Disorders of the Digestive System
Anatomy and Physiology
Dentition
Infant New World camelids may have up to 22 deciduous teeth, of which 16 generally erupt and become functional.1,2 The deciduous dental pattern per side is as follows:
The single upper incisor is caudal to the last lower incisor. It is crescent shaped and pointed, similar to the canines. Adult canines and upper incisors together make up the fighting teeth (Figure 40-1). They are larger in males than in females and also poorly developed or do not erupt in camelids castrated before sexual maturity. Development of the fighting teeth is the only gender-based differences in dental pattern in camelids.
Eruption times for the lower incisors, from front to back, are 2 to 2.5 years, 3 to 3.25 years, and 3 to 6 years.1 Canines usually erupt at between 2 and 4 years of age but may come in years later and grow more slowly in females or geldings. Premolars usually erupt between 3.5 and 5 years of age, and the molars, from back to front, erupt at 6 to 9 months, 1.5 to 2 years, and 2.75 to 3.75 years of age. The significance of this is that younger adults will frequently have their permanent cheek teeth but still have one or more deciduous incisors (Figure 40-2).
Figure 40-2 Retained deciduous incisors in a camelid. The middle incisors depict malocclusion as well.
Llama and guanaco incisors are covered in enamel and have a broad, flat cutting surface that works against the upper dental pad.3 Alpaca and vicuña incisors have a narrower cutting surface with enamel only on the labial surface. The incisors of llamas and guanacos are tapered toward the root, whereas the incisors of vicuñas and alpacas are rectangular, have an open root, and erupt continuously. Hybridization has confused this distinction somewhat.
Adult premolars are less than one quarter the size of molars and occasionally are absent or do not erupt. Mandibular cheek teeth are narrower compared with maxillary teeth, and mandibular arcades are closer together. Although camelids chew with a rotary motion, they tend to have very uneven wear on the occlusal surface of their cheek teeth (Figure 40-3). Points or ridges may develop on the lateral maxillary arcades and the medial mandibular arcade and may require filing. In spite of the unevenness, evidence that these points cause trauma or chewing disorders is rare.
Salivary Glands and Saliva
Two large salivary glands are located along the caudal border of the vertical ramus of each hemimandible: (1) the parotid glands are the largest and most superficial and have a serous secretion, and (2) the ventromedially located mandibular glands have a mixed mucous–serous secretion.4,5 Numerous mucus-secreting glands are located throughout the oral cavity, including the buccal, palatine, sublingual, and labial regions. Most of these are diffuse aggregates of secretory cells, not distinct tissue masses. Some of these glands, namely, the sublingual glands, also secrete small amounts of serous fluid, but camelids appear to lack a large serous salivary gland in the mouth comparable with the inferior molar gland of sheep.
Several investigations into the content of both parotid and mixed salivary secretions of camelids have been conducted.5–8 Normal camelid saliva is alkaline (pH = 8.0 to 8.6) with high bicarbonate (100 to 150 milliequivalents per liter [mEq/L]), phosphorus (18 to 34 mEq/L), sodium (148 to 175 mEq/L), and potassium (6.5 to 14.0 mEq/L) concentrations. Salivary chloride concentration is very low (20 to 30 mEq/L). A slight increase in bicarbonate concentration occurs during feeding. Total fasting salivary output is approximately 6 milliliters per kilogram per hour [mL/kg/hr], with each parotid salivary gland secreting about 2 mL/kg/hr.6,7 Both parotid and total salivary outputs increase by over 50% during eating and cud chewing. On the basis of this information, camelid saliva appears to have similar biochemical features to ruminant saliva, and probably plays an important role in buffering gastric acid. The rate of saliva production in camelids is also similar to that in roughage-fed ruminants. However, the parotid glands of camelids are proportionally similar in mass to those of ruminants adapted to poorly fermentable diets and approximately one third the size of the glands of ruminants adapted to rich diets.9 This suggests that camelids have limited ability to increase salivary buffering of fermentation in response to higher rates of forestomach acid production.
Cud-Chewing Behavior
Similar to true ruminants, camelids use cud chewing to break down large feed particles. Initial mastication by camelids is cursory and consists of only enough chewing to mix the food with saliva to form a bolus for swallowing. To further decrease particle size and increase surface area for microbial digestion, cud is propelled from the first gastric compartment back up the esophagus into the mouth, where it is rechewed and mixed with saliva. This process has been studied extensively in the vicuña and probably is similar in other camelids.10 After regurgitation of a cud, the mandible makes a “figure-of-8” arc during chewing. In approximately 15 seconds, 25 to 30 chews occur, after which the cud is swallowed. The cycle may repeat itself many times. In contrast to ruminants, which tend to retain a cud on one side for several chews, camelids appear to move the cud from side-to-side with each chew.9 Saliva is expelled primarily from the ipsilateral parotid duct each time, potentially minimizing salivary loss and maximizing the contribution of both glands to the digestive process.
The actual grinding is a concerted lateral-to-medial motion of the working side of the mandible.11 Adults with full molar occlusion have longer chewing cycles compared with juveniles both before and after the first molar comes into wear.12
Esophagus
The esophagus lies deeper in the camelid neck than in other species and is somewhat obscured by thick skin between the trachea and the left transverse processes of the cervical vertebrae. The mucosa and submucosa are similar to those of ruminants, with an abundance of submucosal mucus glands underlying the stratified squamous mucosa. The tunica muscularis consists predominantly of skeletal muscle distributed in an inner longitudinal and an outer circular muscle layer (Figure 40-4). This is opposite to the pattern of fiber orientation in ruminants. The well-developed skeletal musculature of the camelid esophagus is necessary to move a cud up the long, vertical neck. The esophagus receives its sensory and motor innervation from the vagus nerve and its branches.
Gastric Anatomy and Physiology
Adult camelids, similar to adult cattle and other ruminants, rely on bacterial fermentative digestion in the forestomach to extract nutrients from plant material. This requires a warm, moist anaerobic environment with a large capacity and long fiber retention times and the ability to mechanically and enzymatically break down large feed particles. Mechanical breakdown is achieved through cud chewing and gastric contractions. Forestomach enzymes are primarily microbial in origin. Bacterial fermentation of plant carbohydrate results in the production of organic volatile fatty acids (VFAs). Under normal fermentative conditions, acetate, propionate, and butyrate are the major VFAs produced, in a ratio of approximately 4 : 1 : 1 before feeding and approximately 7 : 2 : 1 a few hours after feeding.13 Absolute VFA concentration after feeding in both New World camelids and Old World camelids is approximately 140 millimoles per liter (mmol/L), which is 30% to 40% higher than in ruminants under similar conditions.13,14 The production and presence of these VFAs and other organic acids acidify the fluid within the forestomach compartments. Although gastric (forestomach) fluid pH varies with diet and fermentative activity, camelids on a diet consisting predominantly of forage should have a forestomach fluid pH between 6.4 and 6.8; that is, the VFAs exist predominantly in their ionic forms. Carbon dioxide and methane are additional products of fermentation. Further discussion of fermentative function may be found in Chapter 8.
In camelids, fermentative digestion occurs in the first two gastric compartments (C1 and C2) and the cranial four fifths of the third gastric compartment (C3). The first gastric compartment occupies most of the left side of the abdomen. It consists of large cranial and caudal sacs, which are divided ventrally by a prominent transverse pillar and together contain about 83% of gastric volume and about 50% of abdominal volume (Figure 40-5).15 The delineation between the sacs is easily visible or palpable from either outside of or within the compartment. The transverse pillar forks medially into cranial and caudal portions near the cardia. The small, reniform second compartment (6% of gastric volume) rests atop C3 medial to C1, with its lesser curvature oriented toward C1 and its greater curvature protruding toward the right dorsolateral body wall. The first two compartments and their contents account for approximately 10% of the body weight of an adult alpaca and 15% of an adult llama.7,16 The canal between C2 and C3 is very narrow and probably represents an important size regulator for particle transport to the lower GI tract. The third compartment (11% of gastric volume and 1.5% to 2.5% of adult body weight) is long and tubiform, with both ends upturned.7,16 It extends cranioventrally and then caudally from its attachment to C2, occupying the right cranioventral abdomen. Near the umbilicus, its terminal portion curves craniodorsad and may extend 10 to 30 cm forward atop the tubiform portion.
Figure 40-5 Comparison of the sizes of the large cranial (top left) and caudal (bottom left) sacs of the first gastric compartment, and the smaller, narrower third compartment (bottom right). The small second compartment makes up the rounded canal toward the top right. The two large, dark areas on the surface of the first compartment are the saccular regions.
First and Second Compartments
Stratified squamous epithelium lines most of the superficial mucosal surface of C1 and the lesser curvature and primary septa of C2.15 The squamous region of C2 may contract together with a muscular band located near the cardia in C1 to form the esophageal groove for transport of milk directly into C3. The squamous epithelium of the forestomach is keratinized in camels, but not in New World camelids.17,18 No structures are analogous to the frondular papillae that ruminants have extending into the forestomach lumen (Figure 40-6). Instead, camelids have two types of specialized structures that evaginate from the forestomach lumen: (1) the glandular saccules of C1 and (2) the glandular cells of C2. The saccules of C1 are found in two groups, one on each side of the transverse pillar. Each group is arranged in rows. The smaller group is found at the cranioventral aspect of the cranial sac, and the larger group is found immediately caudal to the transverse pillar on the ventral surface of the caudal sac. The rows are divided by muscular primary septa, which are much more prominent in the caudal sac, where they are contiguous with and perpendicular to the transverse pillar. The saccules in each row are separated by smaller secondary septa. In the middle rows of each group, two saccules usually are present side-by-side between the primary septa, divided by a small tertiary septum and occasionally a quarternary septum. Each saccule has a very small orifice (about 5 millimeters [mm] in an adult llama) controlled by a muscular diaphragm protecting a wider saccular lumen (about 10 mm). The saccules of the caudal sac alone account for half the surface area of the first compartment.7 The saccules are visible as bumps along the serosal surfaces of the gastric compartments or as evaginations when viewed from the mucosal side.
Figure 40-6 The internal surfaces of the first (left) and second (right) compartments. Note the lack of papillae and the presence of muscular septae dividing the glandular saccules or cells, and the wider opening to the second compartment cells.
The glandular cells of C2 are larger with wider openings (see Figure 40-6).15 Primary septa originate along the squamous region and extend to the greater curvature. Secondary and tertiary septa branch off to subdivide the region into cells but do not form muscular sphincters. The mucosa within these cells can have small papillae, but these are not always present. The serosal surface of C2 is uniform, and the cells are not apparent as individual structures. The C2 cells are weakly muscular and do not evert.
The nonsaccular mucosa and septa are covered with stratified squamous epithelium, and the mucosa within the C1 saccules and C2 cells consists of folds of simple columnar epithelial cells.18 These cells have microvilli along their luminal membrane and contain numerous mitochondria. They also contain both mucoid and nonmucoid secretory granules. Cellular morphology suggests both absorptive and secretory functions. Saccular cells may be subdivided into two populations: (1) simple columnar epithelial cells, which resemble those of the small intestinal mucosa and have primarily absorptive function, and (2) a second group that resemble tubular gland cells.7 It is postulated that this second population of cells, especially those nearest to the compartment lumen, secrete a mucus coat that protects the saccular membrane against mechanical trauma, acid injury, and microbial invasion. Evidence of this mucus coat may be found in live camelids.17
In spite of their designation as “glandular” saccules, little evidence exists for substantive secretion beyond this thin mucus coat. Water, electrolytes, and VFAs are absorbed very quickly across the saccular membrane.7,19 Absorption of these molecules across this membrane is two to three times faster than across the rumen membrane in sheep and goats and results in a generally drier gastric contents than is commonly found in cattle or sheep. Unlike ruminants, camelids appear to absorb water across the forestomach membrane in direct relation to the amounts of sodium and VFAs absorbed. In contrast to sodium and water, camelids appear to absorb less chloride. The submucosa of each saccule is filled with a complex network of capillaries, which supports the importance of the saccules in nutrient absorption.
Both camelid saccules and ruminant papillae increase the surface area for the absorption of nutrients but function very differently. The camelid saccules contain small quantities of gastric fluid in small pockets surrounded by highly absorptive epithelium and constantly renew this fluid through saccular eversion and refilling. The high rate of contractions, the high surface area-to-volume ratio, and long particle retention times of the camelid forestomach may be responsible for the digestive advantage that camelids have over ruminants with regard to poor-quality forage.14,20,21 The papillae of ruminants also help preserve the ruminal environment through absorption and removal of organic acids from the lumen. This is especially important when VFAs are produced in large quantities, as occurs when a highly fermentable feed is provided. The size of the papillae and surface area increase, over time and with limitations, when VFA production is high, allowing faster removal of VFAs. Thus, the ruminant can adapt itself to higher energy feeds by increasing the rate of acid removal. We do not know if the saccules of camelids are capable of similar adaptation, and our belief is that they are not. Thus, camelids may be less adapted to handle high energy feeds compared with their ruminant counterparts. This is contrary to the popular belief that camelids are resistant to this type of feed-related disease.
How the saccular epithelium facilitates absorption is unknown. Saccular fluid has a higher bicarbonate concentration than forestomach fluid, and this bicarbonate concentration increases with time if the saccules are exposed to chloride-rich or VFA-rich solutions.7,8 This has led to one theory that bicarbonate is secreted across the saccular membrane into the forestomach in exchange for chloride to buffer the acids produced by fermentation. A similar function has been described for the cells of the gastric cardia of monogastrics, which secrete bicarbonate in exchange for chloride from hydrochloric acid to protect that region from acid damage. The problems with extrapolating this function to camelids are twofold: (1) The gastric saccules are rarely exposed to high chloride concentrations because the chloride concentration in the forestomach fluid of camelids is normally very low (less than 20 mEq/L); and (2) it would be unusual to assume that an animal adapted to a low nutrient environment would have developed a mechanism to protect itself against overingestion of rapidly fermentable nutrients.
According to another theory, the high bicarbonate concentration in the saccule could be an example of ion trapping, rather than active secretion by the columnar epithelium of the saccules.7,22 By this, it is understood that some of the carbon dioxide and water, both of which are found in abundance within the forestomach fluid, combine to form carbonic acid, which also may exist as separate bicarbonate and hydrogen ions. VFA, although a weak acid, usually exists in its ionized form at typical C1 pH. The ionized form crosses the lipid cellular membrane with difficulty, but if it momentarily captures the hydrogen molecule from carbonic acid, it is removed from the system by absorption across the saccular membrane. This leaves the bicarbonate molecule within the saccular fluid, which explains the high bicarbonate concentration at the site of VFA absorption and perceived secretion into the saccular fluid and would be compatible with our understanding of normal conditions in the camelid stomach.
More recent work suggests a third theory. Some evidence suggests that VFAs are transported across the camelid forestomach wall primarily in their ionized form.19 This may occur through VFA–bicarbonate ion exchange. Although this could potentially promote gastric buffering during times of high VFA production, it would not suitably buffer lactate, half of which is poorly absorbed.
Neonatal camelids start with a nonfermentative stomach. In spite of that, their fermentative stomach compartments are relatively large compared to their analogs in neonatal ruminants. The full first compartment is about 45% of total gastric weight at birth, and reaches 60% by about 6 weeks of age.23 The second compartment starts at around 10% of gastric weight and declines slightly into adulthood. A functional microbial population becomes established between the first and second weeks of life, and by 12 weeks, fermentative function is at adult levels.
Forestomach Motility
Two described contraction cycles occur in the forestomach of camelids. The A-cycle begins with a strong contraction of the C2-C3 canal, followed by a rapid contraction of C2 and relaxation of the canal.24–26 Following this, both caudal C1 and the canal contract up to eight times. In guanacos, the C1 contractions move from caudoventral to caudodorsal, followed by a cranial sac contraction.27 In alpacas, the whole caudal sac appears to contract together.26 A final weak contraction of the canal ends the cycle. The entire sequence takes about 1 to 2 minutes. Clinical interpretation of multiple contraction cycles during that time results from interpretation of the multiple C1 contractions of a single A-cycle as multiple cycles. The A-cycle is responsible for fluid passage into C3 (during the first C2 contraction only), expressing fluid from the fiber mass, and mixing of the contents of C1. Experimental data from one llama revealed that fluid passage into C3 occurs at a rate of approximately 7 mL/kg/hr, with about 0.14 mL/kg passing through the canal with each A-cycle.28 The order of contraction of the various parts of caudal C1 is still being debated, but the overall effect is slow counter-clockwise rotation of the ingesta, when viewed from the left.
One or more B-cycles follow each A-cycle.25 The B-cycle starts with contraction of the cranial compartment of C1 during the last previous canal contraction, followed by a weak contraction of C2, and finally by contraction of the caudal compartment of C1. The B-cycle lasts about 9 seconds and may be repeated several times before the next A-cycle. The B-cycle primarily aids in mixing of digesta, expressing fluid from the fiber mass, and renewing the saccular contents but generally does not stimulate passage of fluid into C3. Rumination and eructation of gas each occur during some B-cycles. If the camelid inspires with a closed glottic prior to the contraction of cranial C1, ingesta is drawn into the esophagus by negative intrathoracic pressure, and propelled to the mouth by an antiperistaltic wave of contraction of the esophageal musculature.29 Eructation is accomplished by an additional contracture of caudodorsal C1 at the end of the B-cycle. Up to approximately 100 A- and B-cycles may occur in an hour during eating and rumination, but both cycles may be absent for 10 to 20 minutes at a time when the camelid is not ruminating.
Both A- and B-cycles depend on vagal stimulation, and are blocked by atropine, acetylcholine, or epinephrine.25 The frequency of A-cycles is decreased and B-cycles increased by mechanical distension of cranial C1 (A-cycles only), the C2-C3 canal, and cranial portions of C3. Mechanical distension of distal C3 appears to inhibit A-cycles and stimulate B-cycles, although its effects on A-cycles are inconsistent. The clinical relevance of these effects is that forestomach distension stimulates gastric mixing and rumination but not necessarily gastric outflow, whereas distension of the terminal fermentative stomach (distal C3) alone promotes aborad flow of ingesta. Clinical experiences support that pathologic distension of the gastric compartments inhibits motility as in ruminants, although this effect has not been verified experimentally.
The Third Compartment
The cranial portion of C3 is covered with glandular epithelium similar to that which lines the forestomach saccules.30 Most of this is organized in longitudinal folds, although a small wedge of reticular-type folds is present near the junction with C2. The caudal 20% of the compartment, as measured along the greater curvature, is lined with gastric glands, which secrete hydrochloric acid and acid proteases. The mucosa in this region is much thicker than the mucinous glandular region, 7 to 10 mm compared with the 3 to 4 mm of the fermentative region.31 Because the diameter of the lumen decreases caudally and the lesser curvature bends almost 180 degrees on itself toward the fundus, the gastric glandular portion represents only a small percentage (about 20%) of the surface area of C3. Luminal pH drops abruptly from forestomach levels in the fermentative portion to four (or less) in the gastric portion.9,32 Some researchers consider this area the “true stomach,” a separate entity from C3, although most consider that C3 has both fermentative and acid-secreting regions. The rugal folds become much deeper near the pylorus, which is a fibrous band, and the epithelium in this region is dominated by mucus and bicarbonate-secreting pyloric glands.
Water, sodium, and VFAs are absorbed rapidly across the C3 membrane.28 Approximately 70% of the VFAs, 60% of the sodium, and 30% of the water that enter from C2 are absorbed in C3. Extensive water and chloride are secreted into the terminal gastric portion, but this has not been quantified.
Third Compartment Motility
Circumferential contractions occur along the length of C3. These occur simultaneously and are weak and common (10 per minute) in the cranial portion but have peristaltic organization and are stronger and slower (5 per minute) in the caudal regions.27 Distension stimulates local progressive motility while inhibiting activity both cranially and caudally.25 Distension of the cranial region inhibits the passage of fluid from the forestomach into C3, whereas caudal C3 distension promotes it. Motility of the fermentative region is unaffected by C1 and C2 distension but is inhibited by canal distension and vagolytic drugs. Motility of the fundic region of C3 is affected only by local stimuli, not vagal input. Nitric acid appears to play an inhibitory role.32 Under normal conditions, once fluid and ingesta have reached the fundic portion of C3, they should not reflux into the fermentative portion.27
Small Intestine
The first 10 cm of the duodenum in adult llamas are similar in diameter to terminal C3 (Figure 40-7). This ampulla wraps around the pylorus from the lesser curvature to the greater curvature, turning caudad at the ventral border of the liver. After this, the duodenal diameter decreases abruptly. The most prominent feature of the subsequent duodenum is the M-shaped flexure of its cranial loop, which is intimately attached to the greater curvature of C3 by the greater omentum. This tortuous passage and the approximately 10-fold reduction in diameter from C3 to the small intestine make this a common site for feed or foreign body impaction.
Figure 40-7 The pyloric region of the third compartment and the duodenal ampulla, leading into the narrow cranial duodenum. This area is a common site for bezoar obstruction, especially in alpacas.
After coursing craniad to receive the hepatic ducts, the duodenum courses caudad and wraps around the root of the mesentery (right to left around the back), becoming intertwined with the transverse colon at that point. The intertwining of these two structures suggests that camelids undergo the same clockwise rotation of intestinal structures around the mesenteric root during development as has been described in other mammals. The jejunum begins a short distance cranial to the root of the mesentery, and its abundant loops occupy most of the right dorsal abdomen. The proximal two thirds of the jejunum consist of tight coils on a short mesenteric attachment (5–7 cm). These loops are difficult to exteriorize through an incision. The distal one third consists of a longer (30–35 cm) mesenteric flange, which imparts more mobility. Small rents are occasionally found in this mesenteric flange. The ileum is short and enters the cecum near the midpoint of the abdomen. The small intestine usually is flaccid and contains only a small amount of fluid and semisolid feed boluses. Very little is known about the physiology of the camelid small intestine. It is presumed that fluid and electrolyte cycling, digestion, and nutrient absorption occur much as in other mammals. Sugar digestion has been studied in dromedaries, and it has been found that glucose, galactose, and lactose are readily absorbed, whereas maltose and sucrose are not.33
Except for the duodenal ampulla, the maximal diameter of the small intestine is around 2 cm in an adult llama and 1 cm in an adult alpaca or juvenile llama.31 However, distension to this degree should occur only with the intermittent passage of a food bolus. The cranial mesenteric artery courses through the mesentery, sending short, arcuate branches to the jejunum. These branches become progressively longer into the flange until a single long branch courses along the mesenteric border of the ileum.
Cecum, Colon, and Rectum
The camelid cecum is fairly small (about 7 to 10 cm long in adult alpacas and 15 to 20 cm in adult llamas), and usually contains a few handfuls of doughy material. The ileocecal valve is small and tight. The base is usually centrally located in the abdomen, where it is held in place by ligamentous attachments to both the ileum and the colon and a short mesenteric root. Almost the entire length of the cecum is attached by a ligamentous band to the antimesenteric border of the ileum. The beginning of the ascending colon is usually as large as the cecum, and often more prominent. The ascending colon may be followed into its spiral, which usually centers over the ventral abdomen caudal to the gastric compartments, or extends up to the right lateral abdomen. The mesentery of the cecum and ascending colon is an extension of the distal mesenteric flange (Figure 40-8). Hence, it is long and relatively narrow and contains the arterial blood supply to the region.
Figure 40-8 The pendulous nature of the long, narrow mesenteric attachment to the ascending colon. This section of bowel is most prone to become entrapped or rotate on its root.
The colon aborad to the spiral loop passes very closely to the ileocecocolic junction, to which it is attached by the cecocolic ligament. The transverse colon then reverses direction 4 more times as it courses dorsad, forming another small spiral, and wraps around the mesenteric root (left to right around the front) to intertwine with the duodenum. The descending colon courses caudad into the rectum. These sections of the colon and rectum are held on a very short mesenteric attachment. The cranial portion of the colon is usually filled with doughy feed material which is gradually dehydrated to form fecal pellets. Fecal pellets may be found as far forward as the centrifugal loops of the spiral colon but are more normally found only in the transverse and descending colon and rectum.
Diagnostic Approaches
Physical Examination and Diagnostic Tests
Rectal Examination
Though juvenile llamas and most alpacas may be too small, rectal examination of most adult female llamas is possible with good restraint (preferably in a camelid chute), adequate lubrication and, if necessary, chemical sedation. Adding 20 mL of a local anesthetic agent (2% lidocaine hydrochloride [HCl]) to 100 to 120 mL of lubricant and injecting the mixture into the rectum by plastic dose syringe or insemination pipette may relax the anal sphincters and caudal rectum sufficiently to allow a good rectal examination. However, clinicians with large hands may choose not to perform the examination to avoid the risk of causing injury to the patient.34
Rectal examination of the llama is similar to that of the cow; the bladder, reproductive tract, left kidney, and caudal first gastric compartment can usually be palpated. The right abdomen usually has no palpable viscera. The bladder may be distended with urinary tract obstruction or simply because of lack of urination in the stressed camelid, and portions of the small and large intestine may be distended with intestinal obstruction. Care must be taken, as rectal injuries and bladder rupture have been reported.34 For the most part, abdominal ultrasonography has supplanted rectal palpation.
Abdominal Paracentesis
Abdominal fluid is best obtained from a paracostal site on the right flank (Figure 40-9).35 This site is caudal to the last rib and approximately one half the distance from the ventral to dorsal midline. Alternatively, the operator may choose a site two (alpacas and crias) or three (adult llamas) finger-widths caudal to the caudal-most aspect of the ribs. The patient should be restrained and possibly sedated to restrict movement. The fleece should be clipped and the site aseptically prepared. Two milliliters of lidocaine HCl are infused subcutaneously and intramuscularly, and a stab incision made into the muscle with a #15 scalpel blade. A sterile teat cannula is introduced perpendicular to the skin through the incision into the abdomen, and fluid s collected by gravity flow into a Na-EDTA (sodium–ethylenediaminetetraacetic acid) tube for cytologic analysis and a clot tube for bacteriologic culture (Figure 40-10). Heparinized fluid may be collected for immediate biochemical analysis. This technique is successful in about 95% of camelids, with unintentional gastrocentesis rarely occurring because of the sideways orientation of the cannula. The procedure may be done with the patient standing in the kush position or in left lateral recumbency.
Figure 40-9 The landmarks for right paralumbar abdominocentesis. The black lines represent the caudal margin of the last rib (curve) and the linea semilunaris or aponeurosis of the external abdominal oblique muscle (straight). The red dot represents the approximate site for centesis.
Normal camelid peritoneal fluid is colorless to slightly yellow and clear, and contains up to 2.5 milligrams per deciliter (mg/dL) of protein and 3000 nucleated cells per microliter (µL).35 A few camelids have higher cell counts and protein concentrations, usually not simultaneously and associated with very low fluid volumes. Camelids have a higher percentage of neutrophils (from 15% up to 98%) among the nucleated cells in abdominal fluid compared with most other domestic herbivores. Most of the remainder are large mononuclear cells. Because of the wide variations in normal, drawing inferences from cell percentages is more difficult than from absolute counts. Typically, we see up to about 2000 neutrophils per microliter and 1000 mononuclear cells per microliter in healthy camelids.
Forestomach Fluid Analysis
Although the diagnostic value of forestomach fluid analysis is well established in ruminant medicine, its use is rarely reported in scientific papers about camelids.36 Fluid may be retrieved from the forestomach by passing an orogastric tube into the stomach, applying suction to the tube, and crimping the tube to retrieve the sample held within its distal end.37 Unless abnormal fluid accumulation is present within the forestomach (GI reflux, forestomach acidosis, recent oral fluid administration), it is rare that fluid is siphoned all the way to the proximal end of the tube. Adequate physical restraint is necessary, and chemical sedation may facilitate the procedure. A length of a semirigid hose, a roll of tape, a wooden block with a hole drilled through it, or a polypropylene tube may be used as a mouth speculum (see Figure 30-1). Regurgitation may occur around the tube, especially when abnormal fluid accumulation is present in the forestomach. In the case of regurgitation, the tube should be pulled immediately and the camelid’s head depressed to prevent aspiration.
A percutaneous technique has also been described in llamas.38 With this technique, a 16-gauge, 7.5-cm needle is advanced dorsocraniomedially from a point approximately 20 cm caudal to the costochondral junction of the last rib on the left side. Once the first gastric compartment is punctured, fluid is aspirated and the needle withdrawn rapidly. This technique avoids salivary contamination but has not been used much in sick camelids, which potentially would have more complications from peritonitis compared with their healthy counterparts.
Determination of the chloride concentration of forestomach fluid has proven to be a reliable test for GI obstruction. However, care must be taken to ensure an appropriate method of analysis. Other anions including VFAs affect the chloride electrodes of many analyzers.39 Coulometric titration appears to be the most accurate method. Samples taken from healthy as well as sick and nonobstructed camelids almost always has a concentration less than 30 mEq/L.
Blood Interpretation
1. Fresh whole blood for total and differential white blood cell (WBC) counts, total protein and fibrinogen concentrations, red blood cell (RBC) numbers and morphology
2. Serum or plasma for sodium, potassium, and chloride, total protein, albumin and globulin fractions, bicarbonate–total carbon dioxide (HCO3/TCO2) reflective of gut function and health, and blood urea nitrogen (BUN) and creatinine, liver enzymes and lactate, which are reflective of more global health issues
Radiography
Radiographs are commonly used to evaluate the skull and esophagus, and less frequently, other parts of the gastrointestinal system.40–44,46 In the skull, jaw masses and dental abnormalities are the most common indications. To localize lesions, lateral, dorsoventral, and oblique views are useful. Use of a radiolucent speculum aids in the separation of the dental arcades. Patients must be heavily sedated to allow proper alignment, especially for the dorsoventral and oblique views. Intraoral radiography may be useful for demonstrating cranial jaw lesions.
Cervical and thoracic radiography is often used to assess esophageal function.43 Normally, the esophagus is scarcely recognizable on plain films. Liquid contrast material fed by dose syringe or mixed with grain or feed pellets highlights the mucosa and allows assessment of lumen size and emptying function. We commonly use up to 120 mL of barium sulfate (60% weight/volume) in adults and 20 to 50 mL in neonates. Healthy camelids usually clear the barium within a few seconds, so retention of all but a superficial layer after 30 seconds is considered abnormal.
Contrast radiography has been especially helpful with esophageal disorders. Approximately 100 mL of contrast material given to an adult llama is usually sufficient. Contrast may also be used to highlight the first gastric compartment to help distinguish it from other structures in cases of colic in crias. Intestinal passage of contrast material in crias has been described, but in sick crias, contrast tends to remain in the stomach.45 Contrast normally enters the proximal loop of the ascending colon after 2 to 4 hours but may not enter the spiral colon for over 16 hours.
Advanced radiologic imaging techniques such as computed tomography (CT) have seen limited application in camelids but will doubtless see more application in the future.47,48 Current reports on CT use are mainly descriptive studies of normal camelids, or examinations of the head. CT may also be used to identify vascular ring anomalies in the chest or thickened bowel or mass lesions in the abdomen.
Ultrasonography
We have performed abdominal ultrasonography on a number of camelids with GI disease, but more work is needed to establish parameters of normal and abnormal findings.49 We have used both linear array and sector scanners with 3.5 to 7.5 megahertz (MHz) probes. The 5-MHz equipment commonly used for reproductive evaluation of camelids is usually adequate.
Examination may be performed with the animal in the standing position or in lateral recumbency.31 In the cranioventral abdomen, the first and third gastric compartments (Figure 40-11) appear as large, thick-walled, fluid-filled viscera on the left and right sides of midline, respectively. The space between them, usually 3 to 7 cm right of midline, contains hypoechoic peritoneal fluid. The first compartment extends the length of the abdomen, with the saccules clearly visible as semicircular, thin-walled hyperechoic protrusions from the compartment wall caudal to the transverse pillar and cranial to the umbilicus. The compartment and saccular lumens often contain numerous hyperechoic densities with shadows caused by bezoars, feed material, and gas. These prevent clear imaging of the lumen. The third compartment can be followed from the xiphoid past the umbilicus, where it curves dorsad and craniad. It can be recognized by its curved walls in cross-section. Third compartment ingesta is usually less echogenic and more uniform in appearance than the first compartment fluid and enables good imaging of the wall. It is important to remember that the wall normally thickens from 4 mm in the cranial regions to 7 to 10 mm in the true gastric region.
Exploratory or Laparoscopic Surgery
Exploratory surgery is a useful test in that it allows direct or camera-mediated inspection of various organs, correction of a variety of maladies, or collection of biopsy samples. For most purposes, ventral midline or right paralumbar fossa approaches are satisfactory. Obviously, if the surgeon desires to enter the first compartment, the left flank is preferable to the right, and if terminal C3 is of special interest, a right paracostal approach allows the best inspection of a variety of poorly mobile regions. These are discussed in detail in Chapter 57.
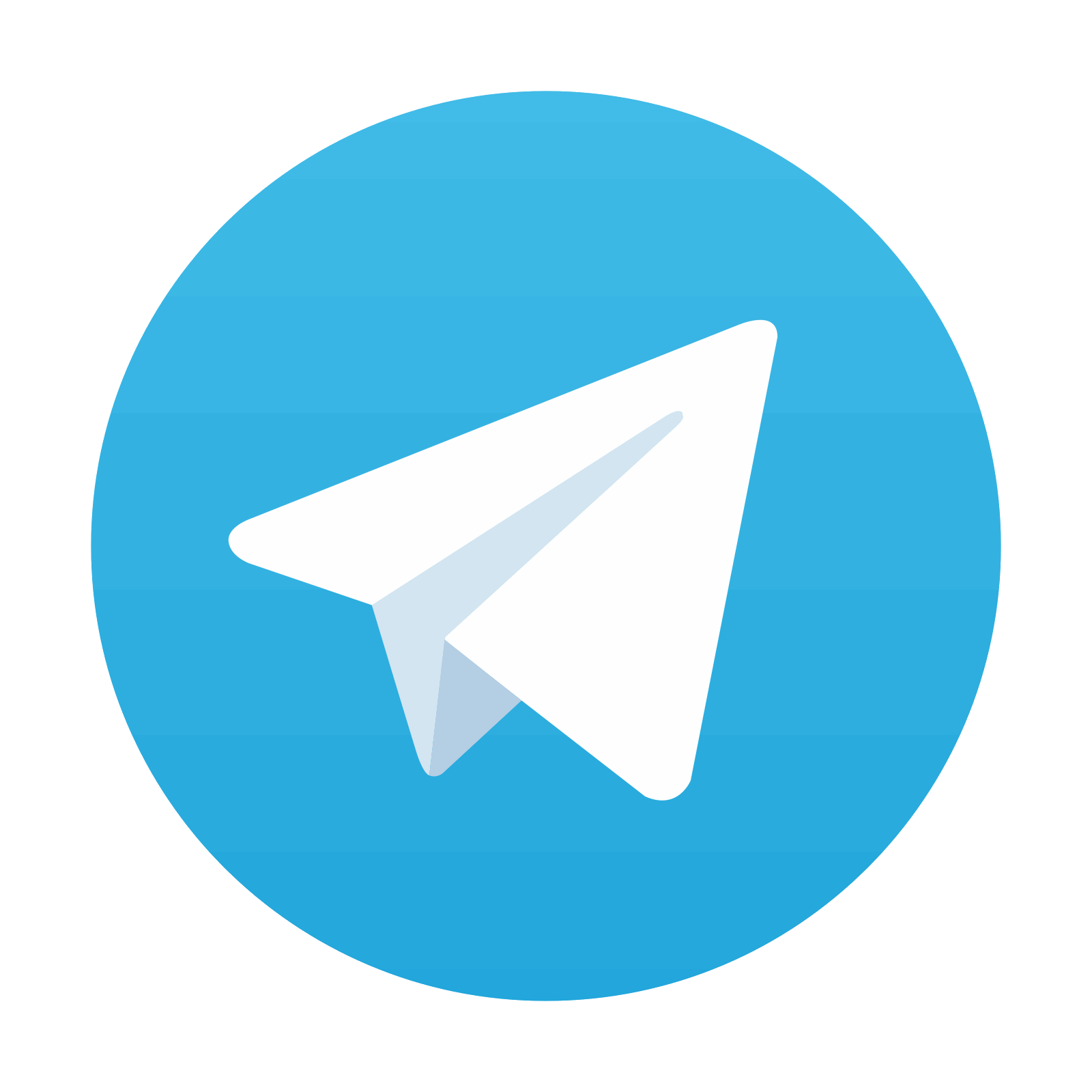
Stay updated, free articles. Join our Telegram channel

Full access? Get Clinical Tree
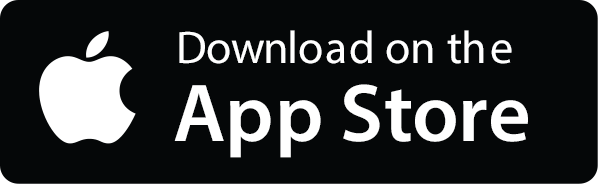
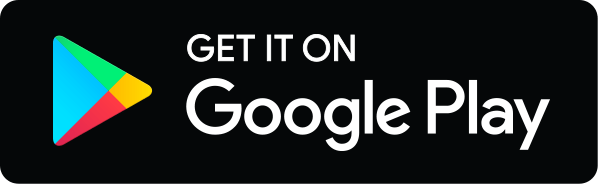