CHAPTER 20 Disorders of the Endocrine System
ADRENAL GLANDS
Unlike other species, maturation of the equine fetal adrenal glands occurs in late gestation and continues in the early postnatal period.1 Diurnal variations in cortisol concentrations are noted in adult horses2,3 and foals.4
ADRENAL INSUFFICIENCY
In adult horses, adrenal insufficiency is often associated with the discontinuation of glucocorticoids or anabolic steroid treatment.5,6 The adrenal gland response to exogenous ACTH in Thoroughbred horses was not altered by training,7 and low serum cortisol concentrations were not found in stressed racehorses with a history of poor performance.8 High plasma cortisol and ACTH concentrations were found in horses after maximal exercise.9–12 Endurance horses in a 160-km ride had significantly higher midride plasma cortisol concentrations than preride concentrations.13 A sixfold increase in cortisol secretion rate and a threefold increase in plasma cortisol concentrations was also found in exercising horses.14
Clinical signs include depression, anorexia, exercise intolerance, weight loss, mild abdominal discomfort, poor hair coat, lameness, and seizures.5,6,15,16 Serum biochemical analysis may be normal, or hyponatremia, hypochloremia, hyperkalemia, and hypoglycemia may be present.5,6,15
Adrenal insufficiency is rarely diagnosed in equine practice; however, it should be suspected in any horse with a history of anorexia, lethargy, poor body condition, poor exercise performance, glucocorticoid administration, electrolyte imbalances (hyponatremia, hypochloremia, hyperkalemia), and hypoglycemia. Baseline cortisol and ACTH concentrations, as well as the ACTH stimulation test, are critical to achieve a diagnosis. Cortisol has daily fluctuations, and single measurements do not provide enough information to make a diagnosis of hypoadrenocorticism. Therefore the ACTH stimulation test is more useful in assessing adrenal gland function.15,16 Horses with adrenal insufficiency have decreased cortisol concentrations and do not or minimally respond to the ACTH stimulation test.15,17 In addition, measuring ACTH concentrations is important to determine other causes of hypoadrenocorticism (iatrogenic, adrenal, pituitary). It is anticipated that exogenous glucocorticoids decrease ACTH concentrations (secondary hypoadrenocorticism), whereas adrenal gland dysfunction (primary hypoadrenocorticism) is associated with increased ACTH concentrations from decreased endogenous glucocorticoid negative feedback. In horses with increased plasma ACTH concentrations, the clinician must rule out pars intermedia dysfunction.
To perform an ACTH stimulation test, one administers 1 IU/kg of natural ACTH gel intramuscularly between 8 and 10 am.17 Immediately before ACTH injection, a blood sample is obtained in heparinized or plain tubes. One obtains additional blood samples 2 and 4 hours after ACTH administration. Horses with a functional adrenal gland should have two- to threefold increases in plasma cortisol concentrations after ACTH stimulation as compared with baseline values.6,17 To perform the cosyntropin stimulation test, one administers 100 IU (1 mg) of synthetic ACTH (cosyntropin; Cortrosyn) intravenously (IV) between 8 am and noon.18 One obtains a baseline, preinjection blood sample and a postinjection sample 2 hours later. As with the ACTH stimulation test, plasma cortisol concentrations should be at least twice baseline in the second sample. ACTH1-24 (25 IU) can also increase cortisol concentrations to twice baseline in horses.19
Treatment of hypoadrenocorticism involves rest and glucocorticoid supplementation. The duration and dosage of exogenous steroid treatment that induces adrenocortical atrophy in healthy horses is unknown. However, information on the doses that inhibit adrenocortical function in horses is available. Doses of dexamethasone as low as 4 mg suppress the HPA axis for up to 24 hours.6 Dexamethasone dosages of 0.044 to 0.088 mg/kg every 5 days for six treatments reduced cortisol concentrations for up to 4 days but did not change the response to ACTH stimulation.20 Toutain et al.21 found that dexamethasone (50 μg/kg, IV or IM) suppressed adrenocortical function for up to 4 days, prednisolone sodium succinate (0.6 mg/kg, IV or IM) for less than 24 hours, and prednisolone acetate suspension (0.6 mg/kg, IM) for up to 21 days. Dexamethasone (0.044 mg/kg, IM) and triamcinolone (0.044 mg/kg, IM) produced HPA axis suppression for 7 and 14 days, respectively.22 A single intra-articular dose of methylprednisolone acetate (200 mg) suppressed adrenocortical function for up to 10 days.23 Based on this information, prednisolone sodium succinate (up to 300 mg daily) is the first choice to treat hypoadrenocorticism; low doses of dexamethasone are the second option (triamcinolone and prednisolone acetate must be avoided). Alternatively, oral prednisolone (200-400 mg/horse) can be used. A case of hypoadrenocorticism secondary to prolonged anabolic steroid administration was successfully treated with 300 mg of oral prednisone for 9 months.5 Prednisone (40 mg, PO, b.i.d., or 50 mg, IV) was also used to treat a foal diagnosed with adrenal insufficiency.15 The efficacy of prednisone as a therapeutic agent in horses is questionable because prednisone in poorly absorbed from the equine intestinal tract and the active metabolite, prednisolone, is rarely produced.24 In contrast, oral prednisolone has excellent bioavailability and may be more useful as a therapeutic agent in horses. Like with any long-term glucocorticoid therapy, slow withdrawal is recommended.
ADRENAL INSUFFICIENCY IN CRITICALLY ILL FOALS
A substantial amount of information on the HPA axis of healthy and critically ill foals has been generated in recent years.1,4,25–28 In foals, HPA axis dysfunction has been defined as an inappropriately low basal serum cortisol concentration (absolute adrenal insufficiency), an inadequate cortisol response to the ACTH stimulation test (low delta cortisol), and an abnormally high ACTH/cortisol ratio (relative adrenal insufficiency [RAI]).4,25–27 For an accurate diagnosis of HPA dysfunction, results should be compared with values from healthy age-matched controls or to values generated in the same laboratory.
For many years it was proposed that adrenal insufficiency was a problem in premature and septic foals; however, not until recently was it demonstrated that dysfunction of the pituitary and adrenal glands is a frequent and serious problem in septic foals.25–27 Hart et al.26 found that 40% of septic foals had HPA axis dysfunction. Gold et al.25 determined the ACTH/cortisol ratio in septic foals and found that RAI was common, with nonsurviving foals having the highest ratio. In a similar study, Hurcombe et al.27 determined the AVP/ACTH and ACTH/cortisol ratios in septic foals and showed that HPA axis dysfunction at the pituitary and adrenal level was frequent in these foals. AVP is a major secretagogue for ACTH in the horse,29 and a high AVP/ACTH ratio suggests a decreased ACTH response to AVP stimulation (pituitary insufficiency). Similarly, a high ACTH/cortisol ratio indicates decreased adrenal response to ACTH (RAI). The low cortisol concentrations observed in premature foals do not appear to be the result of ACTH insufficiency because high ACTH concentrations have been measured in these foals,30 supporting adrenal insufficiency as a major problem in premature foals. A number of studies have found that septic foals with high baseline cortisol concentrations are more likely to die.25–27
To assess RAI in sick foals, a cosyntropin stimulation test was recently validated in healthy foals.4 A baseline blood sample is obtained for cortisol analysis. One then administers 10 μg (low-dose protocol) or 100 μg (high-dose protocol) of IV cosyntropin, and additional blood samples are collected at 30, 60, 90, and 120 minutes. Blood samples for cortisol concentrations can be collected in heparinized tubes or in tubes with no additives. With the 10-μg test, cortisol should peak at a value twice baseline at 30 minutes; with the 100-μg test, cortisol should peak at 90 minutes at two to three times the baseline value. The time of the day did not affect the results of this test.4 A simplified protocol would be to take baseline blood sample, administer 100 μg of IV cosyntropin, and collect second blood sample 90 minutes later. With this test it was found that a poor response to 100 μg of cosyntropin (0- and 90-minute samples) was associated with foal mortality.26
PHEOCHROMOCYTOMA
Pheochromocytomas are tumors that arise from the chromaffin cells of the adrenal medulla, which are part of the sympathochromaffin system (together with the sympathetic nervous system). During fetal life the chromaffin cells are associated with the sympathetic ganglia; after birth most of them degenerate, and the few remaining cells constitute the adrenal medulla. In human beings, approximately 90% of pheochromocytomas arise from the adrenal medulla, and often times are associated with a condition known as multiple endocrine neoplasia (MEN).31 Extra-adrenal pheochromocytomas have been described in human beings but not in horses. In horses these tumors have a low incidence of malignancy and predominantly are unilateral.32,33 Pheochromocytomas may be functional or nonfunctional. Functional pheochromocytomas secrete catecholamines at a rate sufficient to cause clinical signs.33 Thus functional pheochromocytomas are diagnosed with more frequency than nonfunctional pheochromocytomas.34 It is speculated that most equine pheochromocytomas are nonfunctional and go undiagnosed.35 Both epinephrine and norepinephrine have been identified as the catecholamines produced by equine pheochromocytomas.36
Functional pheochromocytomas in horses have no breed or gender predilection33 and have been described in horses older than 12 years.34,37 Because clinical signs are of acute onset and of rapid progression from intense adrenergic stimulation, the clinical signs resemble those of colic, rhabdomyolysis, acute laminitis, enterocolitis, and hyperkalemic periodic paralysis. Clinical signs include abdominal pain from large hematomas or gastrointestinal distension secondary to ileus, anxiety, tachycardia, tachypnea, profuse sweating, muscle tremors, hyperthermia, dry and pale mucous membranes, increased capillary refill time, ataxia, and mydriasis.33,34,37 Abortion has been documented. Clinical signs from nonfunctional pheochromocytomas in general are consistent with abdominal pain.33,35,37
Hematologic abnormalities of functional pheochromocytomas include hemoconcentration, stress leukogram (mature neutrophilia with lymphopenia),37 and leukopenia with neutropenia.34 Hemoconcentration likely results from epinephrine-induced splenic contraction rather than dehydration.34,35,37 Epinephrine and epinephrine-induced steroid release may be responsible for the stress leukogram. Serum biochemical abnormalities are nonspecific and include azotemia, metabolic acidosis, hyperglycemia, hyponatremia, hyperkalemia, hypocalcemia, and hyperphosphatemia.33,37 Hyperglycemia is likely a consequence of increased α- and ß-adrenergic activity.31 Hyponatremia with hyperkalemia, hypocalcemia, and hyperphosphatemia suggests acute renal failure; however, renal function in most reported cases was normal.34,35 Hyperkalemia, hypocalcemia, and hyperphosphatemia could be the result of prolonged muscle activity and ischemia.33–3537 Hyperkalemia is unlikely to be from increased catecholamine concentrations because catecholamines induce hypokalemia by shifting potassium to the intracellular compartment (ß-receptor mediated) and by increasing the activity of the renin-angiotensin system.38 Glucosuria is also a frequent finding. Pheochromocytomas may secrete other hormones of neuroendocrine origin (calcitonin [CT], parathyroid hormone [PTH], ACTH, corticotropin-releasing hormone, somatostatin (SST), vasoactive intestinal peptide, leu-enkephalin);34,39–41 however, this remains to be determined in horses.
The clinician should be aware that this condition carries a poor prognosis, and euthanasia may be a reasonable decision. Measurement of blood catecholamines or their metabolites in urine is valuable in establishing a diagnosis; however, this assessment can be a challenge, and most laboratories do not measure these hormones.33,34,37,42 Ultrasonography and measurement of blood pressure (BP) can support the diagnosis.
If a diagnosis is reached, adrenalectomy can be attempted; however, the location of the adrenal glands, as well as the proximity of major blood vessels, tumor size, and arrhythmias (interaction between catecholamines and anesthetic agents),35 make this a difficult decision.33,35,37 Hypotensive α-adrenergic antagonists such as phentolamine, phenoxybenzamine hydrochloride, and prazosin hydrochloride are used in human beings with functional pheochromocytomas before surgery.
Functional pheochromocytomas in horses do not appear to metastasize, are unilateral, tend to bleed, and at necropsy the tumor is frequently found to be ruptured. Myocardial infarction and degeneration may be present, probably the result of increased concentrations of catecholamines.33–35
Malignant pheochromocytomas have been documented in young and old horses.43,44 A malignant pheochromocytoma was reported in a 6-month-old filly with a history of hindlimb lameness and spinal cord disease.44 Both adrenal glands had multiple and well-circumscribed yellow masses, and metastases were found in the liver, lungs, vertebral canal, left scapula, and azygous vein. A malignant pheochromocytoma was also diagnosed in a 22-year-old mare that died of massive intrauterine hemorrhage.43 This mare also had a thyroid C cell medullary adenoma and bilateral nodular hyperplasia of the adrenal medulla, findings that were consistent with MEN. This was the first documented case of MEN in the horse, although previous reports have been consistent with MEN.45
HYPERADRENOCORTICISM
Hyperadrenocorticism from pituitary pars intermedia dysfunction (PPID; equine Cushing’s disease) is discussed elsewhere in this text. Iatrogenic and adrenal hyperadrenocorticism are less common than pituitary-dependent hyperadrenocorticism (PDH) but have been described in horses.46–48
Iatrogenic hyperadrenocorticism was induced in a horse treated for a pruritic skin condition, after injecting 12 mg of triamcinolone acetonide followed by two injections of 200 mg of the same compound, all within 6 weeks.46 Clinical signs included depression, polyuria-polydipsia, weight loss, and laminitis. Blood and urinalysis abnormalities (neutrophilia, lymphopenia, hyperglycemia, glucosuria) were similar to those of PPID. However, this horse also had increased serum activity of γ-glutamyltransferase (GGT), aspartate transaminase, and bile acid concentrations, indicating steroid-induced hepatopathy.
One case of pituitary-independent (adrenal dependent) hyperadrenocorticism has been documented in the literature.48 In this case, a 12-year-old gelding displayed clinical signs of PDH. Baseline cortisol and ACTH concentrations were within the normal range, and the cortisol response to the ACTH stimulation test was normal. The horse was treated with bromocriptine with no improvement; over the next 11 months the clinical signs worsened. On necropsy, an adrenocortical adenoma associated with pituitary gland and contralateral adrenal gland atrophy was present.
ADRENOCORTICAL NEOPLASIA
Adrenocortical tumors are rare in horses,19,48–50 and the few cases reported in the literature were nonfunctional tumors. One case of a 12-year-old Dutch Warmblood gelding with a functional adrenocortical adenoma and clinical signs consistent with Cushing’s disease (hyperadrenocorticism) was recently reported.48
THYROID GLAND
In the horse, the thyroid glands are two discrete, firm lobes located in the dorsolateral aspect of the third to sixth tracheal rings.1,2 The two lobes are connected by a narrow isthmus that contains fibrous tissue. In most healthy horses the thyroid glands are not visible but can be palpated as firm and movable structures. The weight of the thyroid glands relative to body weight is largest for fetuses and foals (mean, 0.28 g/kg; range, 0.12 to 0.67) and decreases with age; the ratio for adults is 0.08 g/kg (range, 0.01 to 0.15).3 The normal total gland weight for newborn foals is around 15 g.4 The glands are very vascular, receiving blood supply from two major arteries that arise from the external carotid and subclavian arteries. Thyroid gland blood supply is relatively greater (4 to 6 ml/min/g) than other highly vascular organs. Gland size does not parallel function, and functional tests must be used to establish an accurate diagnosis of thyroid dysfunction.
THYROID HORMONE FUNCTION
Understanding TH functions is important for the diagnosis and treatment of thyroidal and nonthyroidal diseases (Box 20-1). THs (triiodothyroidine-T3, thyroxine-T4) are important in cell growth, differentiation, and metabolism in nearly all tissues.
BOX 20-1 COMMON ABBREVIATIONS ASSOCIATED WITH THE THYROID GLAND
TH | Thyroid hormone |
HPT axis | Hypothalamus-pituitary-thyroid axis |
TSH | Thyroid-stimulating hormone |
DA | Dopamine |
SST | Somatostatin |
NIS | Na+/ I− symporter |
MIT | Monoiodotyrosine |
DIT | Diiodotyrosine |
T4 | Thyroxine |
T3 | Triiodothyronine |
TBP | Thyroid-binding proteins |
TBG | Thyroid-binding globulin |
TBPA | Thyroid-binding prealbumin |
NTIS | Nonthyroidal illness syndrome |
MEN | Multiple endocrine neoplasia |
TRH | Thyrotropin-releasing hormone |
The synthesis and secretion of THs is regulated by a negative-feedback system that includes the hypothalamus, the pituitary gland, and the thyroid gland (hypothalamus-pituitary-thyroid [HPT] axis). TH synthesis and secretion is stimulated by thyroid-stimulating hormone (TSH, thyrotropin), which is a glycoprotein with two subunits (α and ß) secreted by the thyrotropes of the anterior pituitary gland. The α subunit of TSH is shared with other pituitary hormones (luteinizing hormone, follicle-stimulating hormone). TSH secretion is controlled by hypothalamic neurons that release a tripeptide, thyrotropin-releasing hormone (TRH).5 TSH synthesis and secretion is also under the control of the negative feedback of THs.6 The hypothalamus can also inhibit TSH secretion through dopamine (DA) and SST.7 In the pituitary, T3 decreases the TSH response to TRH stimulation, and in the hypothalamus, T3 decreases the mRNA expression and secretion of TRH.8–10 In the pituitary gland and central nervous system (CNS), a type II deiodinase converts T4 to T3. For T4 to gain access to hypothalamic neurons it must be transported through the choroid plexus to the lateral ventricles bound to transthyretin (T4-binding protein). In various species, including the horse, TRH induces prolactin release.11–14
Thyroid Hormone Metabolism
A major function of the thyroid gland is to actively trap and conserve iodine against a large concentration gradient.1,7,15 Iodine can be absorbed in its soluble form, iodide (I−), via the intestinal mucosa or any moist body surface or broken skin.1 Iodide is actively transported and concentrated into the thyroid by a Na+/ I− symporter (NIS).16 The trapped iodide is oxidized by a thyroid peroxidase and incorporated into the tyrosine residues of thyroglobulin to form the inactive precursors, monoiodotyrosine (MIT) and diiodotyrosine (DIT). Coupling of these precursors results in the synthesis of thyroxine (T4) and triiodothyronine (T3). The iodinated thyroglobulin containing MIT, DIT, T3, and T4 is stored as an extracellular polypeptide in the colloid of the thyroid follicles.17 When needed, T4 and T3 are cleaved from the thyroglobulin, picked up by the follicular cells via endocytosis, and transported into the circulation.1,7 Most of the TH released into circulation is T4, because total serum T4 concentrations are 20 times higher than serum T3. Hormone synthesis and release are controlled by TSH and iodine availability. The feedback regulation of TSH secretion is closely related to the circulating concentrations of unbound (free) THs (fT4 and fT3).1,7
The circulating THs are T4, T3, and reverse T3 (rT3). Free T4 and T3 are the biologically active hormones. T3 and rT3 are both deiodination products of T4. All of the circulating T4 is directly derived from the thyroid gland, whereas only 10% to 20% of the circulating T3 is directly secreted from the thyroid gland. The major pathway for the production of T3 is the 5’-monodeiodination of T4 by a type I deiodinase (a selenoprotein) in peripheral tissues. This is referred to as T3 neogenesis. In the fetus, type I deiodinase activity is delayed, so T3 neogenesis is low, and most deiodinase activity results in high concentrations of rT3. A switch in enzymatic activity near birth, partly the result of the influence of glucocorticoids, leads to high T3 concentrations in newborns, including foals.7,18–22 THs circulate bound to plasma proteins (thyroid-binding proteins [TBP]). Thyroxine-binding globulin (TBG) is the major protein (70% of T4 and T3 are bound to TBG); transthyretin (thyroid-binding prealbumin [TBPA]), and albumin bind to a lesser degree. In horses, the percentages of circulating T4 bound to TBG, TBPA, and albumin is 61%, 22%, and 17%, respectively.23 T3 is bound to TBG and albumin but not to TBPA. Transthyretin is important to transport T4 into the CNS. As THs are reversibly bound to transport proteins they act as TH reservoirs. T4 has much greater protein-binding affinity and considered a prohormone. Only free, unbound hormones cross the capillary endothelium to exert their biologic functions.24 T3 is much more potent than T4, and it has a shorter half-life. The half-life of T4 in horses is approximately 50 hours.25 Because a large fraction of THs are bound to plasma proteins, changes in protein concentrations may change total TH concentrations.
The enzymes responsible for the production of T3 and rT3 are also responsible for their inactivation. Three classes of 5′-deiodoninases exist. Type I deiodinase is predominantly found in peripheral tissues such as thyroid, kidney, and liver, and is responsible for the conversion of most of T4 to T3 and rT3, and is inhibited by propylthiouracil (PTU). Type I deiodinase activity is increased by TSH and T3, and in hyperthyroidism, and it is decreased in hypothyroidism. Type II deiodinase is found in brown fat tissue, brain, and pituitary gland, is not affected by PTU,7 and its primary function is to convert T4 to T3 in the intracellular compartment.17 Type II deiodinase activity is increased in hypothyroidism, likely to sustain intracellular T3 concentrations despite low peripheral T4 concentrations, especially in the nervous system. Type III deiodinase is found in the placenta, developing brain, and skin, and its primary function is to inactivate T4 and T3 (converts T4 to rT3, inactivates T3) to regulate T3 availability during development. The generation of inactive rT3 by type I and III deiodinases is essential in the regulation of TH function. Metabolites are excreted via the urine, and some are conjugated and enter the enterohepatic circulation. Most iodide is returned to the thyroid gland.
The thyroid gland is also the site of production of CT, secreted by the parafollicular cells (C cells). CT is involved in calcium homeostasis.1,7,26
Molecular Actions of Thyroid Hormones
The TH receptors belong to the superfamily of nuclear receptors that work as transcription factors. Two types of TH receptors exist (TR-α and TR-ß). T3, directly transported into the cell or derived intercellularly from T4, is the effector hormone. TH receptors interact with specific DNA sequences to regulate gene expression. Growth, thermogenesis, and energy metabolism depend on THs. T3 stimulates thermogenesis, oxidative phosphorylation, oxygen consumption, protein synthesis, metabolic rate, carbohydrate absorption, glucose metabolism, lipid metabolism, lipoprotein lipase, lipolysis, and the conversion of cholesterol into bile salts. T3 is also important for growth, maturation, and erythropoiesis, stimulation of heart rate, cardiac output, and blood flow, as well as critical for cerebral and neuronal development in young animals.1,15 T3 decreases the expression and synthesis of TSH and TRH.
FACTORS THAT AFFECT THYROID GLAND FUNCTION AND TESTING
Age
Age-related differences exist in TH concentrations in horses. Late in gestation a powerful central drive exists to stimulate the HPT axis.24 Serum concentrations of T3 and T4 in foals are very high at birth and decrease with age,18–22,27,28 with most of the decline occurring in the first 3 to 4 months of life.20,29 Total T4, fT4, total T3, and fT3 concentrations in the umbilical cord blood of newborn foals were found to be 14, 5, 7, and 3 times the adult concentrations, respectively.24 At birth, plasma rT3 declined rapidly as T3 concentrations rose for the first 24 to 48 hours after birth.24 Another study found that plasma T3 concentrations decreased from 7.9 ng/ml at birth, to 0.9 ng/ml at 6 months, to 0.7 ng/ml at 9 months old.22 Plasma T3 concentration in mares was 0.5 ng/ml. In the same study, T4 concentrations decreased from 233 ng/ml at birth to 49 ng/ml at 14 days and to 35 ng/ml at 6 months. Thus TH concentrations in newborn foals are ~10-fold higher than in adult horses, and TH concentrations in the range of adults may indicate a hypothyroid state.
Gender and Breed
A clear association does not exist between gender and TH concentrations. Various studies have found higher concentrations in male horses,18,30,31 lower concentrations in stallions and geldings,28,32 or lower concentrations in mares than in stallions,30 whereas others have found no differences.29,33–35 No evidence indicates that TH concentrations differ among breeds.18,35
Physiologic Status
Mares tested at all stages of the estrous cycle had no differences in TH concentrations.34 T4 concentrations, however, were lower after ovulation, which is the opposite pattern seen in other species. Higher TH concentrations have been reported in pregnant mares,36 which is similar to other species.7 This may be due in part to the increased TBG concentrations during gestation.7 T4 concentrations were statistically higher between days 49 and 55 of gestation as compared with mares in advanced pregnancy; however, T3 did not vary with gestation.37 Other studies found no differences between pregnant and nonpregnant mares.25,32
Hormones
Exogenous and endogenous glucocorticoids suppress pituitary TSH secretion, decrease the response of TSH to TRH, inhibit deiodination (except in the perinatal period where the conversion of T4 to T3 in enhanced), and decrease TH release.7,38 Glucocorticoids decrease serum T4 and T3 concentrations, with the effect being more profound on T3.6 Dexamethasone administration to healthy horses for 5 days did not change total T3 and total T4 concentrations, but it increased serum rT3 and fT3 concentrations and blunted the fT3 response to TSH stimulation.39 Catecholamines enhance the rate of deiodination of T4 to T3, and have potentiating effects.40 Estrogen enhances TBG production, whereas androgens tend to suppress it.7 Oral administration of levothyroxine to healthy horses blunts the TSH and TH response to TRH stimulation.41
Season and Daily Rhythm
Cold temperatures stimulate and high temperatures inhibit the HPT axis.25,42,43 A daily rhythm in TH concentrations has been identified in horses.44,45 In geldings, T4 peaked around 4 pm (2.4 ± 0.8 μg/dl), with the lowest T4 values around 4 am (1.8 ± 0.6 μg/dl). T3 peaked between 8 am and 4 pm (54 ± 13 ng/dl), with the lowest T3 values around midnight (38.7 ± 10.8 ng/dl).44 Higher T4 concentrations between 5 and 8 pm, with lowest concentrations at 8 am were reported in another study.45 Diurnal variations in glucocorticoid concentrations appear to control the diurnal variations in TH by suppressing the pituitary response to TRH.46
Diseases: Nonthyroidal Illness Syndrome
Stress, illnesses, systemic inflammation, and starvation suppress the HPT axis. Because no clinical evidence indicates that low TH during disease is directly associated with hypothyroidism, this condition has been called the low T3 syndrome or the euthyroid sick syndrome. The designation nonthyroidal illness syndrome (NTIS) has been proposed because it does not presume the metabolic status of the patient.47 In this syndrome, T3 concentrations are decreased, whereas T4 concentrations can be low, normal, or elevated. In critically ill human patients, a significant decrease in T4 and T3 or an increase in rT3 concentrations is associated with increased mortality rate.47 Low TH concentrations were found in premature and septic foals,48 confirming that NTIS occurs in newborn foals. Low TH concentrations are a common finding in obese horses with insulin resistance (IR) (metabolic syndrome). Hypothalamic and pituitary dysfunction appears to be the proximal cause of NTIS,47 because administration of TRH to patients with NTIS increases TSH, T4, and T3 release.49 Although NTIS is a hypothyroid state, no evidence indicates that target tissues are chemically hypothyroid.47
Cytokines, glucocorticoids, and more recently, leptin are the key candidates in the pathogenesis of NTIS. Cytokines such as IL-1, IL-6, and TNF-α suppress TSH, T4 and T3 synthesis and secretion. IL-1 impairs TH synthesis, whereas IL-6 and TNF-α decrease T4 and T3 concentrations and increase rT3 concentrations. Glucocorticoids are negative regulators of thyroid function; increased cortisol concentrations can be present in horses under different conditions, including stress, sepsis, obesity, IR, and pituitary gland dysfunction (Cushing’s disease), and low TH concentrations can be measured in the same animals. The role of leptin in the pathogenesis of NTIS relates to caloric intake. A decline in energy intake decreases leptin concentrations, which reduces the hypothalamic synthesis of TRH.50 No clear evidence indicates that TH replacement therapy in human beings or animals with NTIS is either beneficial or harmful. Some believe that replacement therapy may be harmful because exogenous TH may inhibit the HPT axis. The only clear indication for exogenous supplementation of THs in horses is to induce weight loss and enhance insulin sensitivity in obese animals, in particular those with metabolic syndrome.51
Activity
Several studies have found increased T4 concentrations in horses in training.19,52,53 Another investigator documented increases in T4 within 30 minutes after exercise, with no differences in T3.33,36 Five minutes after a maximal speed race (1200 m), serum T3 concentrations increased in Thoroughbred racehorses (from 55.6 ± 2.9 to 81.0 ± 3.7 ng/dl); however, T4 concentrations did not change (0.67 ± 0.04 to 0.70 ± 0.05 μg/dl).54 Significant increases in T3 and T4 concentrations were found 1 hour after swimming.55
Feeding
Feeding a high-energy and high-protein diet to weanlings stimulated TH secretion with a subsequent decrease 3 hours after each meal.56 Soluble carbohydrates (glucose) rather than protein appear to be responsible for the TH increase in weanlings.57 The conversion of T4 to T3 after a carbohydrate meal was associated with insulin release.58 This response to carbohydrates is age dependent; by the time horses were 12 to 14 months old, feeding high-carbohydrate and protein diets had minimal effect on TH concentrations.58 One study in ponies found greater T3 concentrations when the animals were fed once a day rather than six times a day.59
Starvation in different species, including the horse, decreases T3 and fT3 concentrations.47,60,61 Carbohydrate deprivation inhibits the deiodination of T4 to T3 (by the type I deiodinase) to decrease T3 and increase rT3 concentrations. Because starvation decreases the basal metabolic rate, the decrease in TH concentrations appears to be an adaptive process to conserve energy. In these animals, TSH concentrations are low or within the normal range despite low TH concentrations. When the effect of food deprivation on baseline TH concentrations was evaluated in euthyroid horses,61 it was found that serum T3, fT3, and fT4 concentrations decreased, whereas rT3 concentrations increased after 1 day of food withdrawal. Serum T3 concentrations were lowest after 2 days of deprivation, whereas T4, fT3, and fT4 reached their lowest concentrations on day 4 of deprivation. Three days after the horses were placed on their normal ration, serum T4 and fT4 concentrations increased significantly, and rT3 concentrations decreased.61 Prolonged food restriction to Shetland ponies also decreased serum T3 and fT3 concentrations.62 These studies provided valuable information in the interpretation of TH concentrations in anorectic and sick horses. If a decrease (>50%) in serum T3 and T4 concentrations can be present in 1 to 2 days of food deprivation, then the validity of measuring TH in diseased horses is questionable.
The mechanisms that regulate thyroid gland function during starvation remain unclear. The adipocyte-derived hormone leptin, which is the main regulator of hunger, is the critical neuroendocrine signal that regulates the HPT axis during fasting and disease.63 Leptin concentrations decrease during starvation to increase appetite and conserve energy. Feed restriction decreases and excessive caloric intake increases leptin concentrations in horses,64–66 with THs following similar patterns.47,60,61 Even though information on leptin has been published in horses and foals,64,67,68 its direct role on thyroid gland function is unknown.
Exogenous Compounds
Goitrogenic substances such as thiocyanate and perchlorate compete with iodide uptake. Iodide oxidation and coupling of iodotyrosines are inhibited by drugs such as sulfonamides, phenylbutazone, phenothiazines, thiouracils, thiopental, and methimazole. A recent study found no effect of trimethoprim-sulfadiazine on equine thyroid function.69 Soybean meal, linseed meal, and plants of the Brassicaceae family. (i.e., rapeseed) contain goitrogenic substances.1,15 Phenylbutazone administration decreases TH concentrations in horses,45,70 and this effect can last up to 10 days after discontinuation of phenylbutazone administration.70 The TSH stimulation test may be normal or exacerbated in horses receiving phenylbutazone.45 High levels of nitrates in the water may induce thyroid gland hypertrophy,71 because nitrates reduce the iodine transport into the thyroid gland.
PTU, in addition to affecting iodine metabolism, inhibits the peripheral conversion of T4 to T3. In human beings, aspirin competes for TH binding sites on transthyretin and TBG, decreasing T4 and T3, and increasing fT4 and fT3. This results in a hypermetabolic state from the increased free fractions of T4 and T3.38 Anabolic steroids can decrease TBG concentrations7; however, short-term administration of anabolic steroids to horses did not affect thyroid function tests.72 Exogenous compounds containing iodine or iodides are of particular interest. Iodine excess, as well as deficiency, can induce thyroid dysfunction and interfere with thyroid tests. Horses are exposed to iodine or iodide compounds in the form of feedstuffs, expectorants, leg paints, shampoos, injectable counterirritants, radiographic contrast media, and antiprotozoal drugs (iodochlorhydroxyquin). Animals can respond to excessive amounts of iodine by either suppressing or accelerating hormone production.
Iodine excess reduces iodine uptake and organification, decreases TH concentrations and the response to the TSH stimulation test, and inhibits the cleavage of T4 and T3; this phenomenon is known as the Wolff-Chaikoff effect. The end result of iodine excess may be hypothyroidism. The second way an animal can respond to large amounts of iodide is by elevating TH production. This is referred to as Jod-Basedow phenomenon, and in general it is seen in individuals with iodine deficiency.7
Newborn foals of mares grazing Neotyphodium coenophialum-infected fescue had lower T3 concentrations than controls,73 with no differences in T4 and rT3 concentrations. Endophyte alkaloids are dopaminergic D2-receptor agonists, and DA is an inhibitor of TSH secretion. It is not clear whether the low TH concentrations in these foals is the direct effect of endophyte alkaloids on the fetal HPT axis or on placental and fetal development. Endophyte alkaloids do not appear to affect thyroid function in adult horses.74
DIAGNOSTIC TESTS
Unlike human practice in which a combination of functional and direct measurements of THs are often used, in equine medicine clinicians are far from assessing thyroid gland function in an organized manner. The traditional approach is to determine TH concentrations (compared with reference values) and make a diagnosis and specific recommendations, in many cases ignoring the underlying cause of abnormal TH concentrations. Normal TH concentrations have a wide range of values and vary among laboratories and measurement techniques (Tables 20-1 and 20-2). For consistent results the practitioner should use the same laboratory. Ratios of circulating hormones may be indicative of dysfunction.
The T4 secretion rate (TSR) is a functional test that calculates T4 secretion after83 iodide injection. This method is not clinically practical.19
Immunoassays are currently used to measure total T4 and fT4 concentrations. Equilibrium dialysis is the most accurate method to measure the free fractions of THs; however, it is being replaced by immunoassays. Several radioimmunoassays (RIAs) are validated for equine TH concentrations.35,84 Quantification of T4 and T3 with RIA is not affected by hemolysis.85 Enzyme-linked immunosorbent assays (ELISA) and immunoluminescence methods are also available. The unbound or free fractions of THs must be measured to determine available hormone to target tissues.86
In various species the ratio of circulating total T4 to T3 is 20:1. In horses, the T4/T3 ratio is 23:1 and the fT4/fT3 ratio is 1.83:1.31 The concentrations of rT3 are often increased in hyperthyroidism and NTIS.7
Commercial assays to determine TSH concentrations in human beings and dogs are available. The validity of human TSH assays to measure equine TSH is unknown; however, it is expected to be acceptable based on a 90% homology between human and equine TSH. Increased TSH concentrations are expected in hypothyroidism, and the opposite is expected in hyperthyroidism.14,79 Equine TSH concentrations have been determined using customized assays.48,68,69,76,79 Breuhaus76 validated an equine-specific double-antibody TSH RIA in an equine model of hypothyroidism. In that study, hypothyroidism was induced in healthy horses by administering PTU for 6 weeks. Serum T3 and fT3 fell rapidly, whereas fT4 and T4 did not decrease until weeks 4 and 5, respectively. TSH concentrations remained steady until week 5, increasing thereafter. The TSH response to TRH stimulation (1 and 5 mg) was exaggerated. Similar studies of PTU-induced primary hypothyroidism found a decrease in serum of T3, T4, and rT3, as well as an increase in TSH concentrations.14,79
Thyroid-Stimulating Hormone Stimulation Test
The TSH stimulation test consists of injecting 2.5 to 5.0 IU of TSH, IV and comparing pre- and postinjection TH concentrations.87,88 In normal horses, T4 peaks 3 to 4 hours after injection at a concentration 2.4 times the preinjection value. T3 doubles within 30 minutes and peaks at 2 hours at a concentration five times baseline.89 Normal responses are characterized by a rise in T3 that antedates the T4. When 5 IU of TSH were given intramuscularly to horses, T4 peaked at two times baseline value 3 to 12 hours after injection, and T3 peaked in 1 to 3 hours.45 No differences in T4 concentrations were found between 2.5 and 5 IU of TSH.87 The suggested protocol for the IV TSH response test is to measure TH concentrations at baseline and 3 to 4 hours after injection.89 For the intramuscular protocol, hormone concentrations are measured at baseline and 3 and 6 hours after injection.45 The protocol for 1-day-old foals is to measure T3 concentrations at baseline and 1 and 3 hours after the IV injection of 5 IU of TSH. A normal response is a 50% increase by 3 hours. T4 values after TSH stimulation are variable in foals.81 An insufficient hormonal increase indicates primary hypothyroidism. The ability to perform a TSH stimulation test may be limited by the cost or availability of TSH.
Phenylbutazone decreases TH concentrations in horses, but it does not affect the response of the TSH stimulation test.45 Dexamethasone blunts the TH response to the TSH stimulation test.39 This information should be taken into account in the interpretation of the TSH stimulation test.45,89 Treatment with DA agonists does not appear to affect serum T3, T4, rT3, or TSH concentrations in horses.79
Thyrotropin-Releasing Hormone Stimulation Test
The TRH stimulation has become a widely used method to assess thyroid gland function and pituitary gland dysfunction in horses.87,90–95 TRH is administered IV, 1 mg to horses and 0.5 mg to ponies and foals, with hormone measurements at baseline and 4 to 5 hours later. In a normal response both hormones should increase two- to threefold. An inadequate hormone response to TRH would occur in primary or secondary hypothyroidism. In normal animals, T4 peaks at 4 hours87,92 and T3 at 2 hours after TRH administration.92 Side effects of TRH administration include salivation, urination, defecation, vomiting, pupillary constriction, tachycardia, and tachypnea.91 A low response to TRH administration suggests dysfunction at either the pituitary or the thyroid gland level. Additional tests (TSH concentrations, TSH stimulation test) may be necessary to differentiate thyroid from pituitary disease. Low TH and high TSH concentrations, and a low response to the TSH stimulation test suggest thyroid gland dysfunction. Low TH concentrations with low or normal TSH concentrations suggests hypothalamic or pituitary gland dysfunction. Low TH and TSH concentrations with a normal TSH and TH response to TRH stimulation indicates hypothalamic dysfunction. Levothyroxine administration blunts the response to the TRH stimulation test.41
Thyroid Suppression Test
TSH-independent secretion of THs can be demonstrated by the thyroid-suppression test. Exogenous administration of TH to suppress TSH secretion should also decrease TH secretion. The lack of TH suppression indicates primary hyperthyroidism. After T3 administration, healthy horses are expected to have a decrease in T4 concentrations, whereas no changes in T4 concentrations are anticipated in hyperthyroid animals. This test was used to diagnose hyperthyroidism in a horse with a thyroid adenoma96; 2.5 mg of T3 were administered intramuscularly, and serial sampling revealed no decrease in T4 concentrations.
Imaging
Nuclear scintigraphy (technetium-99m) is useful in assessing equine thyroid gland function.97Abnormal uptake patterns have been seen with thyroid carcinoma in horses.98–100 Ultrasonographic evaluation of the glands can differentiate solid structures from cystic ones.7 Ultrasonography is the most commonly used imaging modality to assess equine thyroid gland morphology.96,101
HYPOTHYROIDISM
Hypothyroidism is defined as TH deficiency, a deficient thyroid activity, or disruption of the HPT axis. Hypothyroidism occurs from diseases that affect thyroid gland function, exogenous compounds that interfere with TH synthesis, and less frequent by disorders of the hypothalamus or pituitary gland. The liver, muscle, skeleton, kidney, heart, salivary glands, pancreas, and CNS during development are target organs for THs.7 Hypothyroidism manifests in many ways, and the diagnosis can be a challenge because many nonthyroidal factors can lead to misinterpretation of the results. Hypothyroidism can be classified as primary, secondary or tertiary. Primary hypothyroidism can be caused by iodine deficiency (endemic goiter) or excess (Wolff-Chaikoff effect), thyroiditis, neoplasia, biochemical defects, thyroid agenesis, or ingestion of goitrogenic compounds that block hormone synthesis. Of these, iodine excess, iodine deficiency, and neoplasia have been reported as causes of equine hypothyroidism. Histological lesions consistent with Hashimoto thyroiditis were recently described in horses.102
Horses with primary hypothyroidism have decreased T4 and T3 concentrations and increased TSH concentrations.76 Secondary (central) hypothyroidism occurs from pituitary or hypothalamic dysfunction. Reports exist of low TH concentrations in horses with pituitary adenoma.90,103,104 Tertiary hypothyroidism denotes a defect in TH use, but it has not been reported in horses.
Hypothyroidism in Foals
The consequences of inadequate circulating TH can be devastating for the developing fetus and newborn foal. Chances for recovery are unlikely once critical developmental stages are passed. Tests to evaluate thyroid gland function may be within normal limits at the time of examination, making it difficult to confirm a previous transient or in utero TH deficiency.24 The equine placenta is permeable to iodine, but impermeable to T3 and T4, and excessive ingestion of iodine may result in hyperplastic goiter (Figure 20-1, A and B).
Signs
Because decreased iodine intake is considered the main cause of neonatal hypothyroidism and goiter, pituitary gland function in these foals may be normal or increased. The lack of pituitary inhibition from low TH concentrations leads to excessive TSH secretion, thyroid follicular cell hyperplasia, and thyroid enlargement (goiter). The clinical signs reported in hypothyroid foals relate to the crucial role of THs in development and maturation of the nervous, respiratory, and musculoskeletal systems during the pre- and postnatal periods. Hypothyroid foals are often affected at birth. Clinical signs reported in hypothyroid foals include stillbirths, prematurity, weakness, and long hair coat4,105; weak suckle reflex106; respiratory insufficiency and distress107,108; incoordination20; lethargy, depression, and rough coat109; cold intolerance and hypothermia24; physeal dysgenesis110; defective ossification with tarsal bone collapse110,111; hypoplastic carpal bones, common digital extensor tendon rupture, forelimb contracture, and mandibular prognathism112,113; delayed incisor eruption, growth retardation, and death.114 Foals can be born apparently normal but may develop skeletal lesions weeks later.109
Cause
The major cause of hypothyroidism in foals is nutritional. Congenital thyroid enlargement (congenital goiter) with decreased thyroid function is associated with both inadequate and excessive iodine intake by the mare. Several reports exist of goiter and hypothyroidism in foals associated with ingestion of excessive amounts of iodine in kelp-supplemented rations.4,105,115–117
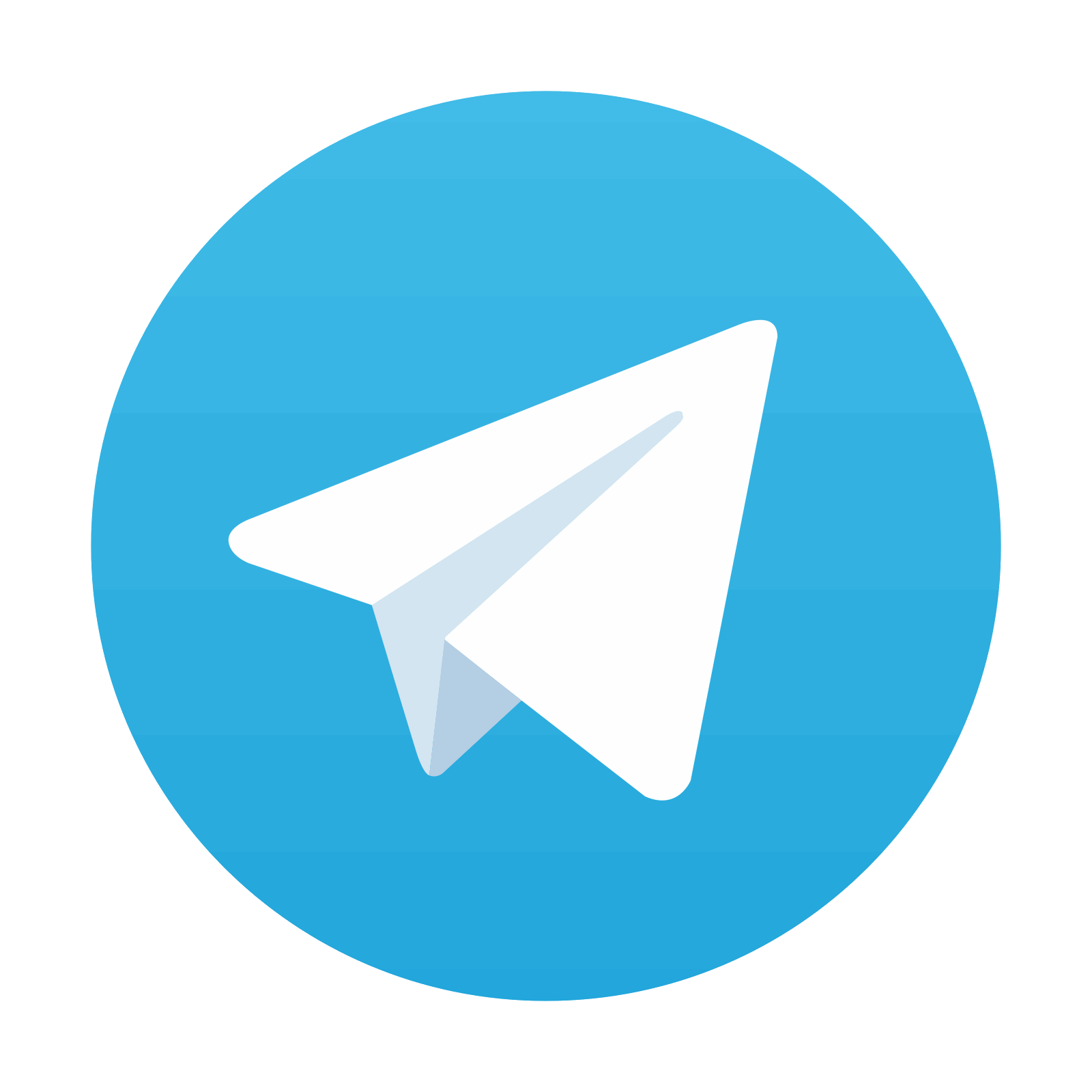
Stay updated, free articles. Join our Telegram channel

Full access? Get Clinical Tree
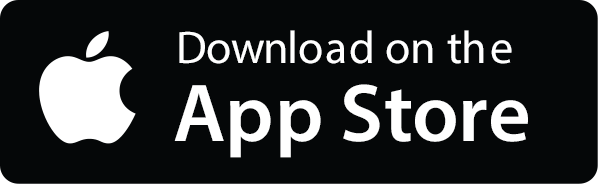
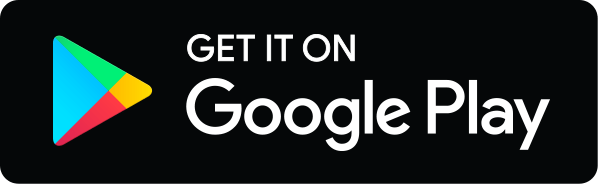