CHAPTER 56 Effects of Environment on Bovine Reproduction
EFFECTS OF HEAT STRESS ON REPRODUCTIVE FUNCTION
Among the highest physiologic priorities of all homeotherms is maintenance of body temperature. The cow maintains a constant core body temperature of approximately 38.5° C by matching internal heat production with net loss of heat to the environment. In situations leading to hyperthermia (internal heat production greater than net loss of heat), thermoregulatory regions of the central nervous system engage physiologic systems to reduce internal heat production and increase net heat flow from the body. Several physiologic changes that stabilize body temperature are deleterious to reproduction. In addition, the hyperthermia experienced by heat-stressed cows can itself compromise reproductive function. Taken together, these effects lead to a severe reduction in reproductive performance of cattle.
Detection of Estrus
As shown in Figure 56-1, ability to detect estrus in Bos taurus declines during periods of heat stress. This decline occurs in large part because of a reduction in duration of estrous behavior. In contrast with B. taurus, evidence for decreased duration of estrus in Bos indicus by heat stress is scarce. Under Florida conditions, estrus in Brahman heifers averaged 8.6 ± 2.3 hours in spring, 5.5 ± 1.2 hours in summer and 5.4 ± 0.5 hours in fall and 6.8 ± 1.6 hours in winter.1 In this same study, under conditions in which variation in feed availability was minimal, the proportion of ovulations without detected estrus was greater in winter (59.7%) than in summer (40.3%).
Effects of Heat Stress on Establishment of Pregnancy
Data in Figure 56-2 illustrate the marked seasonal depression in pregnancy rates per insemination of artificially inseminated dairy cattle in warm months of the year. This depression is most apparent in regions of the world with hot climates but can occur in more temperate areas also. Heat stress is the major environmental factor responsible for lowered pregnancy rates in the summer. Experimental exposure of cattle to heat stress reduced pregnancy rate and embryonic survival, whereas provision of cooling during the summer improved the frequency of cows’ becoming pregnant after insemination (Table 56-1). Moreover, the magnitude of the depression in pregnancy rate is proportional to the degree of hyperthermia; this has been demonstrated for both dairy and beef cows.
It is important to define the phases of the reproductive process leading to fertilization and embryonic development that are sensitive to maternal hyperthermia, because together these phases dictate the critical windows in which cows must be kept homeothermic to maintain high pregnancy rates. Disruption of the reproductive process can occur quite early—during oocyte growth or maturation. Although little seasonal effect on fertilization rate for oocytes subjected to in vitro fertilization (IVF) has been observed, fertilized oocytes collected from cows during the summer are less likely to give rise to an embryo capable of development to the blastocyst stage than are embryos collected during the winter. Seasonal variation in performance of embryo production systems based on IVF has been observed even in a relatively cool region like Wisconsin. Additional evidence from Israel suggests that oocyte quality gradually improves as season progresses from early to late autumn, as supported by data from studies in which cows were subjected to multiple oocyte recoveries.
In another study, it was demonstrated that heat stress of superovulated cows reduced development and viability of embryos if cows were exposed to heat stress at day 1 after estrus but not if heat stress was given on day 3, 5, or 7 after estrus (Fig. 56-3). Moreover, the reduction in development to the blastocyst stage caused by exposure of cultured embryos to elevated temperature declined as embryos proceeded through development (see Fig. 56-3). Thus, embryos become more resistant to effects of heat stress as pregnancy proceeds. Nonetheless, the embryo can still be damaged by severe heat stress even late in development. For example, heat stress of beef cows from days 8 to 16 of pregnancy reduced conceptus size at day 17.
Causes of Heat Stress–Associated Pregnancy Failure
Heat stress could compromise oocyte competence by at least three possible mechanisms: (1) Disruption in patterns of folliculogenesis could lead to ovulation of an aged oocyte with lowered potential for fertilization. Consistent with this idea is the finding that heat stress beginning at day 1 of the estrous cycle caused earlier emergence of the dominant follicle of the second follicular wave. Such an effect could conceivably alter the quality of the oocyte ovulated at the subsequent estrus, because oocytes from persistent dominant follicles have reduced competence. This mechanism must not be the only one operational, however, because it does not explain why season (and presumably heat stress) adversely affects multiple oocytes in the cohort of follicles ovulated after superovulation, collected by transvaginal ultrasound–guided recovery, or aspirated from excised ovaries. (2) Heat stress also has been reported to affect follicular steroidogenesis. Perhaps alterations in the steroidal environment disrupt oocyte development. (3) Finally, oocytes may be similar to male germ cells in that they have heightened sensitivity to elevated temperature. Unlike most cells, the oocyte in developing follicles is transcriptionally inactive after it reaches a diameter of approximately 110 μm (i.e., at about the 3-mm follicle stage). This means that the range of cellular adjustments to elevated temperature that are possible in the oocyte are limited to those not involving transcription. For example, bovine oocytes cannot undergo increased synthesis of heat shock protein 70 (HSP70) in response to elevated temperature.
Sensitivity to elevated temperature persists for several cleavage divisions after fertilization, with the embryo acquiring increased resistance to heat shock as it proceeds through development. Thus, the increased resistance of cows to heat stress as pregnancy proceeds may reflect developmental changes in the embryo that lead to greater cellular resistance to elevated temperatures (see Fig. 56-3). The molecular basis for increased embryonic thermotolerance is not known. Heat shock protein synthesis in embryos exposed to elevated temperature occurs as early as the four-cell stage, and other biochemical changes may therefore also be important.
Effects of Heat Stress in Late Pregnancy and the Postpartum Period
Abortion caused by heat stress is rare, but placental function is compromised by heat stress in the last third of pregnancy. Thus, in one study, secretion of the placental hormone estrone sulfate, placental size, and calf birth weight were reduced in the summer or by experimental heat stress (Table 56-2). These effects of heat stress probably are caused primarily by the reduction in blood flow to the placenta that occurs with heat stress. In one study in California, calf mortality in the summer increased as environmental temperature increased. This relationship could reflect the lower calf birth weights caused by heat stress, as well as reduced immunoglobulin transfer to the neonate.
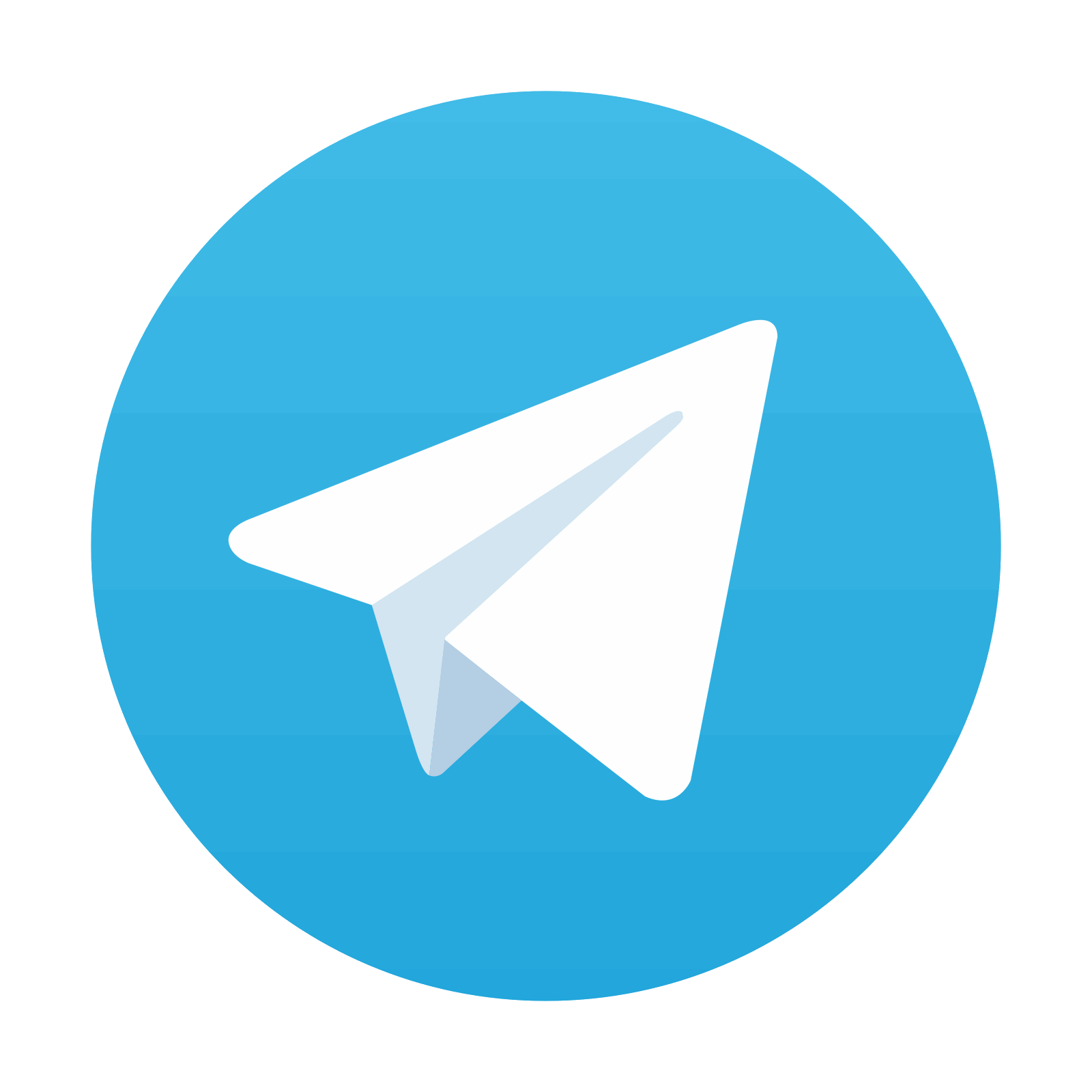
Stay updated, free articles. Join our Telegram channel

Full access? Get Clinical Tree
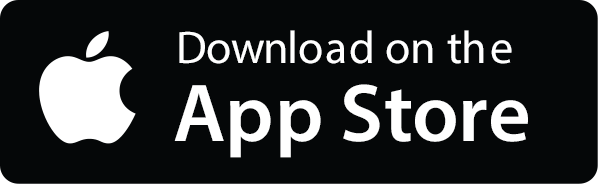
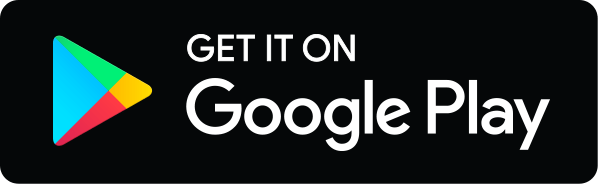