Fig. 1.
Intact, lumbar dorsal root of adult rat. Horizontal section through several dorsal rootlets, immunostained with antibodies to laminin (green) and glial fibrillary acidic protein (GFAP; red ). The dorsal root transitional zone is formed by cone-shaped peripheral projections of central nervous tissue (red), which interdigitate with the peripheral nervous compartment of the root (green) (×200).
After maturation, the prototypic dorsal root ganglion neuron emits one single axon which branches into one process projecting peripherally and one along the dorsal root. At their exit from the dorsal root ganglion, dorsal root axons are collected in a single well circumscribed bundle, surrounded by Schwann cells, basement membrane and a thin connective tissue sheath. Between the dorsal root ganglion and spinal cord, thin subcomponents, rootlets, emerge and display a divergent course toward the spinal cord, giving the roots a fan-like appearance. The fan-like appearance is most striking at the cervical and upper thoracic levels where distances between dorsal root ganglia and dorsal root entry sites are short and the filaments rapidly separate from each other. Some distance outside the spinal cord surface dorsal root axons encounter a loose network mostly composed of astroglial processes, which project peripherally, intermingle with Schwann cell processes and together with oligodendroglial processes form the central tissue projections or glial dome (9, 10, 16). At the transition from PNS to CNS myelinated axons typically have a node of Ranvier with Schwann cell processes surrounding its peripheral and astroglial processes its central part (9, 10, 17). Since myelination in the CNS is triggered at axonal diameters smaller than in the PNS (18) the larger non-myelinated axons in the PNS become myelinated after their entry in the spinal cord (19).
1.3 Advantages of Dorsal Root Injury for Spinal Cord Repair
The sensory neurons in dorsal root ganglia have been and remain attractive for studies on nerve regeneration from the perspective of the PNS as well as the CNS (20). The location of these neurons outside the spinal cord and the complex CNS neural circuitry has practical advantages, and their peripheral or central axons are easily axotomized. Dorsal root ganglion neurons are also accessible for selective experimental manipulation in vivo, e.g., for electroporation of genetic constructs (21). The physiological properties and functional role of the subset of dorsal root ganglion neurons are known in great detail. Nerve cell body and associated non-neuronal responses can therefore be examined in a relatively simple anatomical and physiological context. The dorsal root injury model can also serve as a useful model in cell replacement therapy, as has been shown with implantation into the dorsal root ganglion cavity of embryonic sensory neurons (22, 23), or stem/progenitor cells (24–26). The popularity of dorsal root injury as a model for the study of spinal cord regeneration aspects has perhaps also a historical explanation, since it was a prime example used by Cajal to demonstrate the failure of injured axons to regenerate in the central CNS in contrast to their unimpeded growth in the PNS (27).
2 Dorsal Root Injury Models: Defining Their Objectives
Three principally different types of dorsal root injury should be distinguished on the basis of the site of injury and their pathophysiological consequences for the spinal cord.
Injuries that interrupt dorsal root axons at a restricted site along their course in the PNS compartment of the dorsal root.
Injuries where the dorsal roots are interrupted at their entry into the spinal cord.
Injuries that pull the dorsal root away from its attachment to the spinal cord.
2.1 Injuries that Interrupt Dorsal Root Axons at a Restricted Site Along Their Course in the PNS Compartment of the Dorsal Root
In terms of models for spinal cord repair a localized dorsal root injury in the PNS compartment of the dorsal root primarily addresses the issue of axon regeneration from a permissive (PNS) into a non-permissive (CNS) environment. In this case, injury-induced axon elongation is initiated and the behavior of axons is studied when they reach the interface between the PNS and CNS and attempt to enter the spinal cord. Mechanisms underlying failure of dorsal root axons to re-enter the spinal cord can be explored and approaches designed to attempt to overcome this regeneration failure. For injury reproducibility, sectioning the dorsal root is the best. However, this approach has shortages for studying regeneration of dorsal root axons—proximal and distal stumps of the dorsal root have to be re-apposed, and fixed to each other, usually by biological material such as fibrin glue. If the repair site is not prepared in an optimal way it may present an obstacle for axons to cross the injury site. Crushing the dorsal root eliminates this constraint, but is more prone to injury variability and raises the issue of how to ensure completeness, in particular as it comes to the non-myelinated axons. Since the connective tissues of dorsal root are more delicate than those of peripheral nerves forceful or repeated crushes may result in a complete separation of proximal and distal root stumps. To establish crush injury of the dorsal root as the model, requires control experiments with careful anatomical examination of the injury site and the portion of the nerve isolated from the nerve cell body. Physiological verification that impulse propagation completely fails across the injury site is an additional control, although a transient conduction block without interruption of axonal continuity may be a potential pitfall (cf. (28)).
2.2 Injuries Where the Dorsal Roots Are Interrupted at Their Entry into the Spinal Cord
Interrupting dorsal root axons at the site of their entry into the spinal cord is a useful injury model for probing interventional strategies which may promote functionally useful growth of dorsal root axons into the spinal cord. The anatomy at the junction between dorsal root and spinal cord limits the type of injury to sectioning the individual rootlets, taking care to minimize damage to the spinal cord itself. The procedure involves exposing target dorsal root(s) via a partial laminectomy, carefully lifting the roots close to their entry site, and thereafter cutting the root filaments. Recent examples using this model are studies in which multiple dorsal roots were sectioned, re-attached, and olfactory ensheathing cells implanted at the injury site to bridge growing dorsal root axons (29, 30). This injury model is in principle a CNS type injury, and more closely related to clinical root avulsion injury than the injury models described above. Still, this injury model does not mimic the abrupt and powerful longitudinal forces subjected to the dorsal roots and their entry sites in clinical root avulsion.
2.3 Injuries that Pulls the Dorsal Root(s) Away from Its Attachment to the Spinal Cord
The models that most closely reflect clinical dorsal root avulsion injury involve pulling dorsal roots away from their attachment with the spinal cord. This type of injury results in damage to the DRTZ and spinal cord which is significantly more extensive than after just cutting or crushing the dorsal roots. This injury can be achieved either by forceful pulling of one or more spinal nerves, e.g., the brachial plexus, outside the vertebral column (31–35), or by exposing dorsal root(s) at their entry into the spinal cord and thereafter pulling them away from the spinal cord (36–38). Pulling spinal nerve(s) breaks both ventral and dorsal roots without visual observation of the actual injury, which may increase its variability. Avulsing only dorsal roots will obviously not reflect the entire pattern of pathophysiological and functional effects of clinical plexus avulsion injury, but allows better reproducibility. Both models have so far been explored mainly for spinal cord pathophysiology and neuropathic pain induced by deafferentation, rather than for the purpose of studying regeneration of injured dorsal root axons.
3 Diversity of Dorsal Root Ganglion Neurons and Their Spinal Cord Projections
The compositions of dorsal root ganglia are quite complex, reflecting the broad range of sensory information conveyed by dorsal root ganglion neurons from the periphery to the spinal cord. These features should be kept in mind in outcome assessments after dorsal root injury. Dorsal root ganglion neurons display distinct differences in structure, content of neurosignaling molecules, receptor expression, functional properties (39–42), axonal course into the spinal cord (43), and central projections of their axon terminals (44–46). A majority of mammalian dorsal root axons are non-myelinated, enter the spinal cord mainly in the lateral compartment of the dorsal root and terminate on neurons in the dorsal horn within the entry segment as well as in several segments rostral and caudal to the level of entry. Myelinated dorsal root axons enter the spinal cord within the medial compartment of the dorsal root and reach the dorsal horn largely by turning laterally from the dorsal funiculus. In general axons of myelinated dorsal root axons terminate more ventrally in the dorsal horn than non-myelinated ones. Importantly, collaterals of sensory axons that mediate information from low-threshold mechanoreceptors in skin, muscle and joints, ascend in the dorsal funiculus to terminate in, e.g., the column of Clarke (proprioceptive information), or the dorsal column nuclei (cutaneous mechanical information and positional information). A smaller fraction of myelinated axons that convey sensory information from muscles, including muscle spindle afferents, reach the ventral horn where they terminate on motor neurons or interneurons. The further routes through the spinal cord of the sensory information from the periphery are described in Chap. 2.
4 The Dorsal Root Injury Model for Exploring Basic Aspects of Spinal Cord Repair
This section will briefly highlight some of the most relevant previous contributions from studies using the dorsal root injury model as well as some future challenges for which this model offers an attractive experimental potential. The failure of injured dorsal root axons to enter the mammalian spinal cord is most likely a consequence of the combination of insufficient regenerative response by axotomized dorsal root ganglion neurons and the emergence of growth inhibitory properties of the DRTZ. Thus, the dorsal root ganglion model is useful for exploring neuronal as well as environmental components underlying mammalian CNS regeneration failure, and how these two processes interrelate. Studies in amphibian show that injured dorsal root axons are able to regenerate into the spinal cord and restore sensorimotor reflex activities (47–51). The mechanisms underlying this regenerative capacity are largely unexplored and deserve more attention.
The preferred model for exploring insufficient neuronal growth responses is to cut or crush dorsal root(s) at a distance from the DRTZ. Lesions at the lumbosacral level offer the best possibility to perform this with minimal direct disturbance of the spinal cord. Typically, one or more of lumbar dorsal roots L4–6 are targeted, since they carry all or almost all of the sensory afferents from the sciatic nerve.
4.1 Exploring Properties of Dorsal Root Ganglion Neurons that Promote Regenerative Growth of Dorsal Root Axons
Axotomized CNS neurons appear to be intrinsically deficient in their capacity to mount and sustain an efficient program for long distance axonal growth. Furthermore, this capacity shows a significant decline with age. Dorsal root ganglion neurons respond in a similar way after dorsal root injury. Axotomy of the central processes of dorsal root ganglion neurons elicits a much weaker nerve cell body growth response than axotomy of their peripheral processes. As a consequence, the rate of regeneration of dorsal root axons is significantly less than that of regenerating peripheral sensory axons, ca. 1 and 3 mm, respectively in the rat (2), regenerating dorsal root axons have limited ability to grow for long distances in a peripheral nerve environment (52, 53), and are practically unable to enter the adult spinal cord. The dorsal root injury model has provided important information on interventions that enhance long distance axonal outgrowth, either by directly reactivating the intrinsic growth potential or by extracellular stimuli. The conditioning lesion paradigm has been paramount in this respect, demonstrating that injury of the peripheral processes prior to an injury of the central processes of the same sensory neurons mobilizes growth-associated molecules in dorsal root ganglion cells and enhances their capacity for elongation of injured dorsal root axons (54–59). This action allows limited number of regenerating axons to enter the spinal cord (60), but these presumably belong to a subpopulation of dorsal root axons, since a conditioning lesion appears to selectively stimulate the growth potential of dorsal root ganglion neurons not expressing calcitonin gene-related peptide or binding isolectin B4 (61). The conditioning lesion paradigm has also provided information on conditions that allow elongation of injured axons in the CNS. Thus, injury to peripheral sensory axons results in significant growth of injured primary sensory axons in the dorsal column (62), an effect that can be induced even a considerable time after the central lesion has occurred (63).
Extracellular delivery of growth factors enhances the potential of dorsal root regeneration into the spinal cord. The most striking effects have been shown with intrathecal delivery of neurotrophin (NT)3, or glial cell line-derived neurotrophic factor (GDNF) (64, 65), or systemic administration of Artemin (66, 67), a member of the GDNF family. These effects may be mediated by intracellular pathways which are at least in part similar as in the conditioning lesion situation (57), but concomitant effects on the properties of non-neuronal cells at the DRTZ may also occur (68). Notably, the time window for neurotrophin-mediated entry of regenerating dorsal root axons is restricted, since delayed treatment failed to induce spinal cord ingrowth (69).
Recently new mechanisms regulating the intrinsic growth state of neurons during postnatal development and after axon injury have been explored. Members of the Krüppel-like family of transcription factors regulate axonal growth development and their re-activation postnatally promotes axon regeneration in vivo (70, 71). PTEN (phosphatase and tensin homolog deleted on chromosome 10), inhibits phosphoinositide 3-kinase (PI3-K)/Akt signaling, a pathway critical for sustained neurite outgrowth. PTEN activation also appears to participate in mediating the growth inhibitory effect of myelin-associated glycoprotein (MAG) (72). Blocking the action of PTEN significantly promotes regenerative outgrowth in vivo from retinal ganglion cells (73, 74), corticospinal neurons (75), and even from peripheral nerve axons (76). Another intracellular signaling system which regulates the capacity for neural regeneration is the Jak-STAT (signal transducer and activator of transcription) pathway. SOCS3 (suppressor of cytokine signaling 3) inhibits this pathway, and relieving this inhibition markedly promotes regeneration of optic nerve axons (77), which is further enhanced by co-inactivation of PTEN (78). Combining PTEN inactivation with cAMP and the macrophage derived factor oncomodulin also enhanced growth of injured optic nerve axons (79), although oncomodulin by itself appears to have little effect on the regenerative capacity of injured dorsal root axons (80).
Another line of exploration has highlighted the fundamental role of microtubule stability for axonal regeneration in the CNS (81). Studies in non-mammalian species demonstrated efficient microtubule-based axon regeneration without the formation of growth cones (82). Treatment with the microtubule stabilizing agent taxol promoted axon regeneration in the mammalian spinal cord (83) and optic nerve (84). Furthermore, inhibition of kinesin-5, a motor protein that counteracts microtubule advancement, promotes sensory neurite extension on a growth inhibitory substrate in vitro (85). The dorsal root injury model is undoubtedly a useful model for exploring the intracellular pathways that are implicated in these effects, how these pathways interact (86) and in the identification of additional, hitherto unknown molecular mechanisms that could be targeted to optimize conditions for regeneration in the spinal cord.
4.2 Exploring the Growth Inhibitory Properties of the DRTZ
Within days after dorsal root injury the DRTZ rapidly presents a virtually full-proof barrier for sensory axons to re-enter the spinal cord. A reasonable explanation for this situation would be the presence of a non-permissive environment for axon growth at the DRTZ, more or less analogous with the inability of adult degenerating CNS tissue in general to support axon growth. After dorsal root injury, DRTZ astrocytes proliferate (87), undergo a marked hypertrophy (88, 89) (Fig. 2), and growth inhibitory proteoglycans as well as myelin-associated growth inhibitors, including Nogo, myelin-associated glycoprotein (MAG) and oligodendrocytes myelin glycoprotein (OMgp) accumulate at this site (90, 91). Blockade of the Nogo-receptor (NgR) using soluble NgR peptide allows regeneration of myelinated axons into the spinal cord, restores connections with postsynaptic neurons and results in some degree of functional recovery (92). However, in other situations when the effect of growth inhibitors are blocked, this needs to be combined with a conditioning lesion of the dorsal root ganglion neurons (93, 94) or combined with potent immunostimulatory agents (95) in order to achieve significant regeneration into the spinal cord. One intriguing feature of the axon-glial interactions at the DRTZ after dorsal root injury is the formation of synapse-like contacts with DRTZ glial cell processes (96–98). The mechanism and significance of this process is still unclear, but its further studies may reveal novel information on the mechanisms preventing regenerating dorsal root axons from entering the spinal cord. Furthermore, exploring the interaction between regenerating dorsal root axons and glial processes my help to reveal novel processes that operate within the spinal cord to prevent long distance extension and/or plasticity of axons that attempt to grow or sprout after injury. In addition, the impact of pericytes and leptomeningeal cells in the emergence of a growth inhibitory environment at the DRTZ warrants exploration in view of their recently described roles in the formation of a scar after spinal cord injury (99, 100).
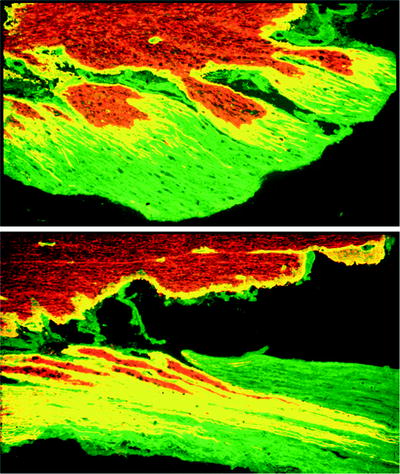
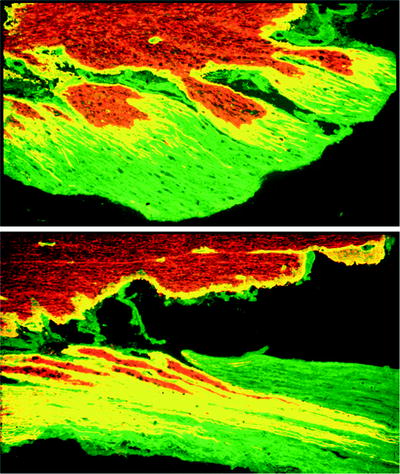
Fig. 2.
Lumbar dorsal roots following ipsilateral dorsal root injury. Horizontal section, immunostained with antibodies to laminin (green) and GFAP (red). Extensive outgrowth of astroglial processes (red and yellow) into the peripheral compartment is shown at 1 week (above) and 2 (below) weeks following injury (×200).
5 Dorsal Root Injury for Exploring Spinal Cord Injury Pathophysiology
Dorsal root injury is a useful model for exploring the process of axon and myelin breakdown and the associated non-neuronal responses in the spinal cord white matter. The dorsal root injury model is also useful for exploring non-neuronal responses and synaptic plasticity in the partially deafferented spinal cord. Whereas, these aspects are best approached after sectioning one or more dorsal roots, for translation to clinical root avulsion, models of dorsal root avulsion should eventually be the choice.
5.1 Dorsal Root Injury for Examining Mechanisms of Axonal Degeneration and Myelin Breakdown in the Spinal Cord
Section of one or more dorsal roots at the lumbosacral level is usually the best ones for studies on neuronal and non-neuronal processes associated with degeneration of the ascending axons in the dorsal column. An obvious advantage of this model is its absence of direct damage to the spinal cord, implying that its vascular supply and tissue architecture will be essentially untouched.
Axonal degeneration and secondary disintegration of myelin proceed from the injury area toward the spinal cord and along the axonal projections within the spinal cord although terminal parts of the axon appear to undergo degeneration even before their stem axon has disintegrated (101). Astrocytes and microglia undergo marked changes, and interact with infiltrating hematogenous cells in the removal of disintegrating axons and myelin, as well as in the formation of a tissue enriched in filamentous astroglial processes. Whereas axonal fragments are rapidly removed, myelin debris remains for long periods of time (102–104). In this context, important species variations exist. Remnants of myelin from degenerating heavily myelinated axons remain for decades in humans, and at least for years in, e.g., cats and rabbits. Astroglial processes may even be surrounded by myelin profiles in late stages of Wallerian degeneration in the cat spinal cord (105). One reason for this protracted presence of myelin debris may be an incomplete activation of the molecular mechanisms required for rapid myelin phagocytosis (106–108). The long-term presence of myelin-associated growth inhibitory molecules is likely to be a factor which counteracts growth of axons in the deafferented spinal cord (109). Thus, the dorsal root injury model can help to clarify the impact of the protracted Wallerian degeneration on axon growth in the spinal cord regeneration and possible ways to accelerate the elimination of myelin residues should this be beneficial. Recent studies, using dorsal root injury as experimental system, have also identified mechanisms involved in microglial-mediated clearance of axonal debris, a process which may also influence the conditions for regeneration in the spinal cord (110).
During Wallerian degeneration in the spinal cord white matter of monkeys abnormal profiles termed “myelinoclasts” appear (111). Similar structures were also observed in the cat (112, 113), but not in the rat (103). These profiles were characterized by layers of myelin wrapped around non-neuronal cells, presumably in most cases microglia, which at some later point in time appear themselves to undergo degeneration (113). Thus, the time course of myelin degradation and its associated microglial responses are distinctly different in small (e.g., rat, mouse) compared to large (e.g., cat, monkey, human) mammals, an observation which needs attention in translating findings from experimental models of spinal cord injury repair to humans.
< div class='tao-gold-member'>
Only gold members can continue reading. Log In or Register a > to continue
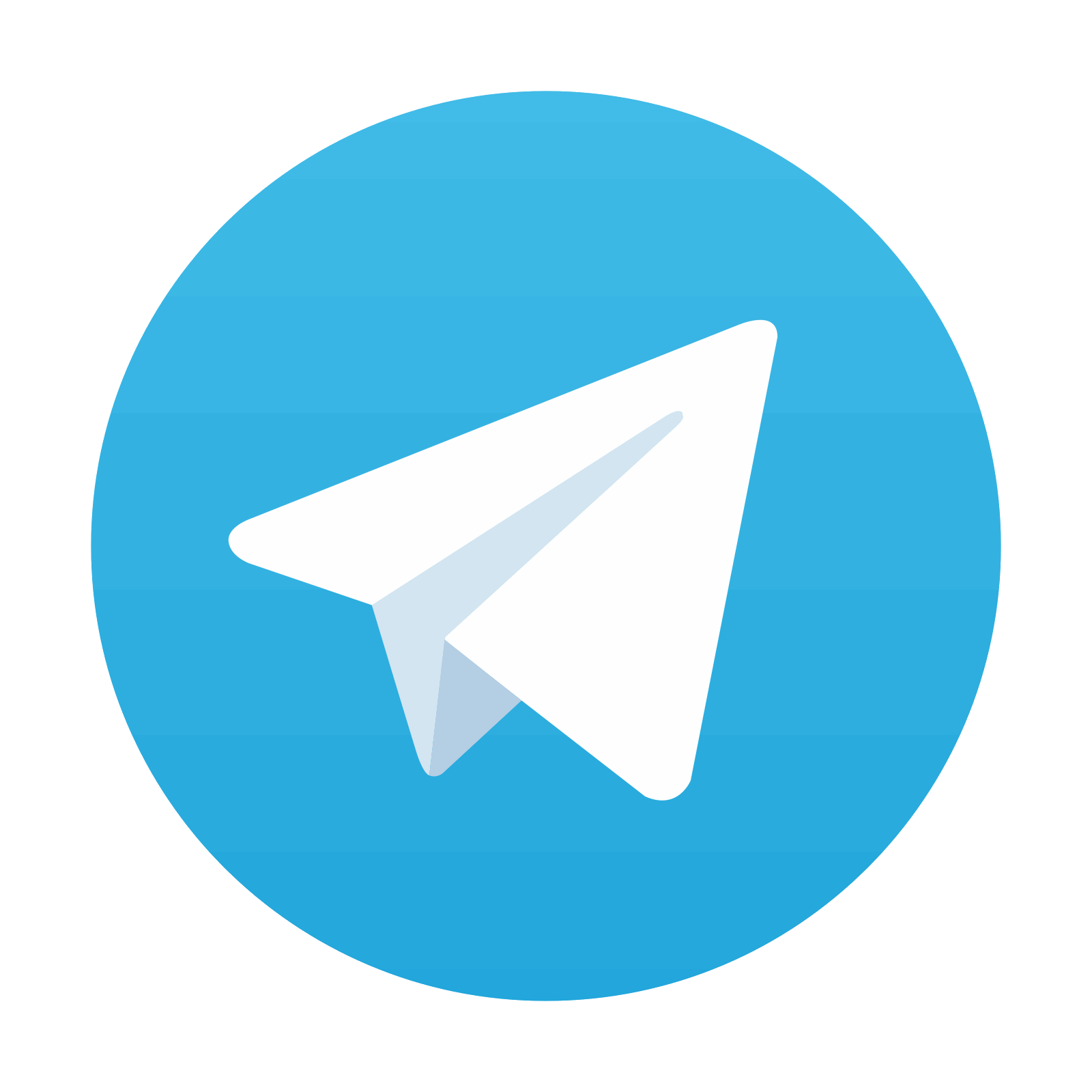
Stay updated, free articles. Join our Telegram channel

Full access? Get Clinical Tree
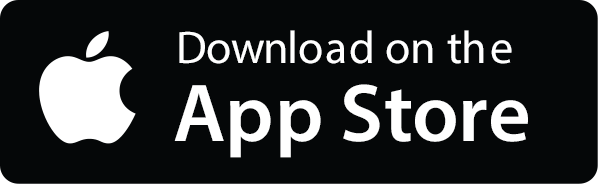
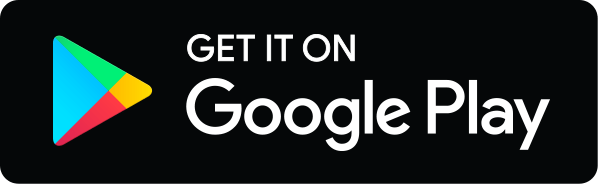