Kyle J. Bartholomew Department of Surgical Sciences, School of Veterinary Medicine, University of Wisconsin, Madison, Wisconsin, USA An electrocardiogram (ECG) is the graphical representation of the cardiac electrical activity recorded over time. The ECG allows the anesthetist to evaluate the heart rate and rhythm and, when evaluated in combination with blood pressure and other indicators of tissue perfusion and cardiac output, allows for an assessment of the cardiovascular status of the patient. Many disease states may lead to the development of arrhythmias (e.g., electrolyte disturbances, diseases of splanchnic viscera, paraneoplastic diseases, etc.), and some anesthetic drugs can increase the risk of spontaneous arrhythmias in otherwise healthy animals [1–4]. Due to the widespread availability of this technology, ECG monitoring should be considered for any patient undergoing anesthesia, even for short‐duration or routine procedures. A normal ECG is easily recognizable and represents the electrical activity of the heart. However, to understand why and how an abnormal rhythm may develop, it is important to understand the basic physics behind the ECG as well as the minor species differences. It is also important to understand the limitations of an ECG and how to differentiate common artifacts from true arrhythmias. There are many different systems available that can obtain an ECG; the underlying principles remain fairly constant between machines. Understanding the basics of electrocardiography requires integration of multiple concepts including generation of the cardiac action potential, the equivalent dipole theory, and the lead system. The cardiac action potential is covered in detail elsewhere (see Chapter 33). What follows is a brief description of the equivalent dipole theory, cardiac vectors, and the lead system. The equivalent dipole theory states that the heart can be approximated to a dipole (i.e., it is equivalent to a dipole when measured); the force that is generated has a direction and magnitude and can be represented as a vector [5,6]. A dipole is a pair of electrical charges of equal and opposite polarity that are separated by a distance. The electrical potential generated can be measured with a galvanometer, which consists of two exploring electrodes placed on each side of the system. The electrical potential measured by the galvanometer is dependent on the intensity of the electrical force generated, the distance the electrodes from the dipole, and the position of the electrodes relative to the orientation of the dipole. Depolarization of the myocardium generates electrical forces of varying magnitudes and directions; this electrical activity can be thought of as vectors of electrical activation. Electrical activity occurring simultaneously creates a summation vector. The magnitude of these vectors is detected on the body surface, depending on the site of the exploring electrode and the direction of the vector. The electrode placed on a line parallel to the direction of the vector will be able to record the maximum amplitude of that activity [6]. When several exploring electrodes are attached on the surface of the patient, it is possible to record the magnitude and direction of these cardiac vectors. The ECG records the electric potential (in mV, on the y axis) over time (in seconds, on the x axis) between a point on the body surface and an indifferent (or reference) electrode or between two recording electrodes. The lines joining these electrodes form what we refer to as “leads” along which the cardiac vectors are measured [6]. Leads may be defined as unipolar or bipolar. If the potential is recorded between an electrode on the body surface (exploring electrode) and an indifferent (or reference) electrode, the leads are unipolar. If the difference is recorded between two recording electrodes, the leads are bipolar. The voltage change recorded by the leads is displayed graphically. Each electrical wave is defined by its amplitude, polarity, and duration. When the cardiac depolarization vector is parallel to the lead, the wave is of maximum amplitude; a depolarization vector perpendicular to the lead produces a wave of minimal amplitude. Typically, a vector directed toward the positive electrode is represented as a positive wave and a vector directed toward the negative electrode is represented as a negative wave. Due to the directionality of an ECG, the direction of electrical activity can be “seen” from different directions depending on the leads used. A diagnostic ECG may be capable of up to 12 viewpoints in two planes: frontal and transverse. Clinically, the frontal plane is most commonly used. Electrodes placed on the right forelimb, left forelimb, and left hindlimb make up an arrangement known as “Einthoven’s triangle,” named after Willem Einthoven, a Dutch doctor who is credited with developing the first apparatus utilized to detect electrical activity of the heart. The three potential differences created by this arrangement are called leads I, II, and III (Fig. 11.1). It has been suggested that this naming convention is largely historical, based on Einthoven’s original description, rather than any intrinsic meaning [7]. Figure 11.1 A. Einthoven’s Triangle, the basic schematic for the modern bipolar lead system. B. Standard bipolar lead placement in a dog, used on most small domestic species; the white lead (RA) is placed on the right forelimb, the black lead (LA) is placed on the left forelimb, and the red lead (LL) is placed on the left hindlimb. Source: Dr. Kyle Bartholomew, with permission. Leads I, II, and III are referred to as the “standard bipolar leads” because they measure between two points of the electrodes (Fig. 11.1). Lead I measures the potential difference between the right and left forelimb with the left considered positive. Lead II, the most common lead used clinically, is between the right forelimb and left hindlimb with the hindlimb considered positive. Lead III is between the left forelimb and left hindlimb with the hindlimb considered positive [7]. As bipolar leads (and lead II specifically) are the primary leads utilized in perianesthetic monitoring, they will be the focus of the rest of the chapter; however, the other diagnostic leads are briefly discussed below. Because a lead is, by nature, the potential difference between two points, the theoretical number of leads that could exist is nearly limitless. Cardiologists commonly use other lead systems, such as the modified orthogonal lead system, and the descriptions provided below should by no means be considered exhaustive, only a summary of common configurations that may be used in the perianesthetic period [6]. The unipolar leads are created by comparing the three electrodes to a reference voltage created by the monitor, known as “Wilson’s central terminal.” The signal between the three electrodes and the reference (Wilson’s central terminal) are known as aVL (left forelimb), aVR (right forelimb), and aVF (left hindlimb). These are also known as the “augmented limb leads,” and they allow for a slightly different angle of evaluation in the frontal plane [7]. The unipolar precordial leads are based on a similar concept: six additional electrodes can be placed on the thorax near the heart and are compared to a reference electrode (Wilson’s central terminal). These leads (V1–V6) provide information in the transverse plane rather than the frontal plane like other leads. The precordial system is used with some frequency in human patients; however, it is less commonly used in veterinary medicine during the perianesthetic period. In small animals, a multilead ECG may be used to generate targeted information about chamber enlargement, and lead II is used predominantly to monitor patients intraoperatively. In horses, however, it has been suggested that a multilead ECG is of less value because of the extensive branching of the Purkinje system in the ventricles. In these cases, a single‐lead system is typically sufficient to optimize the signal along the direction of the maximal deflection of the electrical impulse generated within the heart. To accomplish this, the electrodes are placed parallel to this impulse in what is often referred to as the “base‐apex lead” (Fig. 11.2) [8]. Although there is some debate as to whether the base‐apex lead is the most effective lead for diagnostic evaluation of the horse, it is currently the most well established [9]. It has also been suggested that the base‐apex lead arrangement accentuates the P wave of the equine ECG [10]. This arrangement historically uses the lead I setting with the negative (–) electrode (right forelimb/RA/white) placed over the jugular furrow or near the manubrium. The positive (+) electrode (left forelimb/LL/black) may be placed over the left apex of the heart, on the left side of the chest at the level of the olecranon or at the xiphoid. The ground electrode (left hindlimb/LL/red) may be attached to either side of the neck. Some portable ECG monitors utilize only two electrodes, which can be used to generate a single lead; the lead that is generated (I, II, or III) depends on where the electrodes are placed. Figure 11.2 Base‐apex lead arrangement in a horse: bipolar lead I is used with the right forelimb (RA/white) electrode placed over the jugular furrow, the left forelimb (LA/black) electrode placed on the left side of the chest just caudal to the forelimb, and ground electrode (left hindlimb/LL/red) is attached to either side of the neck. Source: Dr. Kyle Bartholomew, with permission. It is also important to note that many systems available in North America use a standard color‐coding system of right forelimb or RA (white), left forelimb or LA (black), and left hindlimb or LL (red). This color scheme is not always the same as that used internationally [11]. The ECG complex is a representation of the electrical activity occurring in the myocardium during a single cardiac cycle, from cell depolarization at the beginning of the cycle to the cell repolarization at the end of the cycle. Different terminology may be used by different sources to discuss these concepts, but terms used here are wave, segment, and interval. A “wave” represents an electrical event in the myocardium and is represented as a deflection above or below baseline. A segment is a line joining two waves, and an interval is the time (or graphical distance) between two events. The primary deflections in an ECG are the P wave, the Q, R, and S waves (often referred to as the “QRS complex”), and the T wave (Fig. 11.3) [12]. In a normal heart, the cardiac cycle begins at the sinoatrial (SA) node, a small group of cells located in the right atrium that act as the dominant pacemaker of the heart. This event is not represented by a wave on the ECG due to the small number of cells that make up the SA node and, therefore, the very small amount of voltage this event produces. However, immediately following SA node activation, a wave of depolarization travels across the atria facilitated by the atrial conduction system, including Bachmann’s bundle [13]. The depolarization of the atria is represented by the P wave on the ECG. As the wave of depolarization sweeps across the atria, it eventually reaches the atrioventricular (AV) node. The wave of depolarization propagates through the AV node and along the bundle of His before following the right and left bundle branches and, finally, through the Purkinje network on its way to the contractile cardiac myocytes. The time it takes for the wave of depolarization to propagate from the atria through the ventricular conduction system is represented by the PQ (also referred to as PR) interval and is defined as the time from the onset of the P wave to the onset of the QRS complex [12]. The extensive Purkinje network spreads the impulse to the contractile cardiac myocytes starting at the apex of the heart and begins the formation of the QRS complex (i.e., the three waves representing ventricular depolarization: Q, R, and S). It should be noted that most individuals refer to the complex that represents ventricular depolarization as the QRS, regardless of whether the complex contains a distinct Q, R, and S wave. Mechanical contraction of the myocardium follows electrical depolarization. After depolarization, repolarization of the ventricles follows to reestablish the cellular electrical potential that allows for the next electrical depolarization and mechanical contraction. The electrical repolarization of the ventricle is represented as the T wave on the ECG. The atria also experience repolarization which is sometimes represented as the Ta wave on the ECG; however, this wave normally occurs during the depolarization of the ventricles (i.e., the QRS complex) and, therefore, is obscured due to the larger electrical potential of ventricular depolarization. The ST segment, defined as the segment between the end of the QRS complex (sometimes referred to as the J point) and the T wave, corresponds to ventricular systole during which the membrane potential of ventricular myocytes is equal and is, therefore, normally represented as a horizontal line [12]. The J wave and U wave are two underappreciated waves that may represent early and late ventricular repolarization, respectively. In humans, the J wave may be appreciated in a normal heart but can also appear as result of hypothermia, hypercalcemia, or myocardial ischemia [14,15]. The U wave, theorized to originate from repolarization of the mid‐myocardial cells (M cells) or Purkinje cells, may be seen in patients who are bradycardic or suffer from hypokalemia [15]. Figure 11.3 An example of the classic ECG recording; the P wave represents atrial depolarization and the QRS complex represents ventricular depolarization. The T wave represents ventricular repolarization. The blue dotted structures are rarely appreciated, the U wave is theorized to originate from late repolarization of the mid‐myocardial cells or Purkinje cells and may be seen in patients who are bradycardic or suffering from hypokalemia; the Ta wave represents atrial repolarization and is rarely seen because its occurrence during the QRS complex obscures it. Source: Dr. Kyle Bartholomew, with permission. The line that occurs between the end of the T wave and the beginning of the next P wave is generally called “baseline” or the “isoelectric line” and is associated with the absence of electrical activity. This becomes important when considering the pauses that occur during a single cardiac depolarization and the time between the cardiac cycles. Evaluation of an ECG during anesthesia can give valuable information about the patient’s heart rate and can lead to early recognition of dysrhythmias that could become pathologic if left untreated. However, perianesthetic ECG interpretation may come with limitations, such as patient positioning, limited sites for electrode placement, and operating room artifacts. Therefore, ECG tracings recorded as part of continuous anesthetic monitoring may not meet all the recommendations for standardization associated with a fully diagnostic ECG. Regardless, there is still crucially important information that may be obtained from perianesthetic ECG monitoring. When evaluating the ECG, it is important to note that the horizontal axis of an ECG printout (also referred to as a strip or tracing) is time in seconds while the vertical axis is voltage, displayed in millivolts (mV). It is also important to note the underlying paper or sweep speed, the rate at which the ECG paper or screen travels while the ECG is being traced, commonly 25 mm/s or 50 mm/s. At a faster paper speed (50 mm/s), each small box (1 mm) on the horizontal axis represents 0.02 s and the ECG complex will appear wider. At a slower speed (25 mm/s), each small box represents 0.04 s, and the ECG complex will appear narrower comparatively. The ECG can be used as a source for determining the patient’s heart rate; however, it can be susceptible to artifact and interference, which may make automated heart rate calculation by the monitor less accurate. There are multiple methods available to manually calculate a heart rate from a printed ECG. The simplest, albeit least accurate, way to calculate the heart rate is to determine the number of R waves within 3 s and multiply by 20 to obtain beats per minute (Fig. 11.4) [16]. A more useful method, especially in patients with an irregular rhythm, is calculation of an “instantaneous” heart rate using only one R‐R interval, defined as the distance between two sequential R waves of the QRS complex. This method requires knowledge of the underlying paper or sweep speed. The R‐R interval can be counted in terms of small boxes (mm); the number of boxes counted can then be multiplied by s/mm (0.02 for 50 mm/s paper speed and 0.04 for 25 mm/s paper speed) to obtain the amount of time (seconds) per beat. To convert this into beats per minute, simply divide 60 (s/min) by the number of seconds per beat. For example, an R‐R interval that was counted to be 18 small boxes at a paper speed of 25 mm/s would be:
11
Electrocardiography
Introduction
Electrical potentials and the lead system
Einthoven’s triangle
Bipolar, unipolar, and precordial leads
The ECG complex
Evaluating an ECG
Heart rate calculation
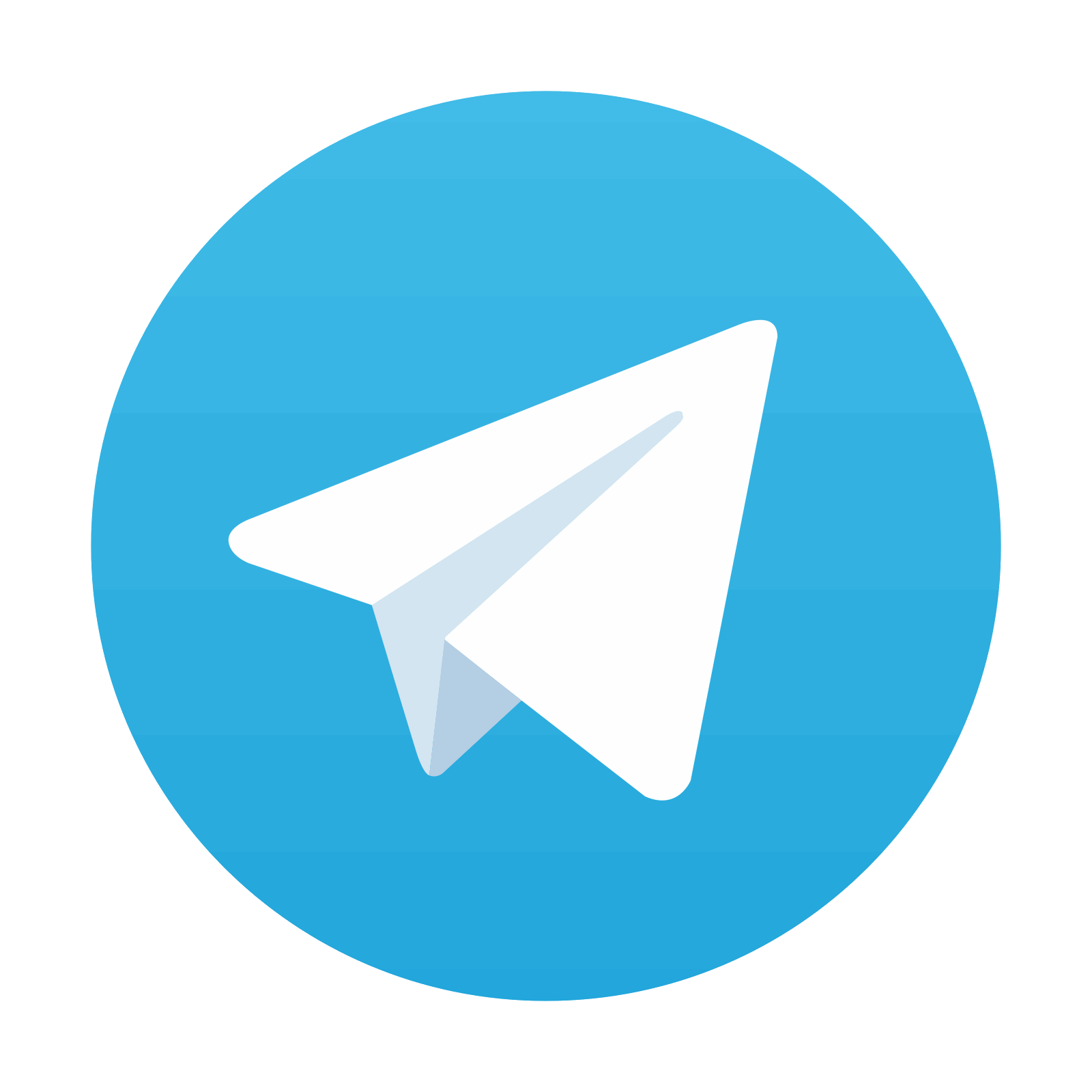
Stay updated, free articles. Join our Telegram channel

Full access? Get Clinical Tree
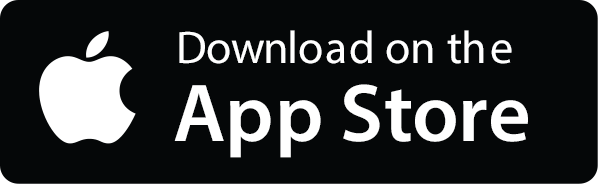
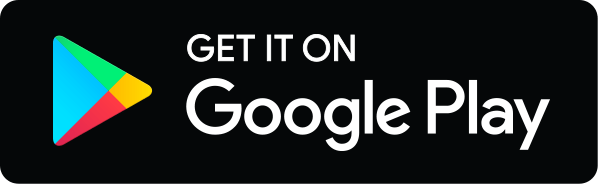