Chapter 64 Peter J. Hansen Department of Animal Sciences, University of Florida, Gainesville Florida, USA Adverse changes in the maternal environment can perturb embryonic development and lead to embryonic loss. Among the most consequential of these changes is hyperthermia, which occurs most typically because of heat stress but also via pyrogens generated during inflammation. Even a small increase in body temperature can compromise embryonic survival. In lactating dairy cows,1 conception rate declined 6.9–12.8% for each 0.5 °C increase in uterine temperature above the mean temperature of 38.3–38.6 °C. In this study, rectal temperature was about 0.2 °C lower than uterine temperature1 and therefore it can be inferred that fertility declines when rectal temperature reaches about 39 °C. In an earlier study, fertility of lactating dairy cows also declined at rectal temperatures of 39 °C and higher.2 Embryonic loss caused by maternal hyperthermia is largely caused by direct and indirect actions of elevated body temperature on oocyte function and embryonic development. The window in the reproductive process when hyperthermia can cause embryonic loss is a wide one. It extends from as early as 15–19 weeks before ovulation, because exposure to a 28-day heat stress reduces the competence of the oocyte to become a blastocyst 15 weeks after the termination of heat stress,3 and continues until 2–3 days after ovulation when the preimplantation embryo becomes resistant to disruption by elevated temperature.4–8 This chapter will focus on the mechanisms by which heat stress compromises the oocyte and embryo and the implications for management strategies to improve fertility during heat stress. Throughout the chapter, the term “heat stress” will refer to the combination of environmental characteristics that reduce the capacity of an animal to regulate its body temperature while “heat shock” will refer to the effect of elevated temperature on cellular function. Heat stress can affect oocyte function during two distinct periods of oocyte development. The first is the period of follicular growth, when the oocyte experiences activation and then silencing of the genome, establishes communication with cumulus cells, and synthesizes cortical granules.9 The second period is following ovulation when the oocyte undergoes nuclear and cytoplasmic maturation in preparation for fertilization and embryonic development. Damage to the oocyte during follicular growth has been demonstrated in experiments to evaluate seasonal variation in the competence of oocytes (harvested at slaughter or via ultrasound-guided aspiration) to be fertilized in vitro and develop into blastocysts. In these experiments, which used lactating Holstein cows, the proportion of oocytes that became blastocysts after in vitro fertilization10–12 or chemical activation13 was lower in summer than winter (see Figure 64.1 for an example). Figure 64.1 Seasonal variation in Brazil for oocyte competence for development in Holstein heifers (H), lactating cows at the peak of lactation (PL), and lactating cows classified as repeat breeders (RB). Oocytes were harvested by transvaginal aspiration and subjected to in vitro maturation and fertilization. Resultant embryos were cultured to the blastocyst stage. Asterisks represent differences within animal type between seasons. Letters above each bar represent differences between animal types within an individual season. Data are reproduced from Ferreira R, Ayres H, Chiaratti M et al. The low fertility of repeat-breeder cows during summer heat stress is related to a low oocyte competence to develop into blastocysts. J Dairy Sci 2011;94:2383–2392 with permission. The seasonal effects on blastocyst formation largely represented decreased competence of cleaved embryos to develop rather than competence of the oocyte to cleave. One reason is that heat stress reduces accumulation of molecules in the oocyte important for early embryonic development. Actually, there are differences in transcript abundance between embryos produced by in vitro fertilization of oocytes collected in summer versus winter.14 Also, perhaps, oocytes in the summer are more likely to undergo abnormal chromosomal segregation so that subsequent embryos are chromosomally abnormal. Occurrence of chromosomal abnormalities can affect the rate of embryonic development.15 Appropriate follicular development is critical to the ovulation of an oocyte competent to be fertilized and able to transform into an embryo capable of successful development.16,17 Heat stress can alter follicular dynamics by reducing growth of the dominant follicle and increasing the number of smaller-sized follicles.3,18–20 Furthermore, heat stress can reduce follicular concentrations of estradiol-17β21 and insulin-like growth factor (IGF)-1,20 while increasing follicular concentrations of androstenedione.21 The mechanism by which heat stress alters follicular development is not well understood. Experimentally, heat stress can reduce luteinizing hormone (LH) secretion.22,23 There may also be direct effects of elevated body temperature on steroid synthesis. Culture of cumulus cells at 40.5 °C reduced estradiol-17β production in the fall but not in the summer or winter.21 In the same study, incubation at 40.5 °C reduced forskolin-stimulated androstenedione production by thecal cells when cells were collected in winter but not when cells were collected in summer or fall. Heat shock of 41 °C reduced basal and gonadotropin-stimulated synthesis of androstenedione and estradiol-17β by cultured follicular walls from the dominant follicle.24 In some cases, heat stress can cause a reduction in circulating progesterone concentrations25 and such a decline could conceivably contribute to a reduction in oocyte competence. This idea is based on the observation that fertility following ovulation of a first-wave dominant follicle, when circulating progesterone concentrations are low, is less than fertility following ovulation of a second-wave dominant follicle, when progesterone concentrations are high.26,27 Moreover, supplementation with progesterone during the first follicular wave improved fertility.27 Summer heat stress is followed by a gradual restoration of oocyte competence for fertility28,29 and oocyte competence for chemically activated development.30 These observations are symptomatic that heat stress can affect follicular development early in the 16-week period that is required for a primordial follicle to reach dominance.31 Indeed, there is experimental evidence for delayed effects of heat stress on function of the follicle and oocyte. In one study,32 exposure of lactating Holsteins to heat stress affected follicular steroid production 20–26 days later. An experiment in Gir cattle (Bos indicus) indicated that carry-over effects of heat stress can persist for up to 133 days after initiation of heat stress (i.e., 105 days after the end of heat stress).3 Cows were exposed to heat stress for 28 days and oocytes aspirated and subjected to in vitro maturation and fertilization at weekly intervals for up to 133 days after the beginning of heat stress. Heat stress did not affect follicular dynamics during the 28-day heat stress period but increased the diameter of the first and second largest follicles, as well as the number of follicles over 9 mm, from days 28 to 49 after initiation of heat stress. Moreover, the percent of oocytes that became a blastocyst was reduced by heat stress even at the end of the experiment at 133 days after initiation of heat stress (Figure 64.2). Figure 64.2 Changes in developmental competence of oocytes harvested from Gir cows that were either experimentally heat-stressed for 28 days (HS) or were maintained in a control environment (control group, CG). Oocytes were subjected to in vitro maturation and fertilization. Resultant embryos were cultured to the blastocyst stage. Note that the competence of oocytes to become blastocysts remained lower for the HS group for up to 133 days after the initiation of heat stress (i.e., 105 days after the end of heat stress). Data are reproduced from Torres-Júnior JR de S, de FA Pires M, de Sá WF et al. Effect of maternal heat-stress on follicular growth and oocyte competence in Bos indicus cattle. Theriogenology 2008;69:155–166 with permission. The oocyte remains susceptible to disruption by heat stress during the process of nuclear and cytoplasmic maturation coincident with ovulation. Heat stress of superovulated heifers for a 10-hour period beginning at the onset of estrus and before insemination at 15–20 hours after the beginning of estrus decreased the proportion of embryos recovered at day 7 after estrus that were classified as normal.33 There was no effect of heat stress on fertilization rate so damage to the oocyte gave rise to an embryo with reduced competence for development. Some of the effects of heat stress on oocyte function during maturation could be due to alterations in hormone secretion because heat stress lowered the magnitude of the preovulatory surge in LH and estradiol-17β.23,34 In addition, exposure to elevated temperature can induce errors in oocyte maturation. Culture at elevated temperatures during maturation reduced the proportion of oocytes that completed nuclear maturation, increased the proportion with abnormal spindle formation, cortical granule distribution, depolarized mitochondria and apoptotic pronuclei, and decreased the proportion that cleaved and became blastocysts after insemination.35–40 Apoptosis has been implicated in disruption of oocyte function during maturation. Inhibition of apoptosis with a caspase inhibitor,36 sphingosine 1-phosphate,41 or inhibitor of ceramide synthesis42 reduced the effects of heat shock on developmental competence of the oocyte. There are also indications that the effects of heat shock involve acceleration of maturation and oocyte aging. Exposure of oocytes to 41 °C for the first 12 hours of maturation hastened both nuclear and cytoplasmic maturation.43 Moreover, the deleterious effects of heat shock on development could be avoided if oocytes were fertilized at 19 hours of maturation instead of 24 hours43 or if chemical activation was used to trigger development.44 As shown in Figure 64.3, there are differences in fertilization rate between lactating cows in summer and winter.45 Reduced fertilization rate is probably largely caused by damage to the oocyte. The other cell important for fertilization, the spermatozoon, is much more resistant to elevated temperature. Formation of spermatozoa in the testis can be compromised by heat stress,46 but function of the spermatozoa deposited in the female reproductive tract is probably not disrupted by maternal hyperthermia. Heat shock did not reduce fertilizing capacity of sperm in vitro or subsequent development of embryos to the blastocyst stage.47,48 There are reports in the rabbit that exposure of sperm to elevated temperature in vitro49 or in vivo50 can result in abnormalities in development, leading to a reduction in the number of implantation sites despite a lack of reduction in fertilization rate. More work is necessary to determine if damage to the spermatozoa by heat shock can lead to developmental abnormalities later in development in cattle. Figure 64.3 Seasonal changes in fertilization rate in Holsteins in Wisconsin. The reproductive tract of inseminated females was flushed 5 days after ovulation to recover oocytes and embryos. Fertilization rate was based on the number of recovered embryos (two-cell or greater) divided by the number of recovered oocytes and embryos (see numbers above each bar). For lactating cows, there was a decrease in fertilization rate in the summer compared with winter. For nonlactating animals, however, there was no difference in fertilization rate between seasons (compare dry cows in winter with heifers in summer). Data are taken from Sartori R, Sartor-Bergfelt R, Mertens S, Guenther J, Parrish J, Wiltbank M. Fertilization and early embryonic development in heifers and lactating cows in summer and lactating and dry cows in winter. J Dairy Sci 2002;85:2803–2812. When first formed, the preimplantation embryo is very susceptible to elevated temperature. A heat shock as low as 40 °C for 12 hours can reduce development of zygote-stage embryos to the blastocyst stage.51 Heat shock can also reduce developmental potential of embryos at the 2-cell stage of development,7,52 but thereafter the embryo becomes more resistant to heat shock. Development of 4- to 8-cell embryos can be compromised by heat shock but to a lesser extent than for 2-cell embryos.52 By the morula stage of development, the embryo is substantially resistant to deleterious effects of heat shock.7,8,51,52 The same phenomenon is observed in vivo. Exposure of superovulated cows to heat stress reduced blastocyst yield at day 8 after estrus when occurring at day 1 after estrus but not when occurring at day 3, 5, or 7 after estrus.4 The 2-cell embryo exposed to 41 °C experiences alterations in ultrastructure characterized by swelling of mitochondria and movement of organelles toward the center of the blastomere53 (Figure 64.4). Organellar movement is caused by disruption of the cytoskeleton.54 The early embryo exposed to heat shock also experiences a decrease in protein synthesis.5 Figure 64.4 Effects of heat shock (41°C for 6 hours) on the ultrastructure of the two-cell bovine embryo produced in vivo. (a, c) Images taken using light microscopy after staining with toluidine blue O; (b, d) images from transmission electron microscopy. (a, b) Embryos cultured at 38.5°C; (c, d) heat-shocked embryos. Arrows show an area of the cytoplasm devoid of organelles. ZP, zona pellucida; PVS, perivitelline space; N, nucleus; MT, mitochondrion; Ly, lysosome. Images are reproduced from Rivera R, Kelley K, Erdos G, Hansen P. Alterations in ultrastructural morphology of two-cell bovine embryos produced in vitro and in vivo following a physiologically-relevant heat shock. Biol Reprod 2003;69:2068–2077 with permission. Some of the damage caused by heat shock could be the result of increased production of free radicals as has been reported for embryos at days 0 and 2 after insemination.55 Also, effects of heat shock were greater in 2-cell embryos cultured in a high oxygen atmosphere that would promote oxidative stress.56 In contrast, the effects of heat shock were independent of oxygen tension in other studies.51,57 Similarly, the antioxidant anthocyanin58 and dithiothreitol56 have been reported to mitigate the actions of heat shock whereas other antioxidants including vitamin E,59 glutathione,60 and glutathione ester60 were reported to be without embryoprotective properties. Effects of heat stress on embryonic survival in vivo could include actions on the reproductive tract as well as on the embryo directly. Heat stress can reduce the magnitude of the preovulatory surge in estradiol-17β23 and this could conceivably change function of the oviduct or endometrium. The direct effects of heat shock on oviductal or endometrial function have not been examined at temperatures characteristic of those experienced by heat-stressed cows. However, it is likely that these tissues are resistant to heat shock because even a temperature as high as 43 °C did not have major effects on protein synthesis by endometrium or oviduct.61 Several hypotheses have arisen to explain the process by which the embryo transitions from a state in which it is very susceptible to heat shock, at the 1-cell to 2-cell stage, to one where it has acquired resistance to heat shock, by the 16-cell stage. One possibility is that the early embryo is very susceptible to heat shock because processes required for embryonic genome activation are easily disrupted by heat shock. If this was the case, embryos exposed to heat shock at the 2- or 4-cell stage should block in development between the 8-cell and 16-cell stages; embryonic genome activation is required for development past this stage.62 Such a preferential block at the 8-cell to 16-cell transition was seen in one experiment with 2-cell embryos57 but not in another where embryos were heat-shocked at the 1-cell stage.51 Another possibility is that the acquisition of capacity for transcription after the 8-cell to 16-cell stage allows the embryo to engage a wider range of stress responses to stabilize cellular function during heat shock. However, steady-stage amounts of mRNA for some important stress proteins are higher in the 1-cell or 2-cell embryo than the morula51,62 (Figure 64.5), probably because transcripts are inherited from the oocyte. Heat shock can induce synthesis of heat-shock protein 70 as early as the 2-cell stage.5,63 Moreover, the morula-stage embryo undergoes a muted transcriptional response to heat shock, with only 4 of 68 genes associated with the heat-shock response showing an increase in response to elevated temperature.64 Thus it might be that the damage to cellular macromolecules caused by heat shock that trigger stress protein responses is reduced in embryos at resistant stages of development. One reason might be a change in the balance between free radical generation and antioxidant protection. Heat shock caused increased free radical production at days 0 and 2 but not at days 4 and 6.55 In addition, the cytoplasmic antioxidant glutathione is at its lowest during the 2- to 8-cell stages.65 Figure 64.5 Differences in steady-stage amounts of mRNA for HSPA1A (heat-shock protein 70), HSP90AA1 (heat-shock protein 90), SOD1 (superoxide dismutase), and CAT (catalase) between embryos harvested at the zygote (day 0) or morula stage (day 5) and cultured for 24 hours at 38.5°C (open bar) or 40°C (black bar). . Data are reproduced from Sakatani M, Alvarez N, Takahashi M, Hansen P. Consequences of physiological heat shock beginning at the zygote stage on embryonic development and expression of stress response genes in cattle. J Dairy Sci 2012;95:3080–3091 with permission
Early Embryonic Loss Due to Heat Stress
Nature of the problem
Contribution of the oocyte to embryonic loss
Oocyte damage during follicular growth
Oocyte maturation
Fertilization failure: oocyte versus sperm
Damage to the preimplantation embryo
Mechanism for embryonic mortality caused by heat stress
Developmental acquisition of thermotolerance
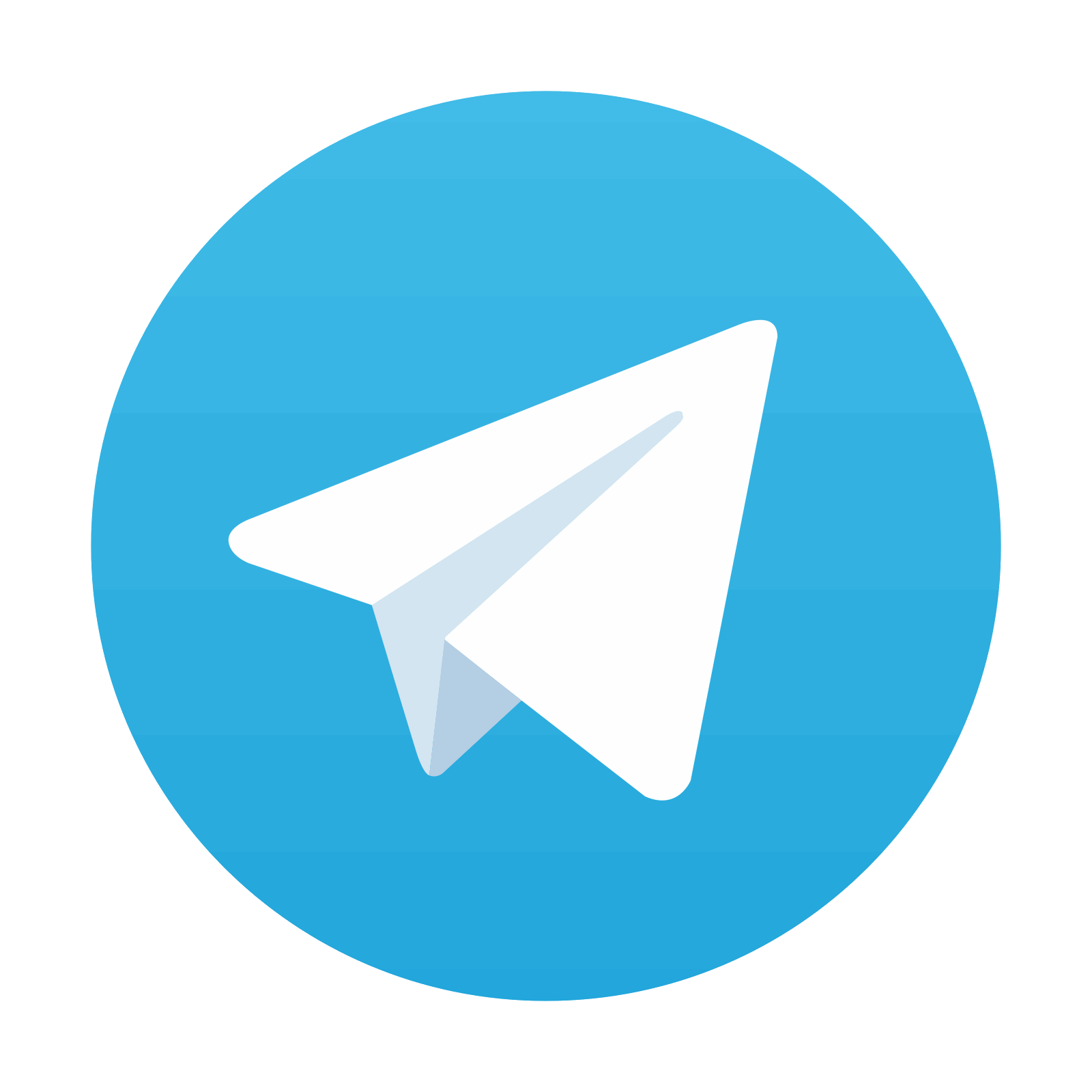
Stay updated, free articles. Join our Telegram channel

Full access? Get Clinical Tree
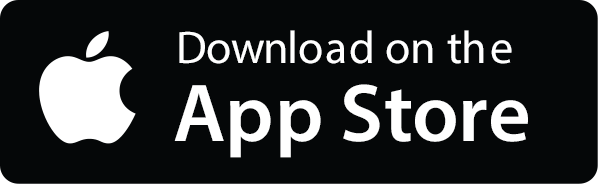
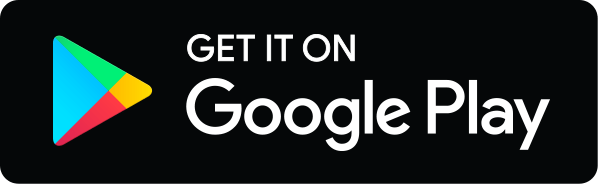