adenohy-pophysis (Fig. 15-2B and E), and constitutes the majority of the outer envelope formed by the embryonic stomodeal pouch. The pars tuberalis is the portion of the pouch that contacts the base of the brain (median eminence/tuber cinereum), forming a collarlike investment of the stalk of the neurohypophysis (Fig. 15-2B), and links the pars distalis and pars intermedia proximally. The lumen of the pouch may remain in some species/individuals as the hypophyseal cavity between the pars intermedia and the pars distalis (Fig. 15-2B and D).
TABLE 15-1 Relationship of Hypothalamic Releasing Hormones, Hormones Released From Adenohypophysis, and Hormones Released by Target Cells

FIGURE 15-2 A. Midsagittal (median) section of the hypophysis cerebri, hypothalamus, and thalamus of a puppy (×2). B. Blood arrives in sinusoids of pars distalis only after perfusing the hypothalamus; a large cyst is present in the pars distalis (×4.5). C. Portion of the hypothalamo-neurohypophyseal tract (×100). D. Adenohypophysis contacting neural lobe (×40). E. Parenchyma of the adenohypophysis (×100). Hematoxylin and eosin.

As an extension of the ventral aspect of the hypothalamus, the neurohypophysis is a continuation of the median eminence. Proximally, the third ventricle extends as a recess into the neurohypophysis (Fig. 15-2A and B). The proximal segment of the neurohypophysis is a funnel-shaped stalk, the infundibulum, which attaches to the median eminence. The infundibulum is continued distally as the neural lobe (pars nervosa) (Fig. 15-2B).
The hypophysis is oriented ventrocaudally from the hypothalamus, straddling the midline (Fig. 15-2B). Like the brain, the hypophysis is invested by the pia-arachnoid; distally, these leptomeninges are fused with the periosteum in a depression of the basisphenoid bone, the sella turcica (Latin sella, meaning “saddle”; Latin turcica, meaning “Turkish”). In species in which this depression is deep, the meninges, including the dura mater, extend as a diaphragm (the diaphragma sellae) from the lateral margins of the saddle at the level of the infundibular stalk. Due to its cryptic location, the common surgical approach to the hypophysis is transpharyngeal. At necropsy, meningeal adhesions with the periosteum (and the diaphragm in some species) make it difficult to remove the hypophysis intact and still attached to the brain.
Pars Distalis
The pars distalis comprises parenchymal cell clusters perfused with sinusoidal capillaries (Fig. 15-2E). The sinusoidal capillaries of the pars distalis form the secondary capillary plexus of the hypothalamo-hypophyseal portal system. Based on routine staining of their stored secretions, the cells of the pars distalis have been classified as acidophils, basophils, or chromophobes. These cell types vary in size, shape, number, and position depending on species, gender, age, and physiologic status (e.g., during pregnancy and lactation or after gonad removal). Five subtypes of cells have been further identified with immunohistochemistry. Each cell type (called a “-troph”) expresses a peptide, protein, or glycoprotein hormone (called a tropin [Greek troph, meaning “food”]) (Fig. 15-2E) and represents a definitive and terminal differentiation. Secretory vesicles store the secretions in the cytoplasm prior to signals for their exocytosis (Fig. 15-4). Secretions include somatotropin hormone (growth hormone); lactotropin (mammotropin, prolactin); thyrotropin (thyroid-stimulating hormone); two gonadotropins (follicle-stimulating hormone and luteinizing hormone); and corticotropin (adrenocorticotropic hormone) (see Table 15-1).
FIGURE 15-3 Schematic drawings of midsagittal sections of the hypophysis cerebri. A. Horse. B. Cow. C. Dog. D. Pig. E. Cat. F. Sheep. White: neurohypophysis with infundibular recess; small dots: pars tuberalis adenohypophysis; large dots: pars distalis adenohypophysis; black: pars intermedia adenohypophysis. (From Dellmann H-D. Veterinary Histology: An Outline Text–Atlas. Philadelphia: Lea & Febiger, 1971.)

Acidophils. Specifically, somatotrophs that synthesize and secrete growth hormone (GH) are concentrated laterally in the pars distalis; their abundant acidophilic secretory granules stain with orange G dye and are approximately 300 to 400 nm in diameter. Lactotrophs, which produce prolactin (PRL), stain lightly with erythrosin and carmine dyes. Lactotroph cell size and dye affinity increase during pregnancy and lactation. The acidophilic secretory granules of lactotrophs may be as large as approximately 800 nm in diameter.
Basophils. Thyrotrophs produce thyroid-stimulating hormone (TSH), are more numerous midventrally, and may be detected with aldehyde-fuchsin dye; their basophilic secretory granules measure approximately 150 nm in diameter. Gonadotrophs coexpress follicle-stimulating hormone (FSH) and luteinizing hormone (LH), are relatively small, and stain with aldehyde-thionine dyes; their stored basophilic secretory granules are approximately 200 nm in diameter. Corticotrophs produce adrenocorticotropic hormone (ACTH) and are uniformly dispersed in the pars distalis. These cells are less conspicuous within the parenchymal cell clusters and are difficult to identify with the light microscope. Corticotrophs may be spherical, ovoid, or stellate, depending on the species. Their basophilic granules measure approximately 150 to 200 nm in diameter and stain with antibody for both ACTH and (β-lipotropin hormone (β-LPH). These peptide hormones are derived from the posttranslational processing of proopiomelanocortin (POMC). All three basophilic cell types stain with periodic acid-Schiff (PAS) and Alcian blue dyes.
Chromophobes. Chromophobes stain poorly with dyes used to identify acidophils and basophils. Some are considered postsecretory acidophils and basophils. Other chromophobes occasionally form the simple epithelium that lines cysts of unknown significance. Still other stellate-shaped chromophobes are interspersed between the other cells of the pars distalis, and it has been suggested that they may represent an undifferentiated stem cell of the adenohypophyseal parenchyma.
Pars Intermedia
In some species, the parenchyma of the pars intermedia is arranged as a simple columnar epithelium; in others, it is a pseudo-stratified columnar epithelium that invades the neural lobe; while in others (e.g., horse), the parenchyma is extensively developed. Melanotrophs are the most abundant parenchymal cell of the pars intermedia, secreting α-melanocyte-stimulating hormone (α-MSH) and β-LPH. These peptide hormones are processed products of POMC expressed by the melanotrophs. Other parenchymal cells include those lining cysts, corticotrophs, stellate cells, and simple epithelium lining the hypophyseal cavity. Blood capillaries are not as extensive as in the pars distalis. Hypothalamic axons terminate in the pars intermedia and include dopaminergic, serotoninergic, adrenergic, and GABAergic (γ-aminobutyric acid) axons that modulate the activity of the parenchyma.
FIGURE 15-4 Organelles typical of an adenohypophyseal cell: granular (rough) endoplasmic reticulum (rER); Golgi complex (Go); mitochondria (M); secretory granules (SG) of varying maturational stages (×28,000).

Pars Tuberalis
The pars tuberalis is composed of cell clusters that form a folded tissue with occasional small cysts (Figs. 15-2B and 15-5). This region of the pituitary is traversed by hypophyseal portal venules that carry blood from the primary capillary plexus in the median eminence to the secondary capillary plexus in the pars distalis. The parenchymal cells of the pars tuberalis have melatonin receptors and are believed to regulate the seasonal reproductive cycle of some domesticated mammals (see Epiphysis Cerebri below). Other cells include those lining cysts, as well as a few Gonadotrophs and thyrotrophs.
Neurohypophysis
Hypothalamo-Neurohypophyseal Tract
Large (magnocellular) neurons clustered in the hypothalamus make up the majority of the supraoptic and paraventricular nuclei. These neurons receive afferent synaptic input that signals the exocytosis of secretory vesicles from their axons. Bundles of these axons and their supporting astroglial cells constitute the median eminence, infundibulum, and neural lobe of the neurohypophysis (Fig. 15-2B), together forming a major portion of a tractlike extension of the hypothalamus known as the hypothalamo-neurohypophyseal tract. The neurohypophysis contains the axons of hypothalamic neurons and central gliocytes (“pituicytes”), but no neuron cell bodies. The neuron cell bodies in the hypothalamus and secretory granules in their axons stain positively with antibody to oxytocin (OT) and antidiuretic hormone (ADH) (Figs. 15-6 and 15-7), also called arginine vasopressin (AVP). In the pig, lysine is substituted for arginine, producing lysine vasopressin (LVP). ADH-producing neurons and OT-producing neurons are present in both the supraoptic nuclei and the para-ventricular nuclei. Secretory vesicles containing the hormone are transported along microtubules to axon terminals near blood capillaries in the neural lobe. Along the course of the axon, secretory vesicles form focal accumulations (Herring bodies); when stained with aldehyde-fuchsin, these can be resolved by light microscopy (Fig. 15-8).
In the hypothalamic nuclei, neurons synthesize preprooxytocin, which is processed to oxytocin and neurophysin and packaged in secretory granules. Exocytosis of the secretory granules is via a calcium-mediated mechanism, initiated by a neural synapse from sensory networks in the brainstem (e.g., sensory input from washing the cow’s udder in the milking parlor or sounds from the sow’s litter). When released, oxytocin binds to its receptor on myoepithelial cells of the mammary alveoli and ducts, causing myofilament-driven cell contraction, thereby resulting in “milk letdown.” In addition, oxytocin binds to its receptor on smooth myocytes of the myometrium, causing uterine contraction and parturition, and to its receptor in the brain, initiating species-specific maternal behaviors (e.g., sheep).
FIGURE 15-5 Section in the dorsal plane through the median eminence (large ruminant). Infundibular recess (A); internal zone (B); external zone (C); pars tuberalis of the adenohypophysis with numerous portal venules (D). Masson’s trichrome (×2).

FIGURE 15-6 Neurosecretory neurons of hypothalamic paraventricular nucleus labeled with antibodies directed against antidiuretic hormone. Transverse section (×20).

FIGURE 15-7 Axons of neurosecretory neurons (A) adjacent to a basal lamina, interstitial connective tissue (B), and a fenestrated capillary (C) in the neural lobe of neurohypophysis. Axon terminals have large electrondense, hormone-containing secretory vesicles; upon depolarization of the neuron, the contents of the vesicles are released by exocytosis (×31,500).

FIGURE 15-8 Hypophysis cerebri of cat. Dark staining within the neural lobe (NL) marks accumulations of neurosecretory granules (Herring bodies). Pars intermedia of the adenohypophysis (PI). Aldehyde-fuchsin (×40).

Other neurons in the hypothalamus synthesize preprovaso-pressin, which is processed to ADH and neurophysin. Secretory granules for ADH are stored and released similar to oxytocin granules. The release is signaled by sensory synapses from neurons monitoring properties of the internal environment, for example, increased plasma osmolality or decreased blood volume (hypovolemia). ADH acts on the kidney by binding to the V2 receptor and activating water channels (aquaporin 2) in the apical membrane of the collecting tubule cells, decreasing water loss through the urine (an antidiuretic effect). ADH also binds to its V1 receptor on vascular smooth muscle, causing increased myocyte tone, vascular constriction, and elevated blood pressure. In addition, binding of ADH to the V3 receptor on hypophyseal corticotrophs causes release of ACTH and a rise in aldosterone secretion. Insufficient production of ADH results in diabetes insipidus, characterized by an increased volume of dilute urine.
Hypothalamo-Adenohypophyseal Axis
Neurons of the hypothalamus also regulate the neuroendocrine and autonomic nervous systems. Within the hypothalamus, a generally diffuse population of small (parvicellular) neurons syn thesizes hormones, which target the cells of the pars distalis. These hormones are termed releasing hormones (factors); they effect the release, by exocytosis, of the specific hormones expressed and synthesized in the pars distalis. In contrast, functions of the pars intermedia are regulated by innervation, whereas regulation of cells in the pars tuberalis remains unclear.
The releasing hormones from the hypothalamus are transported within axons of hypothalamic neurons to the periphery of the median eminence, where they are released near the primary capillary plexus of the hypothalamo-hypophyseal portal system (Greek portal, meaning “gateway”). The capillaries are drained by hypophyseal venules that flow through the pars tuberalis to the secondary capillary plexus in the pars distalis. This portal vascular system establishes the anatomic basis of a functional hypothalamo-adenohypophyseal axis.
In the pars distalis, the hypothalamic neurosecretions leave the secondary capillary (sinusoidal) plexus and bind specific receptors on their respective adenohypophyseal target cells. Hormones are then released from the target cells and affect other target cells in the periphery. In turn, peripheral target cells synthesize and release hormones into the circulation that mediate either negative feedback in other target cells or positive feedback to the hypothalamic neurons and cells of the pars distalis.
Growth hormone–releasing hormone (GHRH) (somato-tropin-releasing hormone [SRH]) is released from neurons of the arcuate nucleus in response to neural stimulation (e.g., during sleep and exercise). It binds to its receptor on the somatotrophs of the pars distalis. The GHRH receptor is a G protein–coupled receptor (GPCR) that, when activated, elevates intracellular concentration of 3′, 5′-cyclic adenosine monophosphate (cAMP), triggering calcium-mediated exocytosis of secretory vesicles containing growth hormone. Growth hormone (GH) is a 22 Kda protein. The GH receptor is a tyrosine kinase (cytokine receptor family) that, upon binding GH, forms a dimer, leading to tyrosine phosphorylation of downstream signaling molecules (transcription factors). GH targets all cells, but especially hepatocytes, skeletal myocytes, adipocytes, and growth plate chondrocytes. GH induces an anabolic effect in muscle; in liver and cartilage, GH induces synthesis and release of insulinlike growth factor I (IGF-I; somatomedin). IGF-I mediates additional GH effects by acting on chondroblasts, promoting their proliferation and thus causing growth of the skeleton. GH causes its own down-regulation (negative feedback) by stimulating somatostatin-producing neurons in the hypothalamus. Somatostatin binds to its GPCR on the GH-producing somatotrophs, uncoupling cAMP production and suppressing release of GH.
Prolactin-releasing factor (PRF) remains poorly understood. Prolactin (PRL) is principally regulated by tonic inhibition provided by dopamine from the hypothalamus. Dopamine binds to its D2 membrane receptor on lactotrophs of the pars distalis, uncoupling cAMP, lowering intracellular calcium, and inhibiting release of prolactin. Several stimuli result in PRL release from the lactotrophs (e.g., TRH, OT, ADH, angiotensin II). Neurogenic stress and sensory stimuli of suckling can override the dopamine inhibition. PRL is a 23 Kda protein; the PRL receptor is a tyrosine kinase. PRL targets its receptor on epithelial cells of the mammary gland, causing tyrosine phosphorylation of signaling molecules that stimulate proliferation and differentiation of the epitheliocytes as milk-synthesizing cells. Further, PRL binds to its receptor on dopaminergic neurons of the hypothalamus, increasing dopamine synthesis and release, thus causing inhibition of PRL release in the pars distalis. Together with GH, PRL also stimulates the immune system, specifically the proliferation and differentiation of lymphocytes.
Thyrotropin-releasing hormone (TRH) is released from small neurons in the paraventricular nucleus and binds to its GPCR on thyrotrophs (see Fig. 15-13). The binding results in the activation of phospholipase C and PI3-kinase and the elevation of intracellular calcium concentration, which is involved in exocytosis of thyroid-stimulating hormone (TSH; thyrotropin). TSH is a glycoprotein that is transported in the blood to the thyroid epithelial cells where it binds to its GPCR, stimulating the synthesis and storage of thyroglobulin and the release of thyroid hormones, triiodothyronine (T3) or tetraiodothyronine (T4). In turn, negative feedback of T3 or T4 to the hypothalamus and the pars distalis regulates the production of TRH and TSH, respectively.
Gonadotropin-releasing hormone (GnRH) is a protein hormone released from neurons diffusely scattered in the hypothalamus; it binds to its GPCR on the gonadotroph, elevating the intracellular concentration of calcium and activating protein kinase C. Protein kinase C signals the coexpression, synthesis, and release of the glycoprotein hormones follicle-stimulating hormone (FSH) and luteinizing hormone (LH). In the female, FSH binds to its GPCR on the follicular epithelial cells; in the male, FSH binds to its GPCR on sustentacular cells (Sertoli cells) of the testis. In both sexes, production of estrogen is signaled by FSH. In the female, LH binds to its GPCR on internal thecal cells, signaling the production of testosterone, and on follicular and thecal cells of the postovulatory follicle, signaling production of progesterone. Cells of the ovarian interstitial stroma in the pig, dog, cat, rabbit, and human also produce testosterone. In the male, LH (also called interstitial cell–stimulating hormone [ICSH]) binds to its GPCR on the interstitial cells (Leydig cells) and signals production of testosterone. In addition to effects on other target cells, these gonadal steroids bind to cells of the hypothalamus and the pars distalis, negatively regulating production of GnRH and gonadotropins. FSH also stimulates follicular cells, corpus luteal cells, and sustentacular cells to synthesize inhibin and activin. As members of the TGF-β superfamily of growth factors, inhibin and activin either inhibit or activate, respectively, production of FSH from the hypophysis.
Corticotropin-releasing hormone (CRH) is a protein hormone released from small neurons in the paraventricular nucleus in response to neural signaling (see Fig. 15-20). CRH binds to its GPCR on the corticotrophs, elevating intracellular concentration of cAMP and triggering calcium-mediated exocytosis of secretory vesicles containing adrenocorticotropic hormone (ACTH). ADH potentiates the release of ACTH from corticotrophs; ADH and CRH are coexpressed in neurons of the paraventricular nucleus that terminate in the median eminence, near the primary capillary plexus of the portal system. IL-1β, IL-6, and TNF-α act in the hypothalamus to enhance CRH production. ACTH is one of the posttranslational cleavage products of the prohormone POMC, processed in corticotrophs to form ACTH, α-MSH, and β-LPH. ACTH binds to its GPCR on adrenal cortical parenchymal cells and, via adenylate cyclase and cAMP, activates proteins that increase transcription of the enzymes involved in steroid hormone biosynthesis (e.g., glucocorticoids). Glucocorticoids negatively regulate CRH production in the hypothalamus and negatively regulate POMC synthesis and ACTH release in the pars distalis. In response to stress, signals creating increased neurogenic input to the hypothalamus overcome this inhibition of ACTH by glucocorticoid.
Epiphysis Cerebri (Pineal Gland)
The pineal gland is a midline evagination of the dorsocaudal aspect of the epithalamus of the diencephalon, to which it remains attached by a stalk that contains a small recess of ventricle III (Fig. 15-9A). The pineal (Latin pinus, meaning “pine, pine cone”) is enveloped by pia-arachnoid from which the blood supply to the gland originates. As a derivative of neural epithelium, the parenchyma is composed of pinealocytes and astrocytelike, central gliocytes; the processes of both cell types form the neuropil between the cells (Fig. 15-9B). The blood-brain barrier is not functional in this circumventricular organ and pinealocyte secretions have easy access to blood capillaries; pineal secretions also appear in the cerebrospinal fluid. In old animals, calcified protein deposits (concretions) termed corpora arenacea (brain sand) may be present. The deposits are easily resolvable in the intercellular neuropil, but are of no known functional significance.
Pinealocytes share gap junctions and extend axons to multiple sites, including other pinealocytes, adjacent capillaries (Fig. 15-9C), and the base of the ependymal lining of the ventricular recess. The pinealocyte receives synaptic input from sympathetic axons coursing in the adventitia of arterioles supplying the pineal. Sympathetic neurons from which these axons arise are in the cranial cervical ganglia. These neurons receive input from preganglionic neurons in the intermediate gray matter of the first thoracic segment of the spinal cord. The thoracic neurons are linked to optic input via a reticulospinal tract to the trigeminal reticular nucleus and, in turn, axonal projections from the suprachiasmatic nucleus of the hypothalamus. The optic nerve sends projections to synapses in the suprachiasmic nucleus.
Pineal function is linked to visual signals relaying information about environmental light. Most understanding of the mechanism of pineal function is based on data from the rat, sheep, and horse. A biorhythm, also known as circadian rhythm, has been linked to the intensity of yellow-green light in the environment and characterizes the secretory activity of the pinealocyte. The primary signal to the pinealocyte is norepinephrine, released from the sympathetic axon, which binds to its β-adrenergic receptor. Light received by an intact visual system inhibits this adrenergic stimulation, and the pinealocyte releases serotonin. In the dark phase, the adrenergic stimulation is operational, resulting in activation of the β-adrenergic GPCR on the pinealocyte. The resulting elevation of cAMP induces the expression of N-acetyltransferase, which converts serotonin to melatonin. Melatonin is released as it is synthesized, rather than stored. Melatonin, released from axons terminating near capillaries, circulates via the blood. At the target cells, melatonin binds to its GPCR, inhibiting neurons in the suprachiasmatic nucleus; it also inhibits gonadotropin release from the pars distalis by an unknown mechanism. In domesticated animals that are seasonally polyestrous, pineal activity resulting in melatonin synthesis is linked to species-dependent, seasonal (circannual) variation in ambient photoperiod, blocking release of gonadotropins and having an “antigonadotrophic effect.”
FIGURE 15-9 Midsagittal section, pineal and epithalamus of puppy. A: ×2; B: ×40; C: ×100. Saffron-modified Masson’s trichrome.

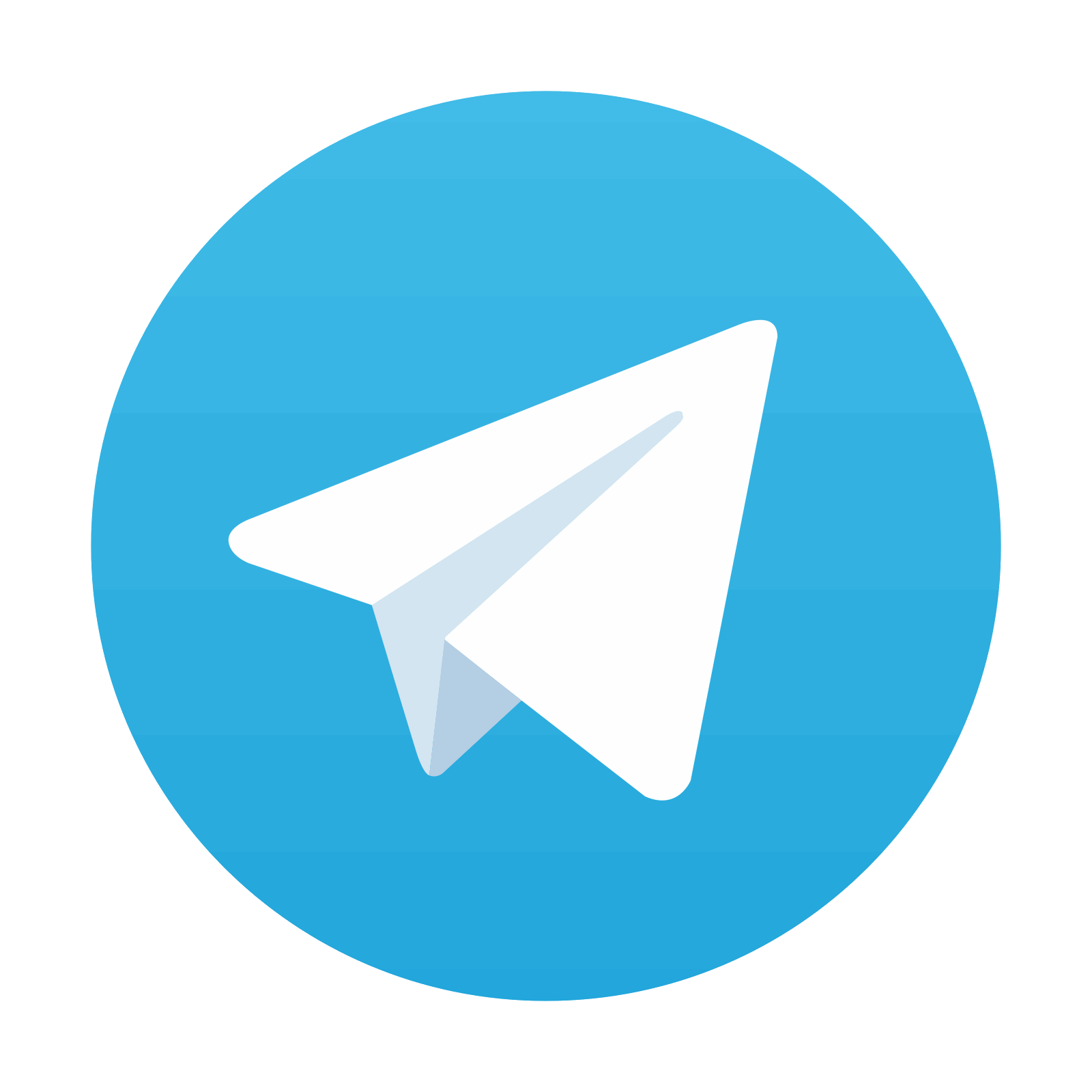
Stay updated, free articles. Join our Telegram channel

Full access? Get Clinical Tree
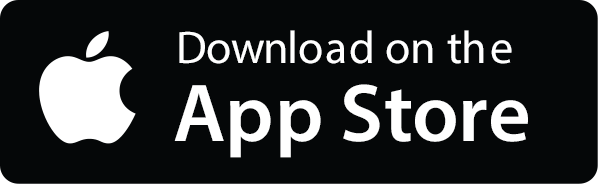
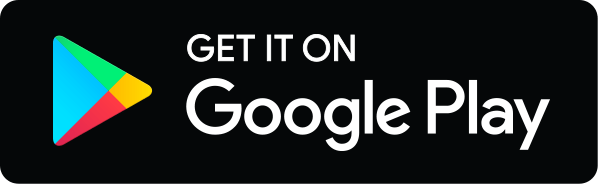