Revised from 6th edition of Veterinary Ophthalmology, Chapter 24: Diseases and Surgery of the Canine Vitreous, by Michael H. Boevé and Frans C. Stades; Chapter 25: Diseases of the Canine Ocular Fundus, by Simon M. Petersen‐Jones and Freya Mowat; Chapter 26: Surgery of the Canine Posterior Segment, by Allison R. Hoffman, Samuel J. Vainisi, Joseph C. Wolfer, and András M. Komáromy; and Chapter 27: Diseases of the Canine Optic Nerve, by Gillian J. McLellan The vitreous, also called the vitreous body or corpus vitreum, is a transparent, elastic hydrogel accounting for approximately 80% of the volume of the canine globe. Posteriorly and about it sides, the vitreous is bordered by the retina, for which it provides support, and the optic disc, and at the anterior side by the posterior lens capsule and the Zinn zonules (i.e., fossa patellaris). By days 16–24 of gestation, the lens vesicle becomes separated from the surface ectoderm from which it originated, and the ectodermal and mesodermal fibrillar material extends into the space between the lens vesicle and presumptive retina, thus forming the primitive vitreous. Later, the primitive vitreous is concentrated centrally, in the optic cup, while the definitive or secondary vitreous develops around it. After formation of the lens vesicle, the hyaloid artery, which is of mesodermal origin, penetrates into the primary (or primitive) vitreous through the optic fissure and grows toward the posterior pole of the lens. Just retrolentally, the hyaloid artery branches, generally into three main trunks, which subsequently develop into a vascular network around the primitive lens, thus forming the tunica vasculosa lentis. More proximally in the vitreous space, the hyaloid artery branches into the vasa hyaloidea propria. On the anterior side of the lens, the primitive vascular system anastomoses with the annular vessel at the rim of the optic cup, which is the location where the iridal base will later be formed. In the dog, the hyaloid system is at its maximal development by day 45. At this stage, the hyaloid system has developed into an elaborate vascular network. After approximately day 45, however, the hyaloid system starts to regress, and between the second and the fourth week after birth, the entire vitreal vascular system has degenerated. Eventually, only a small, short, usually corkscrew‐shaped, rudimentary string of the hyaloid artery remains, which is attached retrolentally to the posterior lens capsule just ventral to the posterior pole between the two ventral suture lines; the site of attachment to the posterior lens capsule is named Mittendorf’s dot. Remainders of the primitive vitreous concentrate around the hyaloid artery. After regression of the latter, a channel‐like structure (i.e., Cloquet’s channel) remains, but this structure is only barely observable in normal adult canine eyes. The vitreous forms one of the refractive media of the eye, and it provides the necessary pressure to maintain the neuroretina properly positioned against the pigment epithelium. The two main solid components of the vitreous are hyaluronic acid and collagen. Approximately 1% of the vitreous consists of a network of polygonal, hydrated fibrils of hyaluronic acid and collagen (type II, with a helical structure of α1(II)3). As cellular component, few hyalocytes, which originated from blood macrophages, are mainly located peripherally, near the ciliary body. The hyalocytes are believed to be responsible for the production of glycosaminoglycans, especially hyaluronic acid. The remaining 98–99% of the vitreous consists of water. The outer limits of the vitreous do not consist of membranes; rather, they consist of condensations of fibrils that are firmly attached to the ora ciliaris retinae and the pars plana ciliaris. The anterior portion of the canine vitreous is strongly attached to the posterior lens capsule, which has given rise to the confusing term hyaloideocapsular ligament (i.e., Wieger’s ligament and Egger’s line). The vitreous is also attached to the region of the ora ciliaris retinae and to the prepapillary area. The vitreoretinal interface consists of outer part of the vitreous, anchoring fibrils of the vitreous body and the inner limiting membrane of the neuroretina. To completely observe and evaluate the vitreous, the pupil should be dilated using a short‐acting mydriatic agent, such as 0.5–1.0% tropicamide. In puppies, however, tropicamide may not cause adequate pupil dilation in all cases; use of 1% atropine may be indicated. The part of the ophthalmic examination dealing with the posterior segment of the globe should be performed in twilight conditions. The eye is both illuminated and inspected from a distance (≈40–60 cm). The light source should be powerful and focal, such as a penlight of good quality, a direct ophthalmoscope, or an otoscope, to allow any vitreal opacities or condensations to be visualized by both direct and indirect illumination (i.e., by light reflected from the tapetal fundus [tapetum fundus]). Because opacities will either partly or completely block the reflected light, they will be seen as dark structures against a bright tapetal reflectivity. Movement of vitreal structures following motions of the globe or lagging behind ocular motions may be determined more easily in this way. As the vitreous becomes more liquefied, opacities can appear unstable with the gel or even settle to the bottom of the vitreal space. A slit‐lamp biomicroscope is a highly useful and necessary tool for examination of the vitreous, even though such inspection using a slit‐lamp biomicroscope will be limited to the anterior part. A slit‐lamp biomicroscope is therefore especially useful to evaluate disorders at the lens/vitreous interface, such as persistence of parts of the tunica vasculosa lentis posterior, persistent hyaloid artery (PHA), persistent hyperplastic tunica vasculosa lentis (PHTVL)/persistent hyperplastic primary vitreous (PHPV), and posterior lental pathology. Examination of the posterior vitreous requires the Hruby contact lens with slit‐lamp biomicroscopy and can provide magnifications from 5× to 50× of the vitreous structure. The direct ophthalmoscope may be used as previously described. By decreasing the distance between the light source and the eye, direct illumination of vitreal structures will increase, enabling better visualization of their morphology. Vitreous liquefaction and floaters may also be visualized with an indirect ophthalmoscope. Ultrasonography, using a high‐resolution ultrasound machine, is a highly useful and noninvasive means of vitreal examination. This is especially true in eyes with opacities of the anterior segment of the globe that exclude visual examination of the vitreous. Only dense vitreal opacities, however, will be visualized during a B‐mode scan. Examples of abnormal structures in the vitreal cavity that may be visible ultrasonographically include hyaloid remnants, vitreal hemorrhage, vitreous degeneration, retinal detachment (RD), and intraocular neoplasia. Use of color‐flow Doppler may be useful to indicate blood flow in suspected structures. Contrast‐enhanced ultrasonography has been described to be a useful tool in the differentiation of RD and vitreous membranes in dogs and cats. In addition, computed tomography (CT) and magnetic resonance imaging (MRI) may contribute to the evaluation of vitreal disease. In diagnostic vitreal paracentesis (i.e., hyalocentesis), a small amount of liquid vitreous is aspirated for analysis, most often cytological examination or microbiological culture. Hyalocentesis is performed after instillation of a short‐acting mydriatic agent, preferably under general anesthesia, and after antiseptic pretreatment of the conjunctival sac (e.g., with a 0.5% povidone–iodine aqueous ophthalmic solution). A 22‐ to 26‐gauge (0.70–0.45 mm) needle is used to penetrate the sclera, and the exact location of needle penetration is of great importance. The eye should be penetrated via the anterior or medial region of the pars plana ciliaris. The corresponding external sites in medium‐sized mesocephalic dogs are 7 mm (i.e., superotemporal quadrant), 6 mm (i.e., inferotemporal quadrant), 5 mm (i.e., inferonasal quadrant), and 4–5 mm (i.e., superonasal quadrant) posterior to the limbus. There are indications that these sites will be more posterior in larger dogs with larger eyes. Needle penetration through the pars plicata ciliaris will result in considerable intraocular hemorrhage, whereas penetration posterior to the pars plana ciliaris will cause punctures of the retina. Because the vitreous is avascular in adult dogs, penetration of systemically administrated drugs is poor. When the blood–aqueous barrier is compromised, such as occurs in uveitis, accessibility of the vitreous for drugs increases. Hence, systemic administration of drugs, even though relatively safe, is of limited use in reaching the vitreous medically. The most direct way to achieve high drug concentrations in the vitreous is by intraocular injection. The globe only tolerates rather low doses and volumes, however, and large doses may produce severe ocular damage. The latter phenomenon is used by injecting gentamicin into the vitreous as a treatment in globes with absolute glaucoma and in which enucleation is contraindicated. Surgical treatment is necessary when the vitreous is displaced within the pupil during anterior segment surgeries and in the treatment of RDs. Additional details on vitreous surgery are provided later in this chapter. Vitrectomy is generally indicated when vitreous presentation or protrusion occurs during anterior segment surgery. Another indication for vitrectomy is during vitreoretinal surgery for the treatment of RDs and vitreal traction bands. Anterior vitrectomy is indicated when formed vitreous protrudes into the pupil or the anterior chamber during anterior segment surgery. In most cases involving dogs, protrusions occur during or directly after intracapsular lens removal, and during phacoemulsification of hypermature cataracts and placement of the intraocular lens (IOL). If the posterior lens capsule is perforated during cataract surgery, the vitreous may protrude into the capsular bag, pupil, anterior chamber, or even into the corneal incision. Protrusion of vitreous in the pupil or the anterior chamber may physically impair or obstruct the flow or drainage of aqueous, resulting in an increased intraocular pressure. In addition, vitreous touching the corneal endothelium may cause persistent corneal edema. Anterior vitrectomy can be performed manually, using microsurgical cellulose sponges or vitreous forceps, or it can be performed using an automated vitrectome. Left untreated, vitreous protrusions may cause the development of traction bands, with the risk of subsequent RD. Anterior vitrectomy is generally performed through an already existing corneal or corneoscleral incision or, seldomly, through a traumatic perforation. A second corneal incision in a different area occasionally is necessary for adequate access to the pupil. In selected cases, a pars plana vitrectomy (PPV) through a newly made surgical entrance (i.e., pars plana posterior vitrectomy) may be indicated. In pars plana posterior vitrectomy, part of the vitreous is removed using an automated vitrectome. Successful use of this method requires considerable experience and specialized instrumentation. The surgical entrance is where the dorsolateral sclera is positioned over the pars plana ciliaris. This procedure is commonly included in surgical treatment of complicated RD and vitreal traction bands. Other indications for this procedure include ophthalmomyiasis interna posterior and uveitis (mainly used in horses with equine recurrent uveitis). Developmental disorders form a group of relatively rare ophthalmic anomalies. Usually, these anomalies are part of syndromes associated with persisting intraocular vasculature or with other ocular developmental disorders, such as microphthalmia, Collie eye anomaly (CEA), and retinal dysplasia. The anomaly PHA results from failure of part or all of the hyaloid artery to regress (Figure 13.1). The artery may have persisted as a string (in some cases containing blood) situated in the vitreous space, between the optic disc and the lens. In PHA, only a small, dense, white, connective tissue string usually remains adhered to the posterior lens capsule. The optic disc may have a conical shape (i.e., Bergmeister’s papilla), and during ocular movements, PHA structures lag slightly behind and thus may show a “waving” motion. PHA has been suggested to be hereditary in the Sussex Spaniel and the Dobermann. Figure 13.1 PHA in a Golden Retriever. The hyaloid artery is visible as a white string (arrow) (Frans C. Stades). PHA alone rarely requires surgical treatment. If the associated cataract formation leads to visual impairment, however, surgery may be indicated. In these cases, the risk of complications is slightly higher than that in cases of cataract alone, because in PHA‐related cataracts, central fenestration of the posterior lens capsule and anterior vitrectomy are indicated. In persistent tunica vasculosa lentis, there are fine, white, strand‐like deformities (i.e., “spiderweb”), or parts of vascular structures, attached to the posterior lens capsule (i.e., persistent tunica vasculosa lentis posterior). These structures are the remainder of the tunica vasculosa lentis (posterior), retrolentally connected to, or “printed” on, the posterior lens capsule. These structures generally have no clinical significance, and they may be observed incidentally during routine ophthalmic examinations. In this group of apparently rare and generally unilateral disorders, parts of the hyaloid system and primitive vitreous have become hyperplastic during early fetal development, combined with a subsequently incomplete regression. This anomaly has been described in several species. In the dog, PHTVL/PHPV has been described in the Bloodhound, Bouvier des Flandres, Samoyed, Siberian Husky, and Spanish Pachon, and studied extensively in the Dobermann in Holland and in the Staffordshire Bull Terrier in England. PHTVL/PHPV generally occurs bilaterally, is inherited (probably because of an incomplete dominant gene in the Dobermann), and hence has a higher prevalence in these breeds compared with that in others. Signs range from very small, retrolentally positioned fibrovascular dots that represent minor remnants of the tunica vasculosa lentis vasculature (i.e., grade 1) (Figures 13.2 and 13.3). The differential diagnosis of PHTVL/PHPV includes cataracts resulting from other causes, microphthalmia alone, and other solitary dysplastic disorders of the lens. In severely affected blind eyes (i.e., grades 2–6), cataract surgery with fenestration of the posterior capsule and transection of the hyaloid artery, if applicable, combined with anterior vitrectomy may be indicated. The prognosis for surgery in severely affected cases of PHTVL/PHPV is less favorable than that in routine cataract surgery because of the higher chance of complications. The vitreous may be involved in diseases of its bordering structures as well. In CEA, a partial failure of vitreal development, which is only recognizable with use of a slit‐lamp microscope, has been described. Vitreal syneresis, vascular anomalies, hemorrhages, and RD may also be found in CEA. These anomalies may include preretinal vascular loops penetrating into the vitreous, and such vessels may easily give rise to vitreal hemorrhage. In case of RD, the neuroretina is partially or totally detached into the vitreous. Figure 13.2 Postnatal persistence of vasculature belonging to the hyaloid system, including the tunica vasculosa lentis posterior, as seen in the right eye of a Dobermann puppy with grade 3 PHTVL/PHPV (Frans C. Stades). Figure 13.3 Right eye of a two‐month‐old Doberman with PHTVL/PHPV. Note the presence of elongated ciliary processes at the 12‐o’clock to 2‐o’clock positions (yellow arrows), macrophakia, and cataract (blue arrow). In the focal and geographic forms of retinal dysplasia, the retina folds slightly into the vitreous. In severe forms of retinal dysplasia, as has been described for the Bedlington Terrier, Yorkshire Terrier, and Labrador Retriever (in combination with skeletal abnormalities), the retina is partly or totally detached and dislocated into the vitreal space. Penetrating trauma, such as caused by air‐rifle or shotgun pellets, easily causes floaters of blood in the vitreous. The penetration tunnel is usually marked by prolapsed lens material, blood residues, traction bands, and other scars, and the foreign body itself may still be present in the vitreous. Less often, blunt trauma causes vitreal hemorrhage. Dogs that show ophthalmic problems of sudden onset that include signs of trauma such as corneal edema, hyphema, uveitis, and vitreal hemorrhage should additionally be examined with diagnostic imaging techniques. Depending on the severity of the concurrent uveitis, therapy in such patients consists of topical antimicrobial treatment/prophylaxis, mydriatics/cycloplegics (e.g., 1% atropine), and 0.1% dexamethasone (four to six times daily), as well as systemic prophylactic antimicrobial and extensive anti‐inflammatory treatment (e.g., corticosteroids or nonsteroidal anti‐inflammatory drugs). In case of ocular hypertension, the intraocular pressure should be reduced as well, in which case use of parasympatholytic mydriatics, and especially atropine, would be contraindicated. The term “vitreous degeneration” is clinically used to indicate vitreous changes that are consistent with breakdown of the vitreous hydrogel (Box 13.1). Signs may comprise liquefaction (syneresis) and opacities (floaters, asteroid hyalosis, and synchysis scintillans). However, in “normal” dogs, a wide variety of vitreous gel consistencies can clinically be noticed. Over the last few years, an entity called primary vitreous degeneration has become an issue in a number of breeds, such as Brussels Griffon, Chihuahua, Chinese Crested, Havanese, Italian Greyhound, Lowchen, Papillon, Shih Tzu, and Whippet. However, there still is a lack of scientific literature concerning this entity in dogs. Small or large opacities that consist of conglomerates of calcium and lipids, condensations of vitreous fibrils, groups of erythrocytes, or pigment cells (including remnants of the hyaloid system) may persist or develop in the vitreous. Such opacities may move as well, following the movements of the eye. In older animals, further degeneration of the vitreous can lead to liquefaction, in which case the opacities have a greater tendency to lag behind ocular movements, whirl about, and then settle to the vitreous floor. Syneresis is a degenerative breakdown of the vitreous gel that separates its liquid from its solid components, resulting in liquefaction and development of fluid‐filled cavities within the vitreous. Syneresis may predispose to posterior vitreous detachment. Development of rhegmatogenous RD in the Shih Tzu has been suggested to occur through primary retinal degeneration and secondary vitreous degeneration (syneresis). Dogs older than 10 years often have some degree of vitreal syneresis. Vitreous floaters, muscae volitantes, or “flying flies” are larger flakes or streaks in the vitreous that may lag behind ocular movements. In the past, this was thought to cause the so‐called fly‐biting syndrome, but today, this behavior is suspected to result mainly from nonophthalmic causes, such as temporal or occipital lobe dysfunction. Vitreous floaters are uncommon and rarely require surgical treatment. Asteroid hyalosis is characterized by many small and possibly slightly pigmented particles (i.e., “asteroid bodies” from 0.03 to 0.10 mm in diameter) in the vitreous of one or both eyes (Figure 13.4a and b). These particles move both during and following movements of the globe, but they also return to their initial position. They generally have no distinct influence on vision by their presence alone; however, asteroid hyalosis may be associated with posterior uveal changes or (progressive) retinal atrophy. Comparisons of vitreous humor containing asteroid bodies from affected dogs indicate that asteroid bodies contain calcium and phosphorus. Figure 13.4 Asteroid hyalosis in a dog. (a) External appearance. (b) Ophthalmoscopic appearance (Frans C. Stades). Synchysis scintillans is characterized by numerous cholesterol particles in a more or less liquefied vitreous. Especially after eye movements, they can resemble a snow flurry behind the lens, and against bright light, they may cause dazzling. With ocular movement, these opacities move within the liquid vitreous; when the globe is till, they settle to the bottom of the vitreous space. The abnormality is rare and seldom causes recognizable problems in dogs. Intravitreal membranes are associated with past and/or current intravitreal hemorrhage. Additional common findings in that study included epiretinal membranes, retinal neovascularization, preiridal fibrovascular membranes, and glaucoma. Because the adult vitreous has no intrinsic vasculature nor nerve supply, the possibilities of reaction to noxae are limited. Hence, inflammation of the vitreous itself (i.e., hyalitis and vitritis) will almost always be secondary to inflammatory reactions of adjacent structures. Floaters of hemorrhagic or other exudate in the vitreous, especially if diffuse or bilateral, often result from exudative uveitis, chorioretinitis, retinitis, or optic neuritis. Free blood in the vitreous is relatively uncommon (Figure 13.5a and b). Hemorrhage may result from congenital anomalies, such as the persistence of fetal vasculature (e.g., PHA and PHTVL/PHPV), CEA, trauma, infection, or systemic diseases (e.g., coagulopathies, vasculitis, systemic hypertension, and neoplasms). Blood in the vitreous has been described to have a destructive effect on the gel structure: vitreous adjacent to a hemorrhage is prone to liquefaction. The arrival of macrophages induces an inflammatory reaction, which results in the formation of vitreal fibrinous membranes and traction bands. Depending on the geographic location of the patient, various infectious agents are known to induce vitreal exudates. Infections reported to cause vitreal exudates include blastomycosis, cryptococcosis, histoplasmosis, brucellosis, and other diseases. In these cases, therapy should be directed primarily at the underlying cause. Figure 13.5 Vitreal hemorrhage following trauma in a dog. (a) Limited hemorrhage. (b) More extensive vitreal hemorrhage. The prognosis of vitritis strongly depends on the underlying cause. Small amounts of blood or exudate may be resorbed, but larger amounts usually cause visual disturbances, even if the inflammatory reaction can be controlled. Vitreal membranes and traction bands may develop. In turn, vitreal displacement, prolapse, herniation, and traction caused by traction bands or membranes may cause secondary RDs even months after the primary disorder has resolved. Cysts, most likely originating from the pigmented epithelial layer of the iris or the ciliary body, may extend into the vitreous. These cysts are much less common than anterior chamber uveal cysts. Cysts may also be caused by parasites. Vitreal cysts have the appearance of spherical or oval, translucent bodies that may be stationary or move slowly in liquefied vitreous following movement of the globe. Depending on their size and location, they seldom interfere with vision. Migrating larvae of Dirofilaria immitis, Toxocara canis, the larvae of flies (i.e., ophthalmomyiasis interna), and Echinococcus sp. may penetrate the vitreous. Living or dead intraocular parasites may cause local irritation, uveitis, or cyst formation. Killing intraocular dirofilaria by medication is sometimes possible. However, the intraocular presence of a dead parasite generally induces severe immunogenic uveitis, with possible subsequent endophthalmitis. Therefore, if the parasite can be reached without an unacceptable risk, surgical removal is to be preferred. Intraocular neoplasms generally arise from the uvea and may occupy part, or almost all, of the vitreous space. Neoplasms may be primary, metastatic, or systemic/multicentric. Depending on location and size, a vitreal tumor may cause more or less displacement of the vitreous and lens as well as posterior uveitis and RD. Primary neoplasms of the retina, choroid, and optic nerve are extremely rare in the dog, and neoplasms originating from the anterior uvea are relatively uncommon in this species. Uveal neoplasms mainly comprise malignant melanomas, but other neoplasms have been reported. The prognosis for an eye affected by a neoplasm extending into the vitreous depends on both the type of neoplasm and its extension, but such a neoplasm generally should be considered an indication for enucleation. The lens may dislocate when its suspension system is compromised by dysplasia, degeneration, and/or rupture of the Zinn zonular fibers. The zonular fibers may have developed abnormally or have degenerated; in exceptional situations, they can rupture from external trauma. If several zonular fibers have ruptured, vitreous may leak into the anterior chamber along the lental equator and through the pupil. If the fibers have ruptured over a greater area, subluxation of the lens will occur. If the lens becomes completely unattached, it may remain more or less in its normal position, be displaced toward anterior or posterior, or topple. Because of its volume and the strong attachment of the posterior lens capsule to the vitreous, a dislocated lens will dislocate part of the vitreous as well. Subsequently, vitreous may prolapse or herniate in the pupil, thus impairing, or even blocking, drainage of aqueous between the posterior surface of the lens and the anterior surface of the iris (if the lens has become dislocated into the anterior chamber) or, at the level of the drainage angle, causing secondary glaucoma in all cases. The earliest recognizable sign of a lens luxation is the presence of slight vitreous protrusions, which are recognizable on slit‐lamp biomicroscopy as thin, white clouds along the pupil margin. Figure 13.6 Bullous RD in the left eye of a Bernese Mountain dog suffering from systemic hypertension. An RD may occupy part, or almost all, of the vitreous space. A small RD is observed as an indistinctly bordered, grayish blue “cyst” or fold of the retina. Large detachments look more like grayish blue to red, parachute‐shaped, vascularized bullae positioned either directly behind the posterior lens capsule or deeper in the vitreous. Depending on the type of detachment, the optic disc may be obscured. Also, dark sheets of blood, pigment, or other inflammatory signs may be found. The combination of intraocular (intravitreal) hemorrhage and RD may be indicative of systemic hypertension (Figure 13.6). Diagnostics should therefore include an internistic workup including blood pressure measurement. Ocular fundus diseases occur frequently in the dog. Inherited vitreal and retinal diseases are more frequent in the dog than in any other animal species as compared to man. Human and canine retinal diseases are often caused by the same DNA mutation, and as a result, the dog is often the best available animal model with a human‐size eye for investigations into their pathogenesis (looking for therapeutic windows) and possible gene therapy. Examination of the posterior segment starts with direct and/or indirect ophthalmoscopy, and with recent noninvasive diagnostic procedures can be imaged at very high levels of resolution (often approaching 10–20 μm). The ocular fundus is examined with various direct and indirect noninvasive diagnostic methods. Several methods are routinely used clinically, while others are more investigative. Behavioral testing is relatively simple and can be quantified by difficulty of the maze, time to complete successfully the maze, amount of illumination, and other measurable factors. Careful questioning of the owner is usually an important source of information about a dog’s visual performance; however, this information may be incomplete or invalid. Furthermore, the ability of a visually impaired or blind dog to adapt to familiar surroundings by relying on other senses and a memorized image of the environment can mask the signs of impaired vision. Vision may also be assessed from the dog’s response to falling objects (visual tracking) that do not create a noise or current of air (e.g., small cotton balls). The balls can be dropped both in front of the dog and to the sides to get an impression of central and peripheral vision. The results should be interpreted with some caution, however, because of patient indifference. The pupillary light reflex (PLR) requires a functional retina but also depends on postretinal transmission of signals. Loss of the PLRs is not a definite sign of retinal dysfunction or even of loss of vision. They can also be partially inhibited in an apprehensive patient because of an increased sympathetic tone. Furthermore, residual PLRs are often present in dogs even with advanced retinal degeneration, such as in progressive retinal atrophy (PRA), especially if the inner retina is preserved. The reason for this phenomenon is that the PLRs are driven by the photoreceptors at low light levels and have been shown in various species to be driven by the melanopsin‐containing ganglion cells at high light intensity stimulation. The presence of the PLRs is therefore not a guarantee of a healthy retina in dogs with opaque media (e.g., preoperatively in those with dense, extensive cataracts) that prevents ophthalmoscopic examination. Direct and indirect ophthalmoscopy are both used in veterinary ophthalmology to visualize the fundus. Three types of ophthalmoscopes are available: (i) the standard monocular direct ophthalmoscope; (ii) pan‐ophthalmoscope; and (iii) monocular and binocular indirect ophthalmoscopes. Each type of ophthalmoscope has advantages and limitations, and can complement each other. The ophthalmoscopic examination is performed in a darkened room using short‐acting mydriatics, such as 0.5–1.0% tropicamide (Mydriacyl; Alcon Laboratories, Ft Worth, TX, USA). Direct ophthalmoscopy, in general, provides the observer with a smaller field of view that is of greater magnification and detail than that with indirect ophthalmoscopy. The newer pan‐ophthalmoscope has lower magnification and a larger viewing field. Direct ophthalmoscopy is mainly used to examine the central parts of the fundus, especially the region of the optic nerve head (ONH), but it is considered inadequate to examine the peripheral fundus of domestic animals. Indirect ophthalmoscopy allows a more complete examination of the fundus in a shorter time. Moreover, it allows stereopsis, which is useful in appreciating the topographical features of the retinal surface such as when appraising RDs, colobomas, and papillary edema. Different indirect lenses can be selected to allow for a more highly magnified view when required. For additional details, see Chapter 4. Scanning laser ophthalmoscopy (SLO) is a diagnostic imaging technique developed for very precise and detailed fundus visualization. Lasers of different wavelengths allow the examiner to obtain information about specific tissues and layers on the basis of their reflection and transmission characteristics. The SLO can also be utilized in an angiography mode in order to correlate functional with morphological aspects in disease processes affecting the retinal and/or the choroidal vasculature. During the past decade, an imaging technique called optical coherence tomography (OCT) has been developed and used both in research and for clinical purposes to evaluate in vivo retinal structure. High‐resolution, cross‐sectional images of optical reflectivity are obtained. The technique is based on the principle of low‐coherence interferometry in which distance information concerning various ocular structures is extracted from time delays of reflected signals. This in vivo microscopy technique gives information about retinal organization and structural integrity. For example, photoreceptor layer thickness can be quantified using OCT. Instrumentation has also been developed that utilizes both OCT and SLO techniques simultaneously. Newer noninvasive imaging techniques are under development. Some use adaptive optics to adjust for the aberrations of the optical media allowing for highly magnified examination of the retina in vivo. Individual photoreceptors can be imaged using the adaptive optics techniques. Ultrasonography is a valuable aid in detecting and monitoring pathological conditions in the canine posterior segment. In patients with opaque media (e.g., dense cataracts and intraocular hemorrhage), ultrasonography can be used to detect, for example, a detached retina or posterior segment neoplasm. Furthermore, ultrasonography can be used to study space‐occupying conditions that are difficult to examine because of their location (e.g., neoplasms in the choroid close to the ciliary body). Angiography is a helpful adjunct in the diagnosis of retinal diseases. It is mainly used to evaluate disease processes in which the vasculature of the eye is involved, such as vascular anomalies, posterior segment neoplasms, hypertension, RD, inflammatory processes, diabetic retinopathy, and degenerative processes. The procedure is performed in sedated, anesthetized, or conscious dogs, depending on the preference and need of the examiner. Fluorescein sodium solution (Fluoresceine 10%; Faure, France) is given as an intravenous bolus into the cephalic vein. Fundus photography is then performed using a fundus camera connected to a power‐back unit to allow for quick recycling of the black‐and‐white or color film, as well as using the newer digital cameras. When the retina is stimulated by light, a diffuse electrical response is generated by neuronal and nonneuronal cells that can be recorded as the electroretinogram (ERG). This response is a summation of electrical potentials that result from light‐induced changes in the movement of ions, mainly sodium and potassium, within the extracellular space. Thus, the ERG is an objective, functional test of the retina and is critically dependent on the function of the photoreceptors (i.e., the rods and cones). It has a characteristic waveform that varies depending on several factors, but mostly on the type of stimuli used. The intensity, duration, frequency, and wavelength of the light stimulus as well as the interval between stimuli, size of the retinal area illuminated, pupil size, and (very important) stage of retinal adaptation are variables that account for alterations in the ERG response. The ERG is recorded by using an active contact lens electrode, and a reference and a ground electrode, and the results are displayed on an oscilloscope, ink writer, or computer screen (Figure 13.7). In clinical veterinary ophthalmology, the flash ERG is used in two broad applications. The first is to test whether a standard stimulus elicits an ERG response. This is useful in assessing retinal activity when the fundus is obscured (e.g., before cataract surgery) and in the differential diagnosis of retinal disease when ophthalmoscopic lesions are absent. The second is to specifically test rod and cone function in conjunction with research into retinal disease processes and in the early diagnosis of hereditary retinal degenerations and dystrophies. Procedures for standardization of ERG procedures have been adopted in human ophthalmology. Guidelines have also been developed for performing dog ERGs. The pattern ERG (PERG) measures a retinal response to a phase‐reversing pattern stimulus that is focused onto the retina and maintains a constant overall mean luminance. The response is an electrical potential thought to derive from the retinal ganglion cells (RGCs) and the neighboring inner retinal structures. The main characteristics of a pattern stimulus include overall screen brightness (i.e., mean luminance), brightness contrast of neighboring bars or checks (i.e., percent contrast), rate of pattern reversal (i.e., temporal frequency), and bar or check size (i.e., spatial frequency). Moreover, for measurements to be reliable, the refractive error of the patient must be corrected during the testing so that the stimulus is focused on the retina. Other methods to estimate canine visual acuity include behavioral testing, assessment of the optokinetic response, and measurement of visually evoked cortical potentials (VEPs). Figure 13.7 Bilateral ERG in progress using a protocol for evaluation of rod and cone function and Ganzfeld (full‐field) stimulation. (a) The dog is under general anesthesia (propofol/isoflurane) in order to obtain maximally stable recordings. (b) Details of the procedure are shown on the right, which starts with dark adaptation, whereafter the eye is stimulated by a low and a high intensity of white light. Light adaptation follows (using 37 cd/m2), and then recordings are obtained using single‐flash and flicker stimulation with white light as indicated. The VEP, or visually evoked response, is a gross electrical signal generated at the occipital cortex in response to visual stimulation. These responses are of small amplitude (1–40 μV) and are extremely sensitive to stimulus changes. Therefore, computer averaging is necessary for their recording, and correct placement of electrodes is a necessary factor in achieving correct and reproducible results. The VEP reflects the electrical activity of the central part of the fundus, because this area has a much larger cortical representation than the peripheral regions. Thus, in veterinary ophthalmology, the VEP is a test not only of the central visual function but also of the optic nerve and the higher visual tracts and visual cortex. It is useful in patients with normal ophthalmic examination results and a normal ERG. Regional functional testing procedures have been developed for both ERG and VEP recordings in order to obtain precise topographical mapping of disease. Both techniques allow for simultaneous measurements of ERG or VEP activity from many different retinal locations. The former technique utilizes SLOs for laser stimulation and direct visualization of the procedure with an additional stimulus in the optical pathway of the SLO by means of a wavelength‐sensitive mirror. Appendix A lists the tests currently available. However, this is a rapidly progressing field and the reader is advised to review the current literature and websites of the laboratories offering genetic tests for the most up‐to‐date information. The canine ocular fundus is a challenge for the examiner because of its enormous variation in normal ophthalmoscopic appearance (Figure 13.8a–j). Normal ocular fundus variations occur by age, breed, iris color, coat color, and other factors. The great range of normal variation in fundic appearance could, perhaps, be expected when the diversity in the gross appearance of different canine breeds is considered. The eyes of one individual are often mirror images of another, though marked differences may be seen in some dogs (e.g., one eye may have a subalbinotic fundus and one fundus with a brightly colored tapetum and pigmented nontapetal area in a Collie or Siberian Husky). The combination of the tapetum lucidum and an absence of pigment in the overlying retinal pigment epithelium (RPE) is the anatomical basis for the tapetal fundus. The tapetal fundus forms an almost triangular area, with a horizontal base, in the dorsal half of the fundus. The area is usually brightly and beautifully colored, and reflective. The size of the tapetal fundus varies extensively. Usually, it is large, and it may sometimes surround the ONH in gaze hounds (i.e., dogs that hunt by sight [e.g., Greyhounds]) and large breeds. It is often poorly developed, however, in toy breeds (e.g., Papillons). In the latter case, the tapetal fundus often only occupies a small area, usually temporal and dorsal to the ONH. The retinal blood vessels transverse the tapetal fundus, and the vessels are more easily viewed in the tapetal zone than in the nontapetal area when ophthalmoscopy is performed. The nontapetal fundus surrounds the entire tapetal area and is typically darkly pigmented. In dogs with a merle coat color (e.g., blue merle Collies, Shetland Sheepdogs, Australian Shepherds, and related breeds), the tapetal fundus may be absent. Occasionally, the tapetal fundus may also be missing in other dogs, and in this case, the entire fundus resembles the nontapetal zone (i.e., dark, dull, and nonreflective). The palette of the tapetal fundus includes hues of yellow, orange, green, and blue. Often, more than one color is present. Among breeds in which pale blue or heterochromic irides may appear (e.g., merle‐coated Collies, Shetland Sheepdogs, and related breeds, harlequin‐coated Great Danes, and Cardigan Welsh Corgis, which also carry merle genes, and some arctic breeds such as Siberian Huskies), a subalbinotic fundus may be found. The appearance of the fundus ranges from a small segment of albinism or subalbinism situated randomly in the tapetal or nontapetal fundus to almost complete absence of pigmentation in the fundus. The tapetal fundus has a light or dark blue color in young pups up to the age of five to seven weeks. After this age, the adult coloration starts to develop. The nontapetal fundus comprises the largest area of the ocular fundus in the dog. The junction between the tapetal and nontapetal fundi exhibits a continuous variation, from a distinct line of demarcation to a gradual transition with scattered foci of tapetal cells, which become more and more sparse with increasing distance from the center of the tapetal fundus. The nontapetal fundus has a nonreflective, usually dark or grayish brown to black color. In the dog (regardless of the breed) with a brown, chocolate, or liver coat color associated with a paler brown iris, the nontapetal fundus is less heavily pigmented and will appear paler brown or reddish brown. Sometimes, the choroidal vessels will cause a striped or tigroid appearance when viewed through the ophthalmoscope. Figure 13.8 Normal appearance of the fundus in different canine breeds. (a) A four‐year‐old Beagle with a large, mostly yellow tapetal area and a relatively large, round disc at the border of the tapetal and nontapetal areas. (b) A one‐year‐old Briard with a yellow to orange tapetal fundus and a disc at the border of the tapetal and nontapetal fundi. (c) A three‐year‐old Irish Wolfhound in which the circular disc is enclosed in the yellow to green tapetal fundus and the vessels are slightly tortuous. (d) A six‐year‐old Toy Poodle in which the tapetal fundus is extremely small and the disc is located in the nontapetal fundus. Some large choroidal vessels are visible (light red) underlying the retinal vessels (dark red). (e) A two‐year‐old Clumber Spaniel with a rather lightly colored nontapetal fundus, with a reddish coloration in the area caused by the underlying choroid. The border of the tapetal and nontapetal fundi is uneven, with some scattering of tapetal cells. (f) A six‐year‐old Cavalier King Charles Spaniel with sparse tapetal cells within the tapetal fundus. The uneven borders of the optic disc are caused by pronounced myelination. (g) A submelanotic right eye in a one‐year‐old Papillon. The regular striation of the choroidal vessels is seen against the white sclera, and there are no tapetal cells. The nontapetal fundus is pigmented. The fellow eye had an orange‐yellow “normal” tapetal and a pigmented nontapetal fundus. (h) A two‐year‐old blue merle Collie with an amelanotic fundus. There is a lack of tapetal cells and of visible pigmentation. (i) A four‐year‐old liver‐colored Labrador Retriever with a light brownish coloration of the entire fundus. No tapetal cells are visible. (j) A seven‐week‐old Collie pup with an immature, blue‐colored fundus. In the dog with a subalbinotic fundus, parts of the nontapetal fundus will be unpigmented, thus showing the choroidal vessels overlying the white sclera. Absence of pigment in the entire nontapetal region is common (e.g., in blue merle Collies). The canine ONH, or the optic disc or papilla, also exhibits a wide range of normal variations in ophthalmoscopic appearance. It is located in the center of the fundus, sometimes in the nontapetal fundus and sometimes in the tapetal region, depending on the extension of the latter. There is an obvious variation in size of the ONH between different individuals and different breeds. There is no strict relationship, however, between breed or size of the dog and the size of the ONH. The extent of myelinization affects the shape as well as the size of the ONH. In young pups where the myelinization is incomplete, the ONH is smaller than that in the adult dog. The shape of the ONH may be round, oval, triangular, or polygonal, and sometimes the disc edge may be clearly indented. The edge may be sharply demarcated to a zone of diffuse transition at which the medullated nerve fibers extend into the surrounding fundus, especially along the retinal blood vessels. The anterior surface of the ONH is elevated compared with the surrounding retina in the adult dog, whereas this topographical difference can be difficult to detect in very young pups with poorly myelinated nerves. The anterior surface rarely is perfectly flat, and an obvious, central prominence of medullated nerve fibers can be seen in some dogs, especially Golden Retrievers and German Shepherds. In the center of the ONH, a minute, round depression is usually seen, which is the physiological cup. The physiological cup, which usually appears grayish in color, represents the origin of the hyaloid vasculature. The color of the ONH varies from pinkish white to deep pink, depending on the extent of visible vasculature. The vessels climb the ONH, and a usually incomplete venous circle is seen on its top. The vascular architecture of the canine fundus is classified as holangiotic. The vasculature consists of arterioles and venules located at the surface of the retina. The diameter of the vessels decreases with increasing distance from the ONH; otherwise, no differences should be observed with location. The arterioles (usually 15–20 in number) radiate away from their origin close to the periphery of the ONH. They appear slightly lighter in color compared with the vessels transporting venous blood, and they may be more tortuous than the venules. The main veins (usually three or four in number) are obviously larger and darker red in color than the arterioles. They end in the usually incomplete venous circle on the top of the ONH but may, in part, be covered by the ONH tissue. Several smaller venules coalesce with the major veins, thereby building a branching tree of vessels over the optic fundus. The area centralis is an indistinctly bordered area slightly superior and about two optic nerve head diameters temporal to the ONH, which is devoid of blood vessels but encircled by fine branches. The difference between the immature ocular fundus of a young pup and the fully developed, functional fundus of an adult dog is striking (Figure 13.9a–c). The process of development includes both a successive series of morphological events and a functional maturation of the retina. It is normally impossible to examine the ocular fundus in pups younger than two weeks, because the eyelids remain closed and the ocular media, especially the cornea, are not sufficiently clear. When the eyelids open and ophthalmoscopic examination can be performed, there is no discernible difference between the tapetal and nontapetal fundi. At this stage of development, the entire ocular fundus has a dull, dark gray color, and the ONH is small and flat due to immature myelination. The retinal blood vessels, however, are easily identifiable and appear relatively large in size. Figure 13.9 Maturation of the canine fundus: (a) 5 weeks of age; (b) 9 weeks of age; and (c) 12 weeks of age. The developing tapetal fundus appears paler, and the nontapetal region relatively darker, after three to four weeks of age. The tapetal fundus then takes on a lilac to blue coloration, which becomes more intense with increasing age. After approximately seven to eight weeks, the tapetal fundus becomes more granular in appearance. The bluish, immature tapetal fundus then becomes more brightly colored over time, until a tapetal fundus of adult structure and coloration is developed at approximately three to four months of age. Retinal differentiation and maturation (mainly of the photoreceptors) in dogs is complete by seven to eight weeks of age. At the end of this period, the retina, as monitored by ERG and both light and electron microscopy, is adult‐like. Congenital abnormalities of the eye can either be hereditary or nonhereditary. CEA is a congenital ocular syndrome involving defects of the posterior vascular and fibrous tunics of the eye. CEA affects primarily the Collie types of dogs but also some other breeds: Rough and Smooth Collie, Shetland Sheepdog, Australian Shepherd, Border Collie, Nova Scotia Duck Tolling Retriever, Longhaired Whippet, Boykin Spaniel, and the Lancaster Heeler. The pathogenesis of the defect is considered to be an abnormal mesodermal differentiation, which results in defects, mainly of the sclera, choroid, optic disc, retina, and retinal vasculature. The severity of the disease varies from no apparent visual deficit to total blindness. CEA is bilateral and has no sex predisposition. There is no difference in frequency related to coat color, type of coat, or presence of the merling gene. The cardinal sign is a geographically defined region of choroidal hypoplasia lateral to the optic disc, which may or may not be accompanied by obvious retinal or scleral defects or by colobomas. Other anomalies are RD and intraocular hemorrhage. The hereditary trait for the disorder was established and a simple autosomal recessive inheritance postulated by Yakely and colleagues through a test breeding scheme. This mode of inheritance was further supported by the results of pedigree analysis and test matings conducted by Bedford in 1982. The genetic studies have established the primary CEA phenotype, choroidal hypoplasia, to segregate as an autosomal recessive trait with nearly 100% penetrance. Some heterozygotes for the mutant allele showed mild choroidal hypoplasia. The CEA locus was mapped to a 3.9‐cM region of canine chromosome 37. An intronic deletion in the NEHJ1 gene was identified. The prevalence of CEA has been high in Rough and Smooth Collies and Shetland Sheepdogs throughout the world. In 1969, the incidence of CEA in the Collie was between 75% and 97% in the United States. Because of selected breeding, this prevalence decreased within several years to 59%. The incidence of CEA in Border Collies is low, and only sporadic cases are diagnosed, while in the Lancashire Heeler, a somewhat high incidence was reported: 13.7%. Clinical findings in CEA include a high degree of pleomorphism. In a single litter, there is often great variation in the severity of the changes and there may be variation between the eyes of an affected individual. Choroidal hypoplasia is considered the diagnostic lesion for CEA and is always bilateral, though the extent of the lesion may vary between eyes (Figures 13.10 and 13.11). The four main defects included in the CEA syndrome are listed in Table 13.1. Other changes also included in this syndrome by some authors are excessive tortuosity of the retinal vasculature and retinal folds in young pups. The CEA syndrome is most accurately diagnosed in pups at six to seven weeks of age. These same dogs should be examined annually (to confirm the puppy exam results) as well as before their first breeding. Figure 13.10 Coloboma in conjunction with a partially deformed disc and choroidal hypoplasia in a two‐year‐old Collie. Figure 13.11 RD is part of the CEA complex. Partial RDs near the disc as well as vascular abnormalities in a young Collie. Now that the causal gene mutation has been identified and a commercial mutation detection test has been developed, breeders will be able to eventually eliminate the condition (by identification of carrier dogs) and preserve the best of their breeds’ other characteristics. The high incidence of CEA in some breeds dictates that breeders should only attempt to reduce the mutation incidence within the breed over several generations. Restricting breeding to homozygous normal dogs could limit the gene pool and could lead to loss of desirable features from the breed and possibly the emergence of a new hereditary disease that was present at low frequency within the breed. To avoid the production of CEA‐affected puppies, breeders just need to ensure that one of the breeding pair is homozygous normal. Difficulties in trying to eliminate the condition by only ophthalmoscopic screening have been partly due to certain difficulties in diagnosis. The “go‐normal” phenomenon was a major issue with genetically affected animals being indistinguishable from unaffected animals by ophthalmoscopic examination as described previously. Another problem of diagnosis is the differentiation between a small, centrally located coloboma in the optic disc and a normal physiological pit. Color‐dilute animals can also cause problems in making an ophthalmoscopic diagnosis of CEA when only mild choroidal hypoplasia is present. In merles, it is important to closely evaluate the area temporal to the optic disc for changes due to choroidal hypoplasia. Table 13.1 Ophthalmoscopic findings in Collie eye anomaly. The Australian Shepherd dog is affected by multiple ocular anomalies. Affected dogs may show microphthalmia to varying extent and varying size of the globes. The defect is associated with microcornea of an irregular shape and, infrequently, with mineralization of the anterior corneal stroma. Affected dogs also exhibit heterochromia irides with dyscoria and corectopia with an oval pupil in which the long axis is vertical. The hypoplastic irides permit transillumination and respond poorly to mydriatics. False polycoria occurs in 5–10% of affected dogs. Often, the defect extends into the iridocorneal angle and posteriorly into the ciliary body. Cataracts occur in 60% of affected dogs with microphthalmia and colobomas. In 54% of affected Australian Shepherds, large equatorial staphylomas occur. The staphylomas are either a single depression or several small excavations grouped together. The choroidal vasculature is greatly reduced or absent in the staphylomas, and the sclera is irregular and thin. Large staphylomas may extend from the ora serrata to the optic disc. Retinal vasculature in the staphylomas may be markedly reduced or even absent. In 50% of cases, complete RD occurs, and approximately 30% of the detachments have intraocular hemorrhage. Microphthalmia with colobomas in Australian Shepherds is inherited as an autosomal recessive trait with incomplete penetrance. Affected dogs are homozygous merles and have excessively white hair coats. A similar syndrome has been observed but not studied in merle Collies, Shetland Sheepdogs, Harlequin Great Danes, and other merle breeds. In general, affected puppies result when both parents are merles (sometimes not very noticeable). Retinal dysplasia has been defined as an anomalous differentiation of the retina accompanied by a proliferation of one or more of its constituent elements. It is characterized histopathologically by linear folding of the sensory retina and formation of rosettes composed of variable numbers of neuronal retinal layers around a central lumen. The defect is associated with several spontaneously occurring and experimentally induced disorders of the developing eye in both humans and animals. Dysplastic retinal lesions may be associated with systemic abnormalities, or they can be a primary ocular defect, either as a single defect or together with other lesions such as microphthalmos or colobomatous defects. Known causes of retinal dysplasia include viral infections, vitamin A deficiency, X‐ray irradiation, certain drugs, and intrauterine trauma. Many forms in dogs have a hereditary basis. The pathogenesis for spontaneous retinal dysplasia may vary, depending on the retinal tissues affected. Often the different forms of retinal dysplasia occur in specific breeds (Tables 13.2 and 13.3). Clinically, the lesions of retinal dysplasia are unilateral or bilateral and may be grossly divided into three different types or forms (see Table 13.3). Clinically, most lesions in retinal dysplasia do not appear to change with time. The least severe type (i.e., focal or multifocal retinal dysplasia) usually remains unaltered (Figure 13.12). In a few cases, however, the lesions become less obvious, and some folds may disappear. At the same time, the area around others, especially if the lesions are numerous, may become more demarcated, with hyperpigmented spots and streaks as well as hyperreflective areas in the vicinity of the dysplastic lesions. In the geographic type, the lesions never disappear but instead become focally more demarcated with time. The most severe form, with complete RD, remains unaltered in most cases, but complications including vitreal hemorrhage, cataracts, and secondary glaucoma may arise (Figure 13.13a–c). Table 13.2 Ophthalmoscopic findings in canine retinal dysplasia. Table 13.3 Breeds of dogs affected with retinal dysplasia (RD). AD, autosomal dominant trait with variable penetrance; AR, autosomal recessive trait. Since retinal dysplasia is a defect of retinal differentiation, lesions are mainly congenital in nature. Therefore, early screening of dogs at six to eight weeks has been encouraged for breeds in which the defect is prevalent. A second examination was recommended to be performed between six months and one year of age in breeds affected with the geographic form of retinal dysplasia. Figure 13.12 Multifocal retinal dysplasia in a one‐year‐old Labrador Retriever. Several grayish hyporeflective streaks and spots are seen in the tapetal fundus. Spontaneous retinal dysplasia occurs in several breeds, and hereditary factors have been shown or suspected to be the cause in many breeds (see Table 15.3). It is not uncommon to find retinal dysplasia in conjunction with other severe ocular defects, as described in the Doberman Pinscher, Akita, Chow Chow, and Australian Shepherd dog. In some breeds, such as in the Samoyed and the Labrador Retriever, retinal dysplasia occurs in combination with other nonocular defects (termed canine oculoskeletal dysplasia). Labrador Retrievers and Samoyed dogs are affected by another inherited syndrome, canine oculoskeletal dysplasia, in which there are both ocular lesions and skeletal abnormalities. Cataracts and complete RD are seen together with retarded growth of the radius, ulna, and tibia; separated hypoplastic anconeal and coronoid processes; hip dysplasia; and delayed development of the epiphysis. In the two breeds, the disease loci are nonallelic. They have been termed dwarfism with retinal dysplasia type 1 (drd1) for the Labrador type and dwarfism with retinal dysplasia type 2 (drd2) for the Samoyed. Dwarfism with retinal dysplasia results from mutations in the collagen 9 genes COL9A2 and COL9A3. drd1 in the Labrador results from an insertional mutation in COL9A3, whereas drd2 in the Samoyed results from a deletion in COL9A2. Identification of the mutations has allowed the development of DNA‐based genotyping tests (see Appendix A and for up‐to‐date information consult the European College of Veterinary Ophthalmologists [ECVO] Hereditary Eye Diseases Manual [http://ECVO.org] and/or http://Optigen.com). A clinical syndrome of congenital retinal dysplasia and persistent primary vitreous occurs in the Miniature Schnauzer. Through analysis of an extended pedigree and by test breeding, a simple autosomal inheritance for the disorder has been postulated. In affected dogs, the retinal dysplasia is usually generalized, involves the temporal and nasal retina most severely, and is most extensive dorsal to the optic disc (Figure 13.14a–c). Concurrently with the dysplastic retina, many dogs also show mild unilateral or bilateral PHPV. The PHPV changes are usually associated with small, white posterior lens capsule plaques, manifested as a central posterior lens capsule ring. Infection with canine adenovirus has been shown to cause retinal dysplasia. Another infectious agent causing retinal dysplasia is canine herpesvirus. Experimental studies have demonstrated that severe ocular inflammation with subsequent retinal dysplasia and associated ocular anomalies can be induced in neonatal pups. The immature mammalian retina is also susceptible to radiation, which has been demonstrated in the dog. Irradiated newborn puppies exhibited retinal rosette formation. The rosettes were either few or numerous, and they involved both tapetal and nontapetal fundi. Morphological studies showed lesions indicative of aberrant development after radiation‐induced damage. Figure 13.13 Oculoskeletal dysplasia in a Labrador Retriever. (a) Note the angular limb deformities. (b) Bilateral cataract formation is obvious. (c) Ocular ultrasound reveals the presence of RD. Figure 13.14 Retinal dysplasia and PHPV in a young Miniature Schnauzer. (a) There are posterior lenticular opacities, mainly manifested by a lens capsule ring, with some centrally located opacities. (b) White primary vitreous strands are seen, and a pertinent hyaloid vessel extends to the Bergmeister’s papilla. (c) Histopathological section of the posterior segment with severely dysplastic retina. Retinal dysplasia includes a diverse group of entities with variable clinical and histopathological appearances, variable pathogeneses, and variable etiologies. Retinal dysplasia is an abnormality that can occur in conjunction with many other eye anomalies, with or without systemic anomalies, either unilaterally or bilaterally. The clinical appearance of retinal dysplasia is often similar regardless of the cause in selected breeds. Among the breeds in which the defect has been recognized to be inherited, certain characteristic features, such as the central, mainly tapetal location of the folds and rosettes, are evident, which simplifies diagnosis in these breeds. The high frequency of retinal folds among the eyes of pups in some breeds, particularly the Collie and Shetland Sheepdog, has sometimes been interpreted as being a very mild form of retinal dysplasia. Such folds, however, usually disappear as the eye grows, and they probably represent uncoordinated growth of the inner and outer layers of the optic cup. As yet, there is no evidence for genetic determination except on a breed basis, and there is probably an association with the small eyes typical of these breeds. Retinal dysplasia represents abnormal differentiation of the retina and therefore would be expected to be permanent; however, in the young puppy (six to eight weeks old), diagnosis can be difficult. Thus, a follow‐up of these funduscopic alterations in dogs 6–12 months of age could indicate whether the lesions should be designated as retinal folds or retinal dysplasia. A unique inherited multifocal serious and bullous retinopathy was first described in the Great Pyrenees breed. Similar changes were then observed in the Coton de Tulear, English and French Mastiff and Bullmastiff dog breeds, and the Lapponian Herder. Pedigree analysis and prospective matings in the Great Pyrenees dog indicate an autosomal recessive inheritance for the disorder. Ophthalmoscopic lesions have been described as multifocal, gray to tan fundic patches that vary in size from barely visible to some lesions that are larger than the optic disc. Lesions start to develop at approximately 11 weeks of age; usually they are sparse, but they develop bilaterally in the peripheral tapetal fundus, around the optic discs, and occasionally under the major veins inferior to the optic disc (Figure 13.15). The peripapillary lesions, observed as serous RDs, develop within a few hours but remain the same size for years, while the peripheral lesions develop gradually over years and appear to increase slowly in size and number until the age of 20 weeks. The lesions do not appear to be congenital but are detected suddenly in young puppies. The retinal degeneration and RPE changes are focal and related only to the serous retinal and RPE detachments. An investigation of potential candidate genes revealed that affected dogs had a mutation in the canine BEST1 gene (cBEST1). In humans, mutations of this gene cause vitelliform macular dystrophy type 2, or Best macular dystrophy. The cBEST1 gene is predominantly expressed in RPE/choroid and encodes bestrophin, a 580‐amino acid protein of 66 kDa. Two disease‐specific mutations were identified in the cBEST1 gene of affected dogs, cmr1 mutation in mastiff‐related and the Great Pyrenees dog breeds, and cmr2 in the Coton de Tulear dog breed. Recently, a third form of cmr disease was identified in the Lapponian Herder breed, although this also resulted from a mutation in cBEST1. It manifested as a somewhat later onset of the multifocal lesions, at approximately one year of age. Figure 13.15 Canine multifocal retinopathy. An eight‐year‐old Mastiff with multiple bullae with tan‐colored subretinal fluid. All cellular layers of the retina are potentially susceptible to hereditary abnormalities. Most of these, however, relate to the parts that are physiologically the most complex: the layers of the photoreceptors and the RPE. In the dog, there is a broad range and diversity of inherited retinal diseases that affect these structures. Canine retinal degenerations primarily affect the photoreceptors, RPE, or both. The first well‐documented report of an inherited retinal degeneration in a Gordon Setter from Sweden was in 1910. To these conditions, the general term PRA has been applied. Since then, clinicians have divided PRA into two types depending on the ophthalmoscopic appearance of the fundus lesions: generalized PRA and central PRA (CPRA). In generalized PRA, there is generalized hyperreflectivity of the retina at the end stage of the disease, indicating a generalized atrophy of the neural retinal structures and clinical blindness. In CPRA, there are multifocal accumulations of pigment within the retina, and encircling these changes are areas of hyperreflectivity at the end stage. The latter disorder is the result of a primary defect in the RPE and does not always lead to blindness. Thus, the two disease entities represent completely different disorders. During the last few decades, PRA has been subdivided further into more specific diseases at the cellular level and also at the molecular level, and gene symbols are used to differentiate these disorders (see Appendix A). The term CPRA has been replaced by the term retinal pigment epithelial dystrophy (RPED), which more specifically relates to the disease entity. The PRA diseases are subdivided grossly into developmental and degenerative diseases. The developmental class represents a large aggregate of genetically distinct disorders expressed cytologically during the postnatal period, when the visual cells are beginning to differentiate, i.e., dysplasia of the rod or cone photoreceptors (or both), and each has its own unique disease course and phenotype as assessed by functional and morphological criteria. Typical for the photoreceptor dysplasias is that before the retina is adult‐like (approximately eight weeks of age in the dog), they show rather severe structural alterations of the rod or cone photoreceptor cells, and the rate of progression and loss of photoreceptors in the disease process is most often rapid. In contrast, the degenerative diseases represent defects in which photoreceptor cells degenerate after having differentiated mainly in a normal way. In the latter group, disease occurs more slowly and is modified by temporal and topographical factors. One form of primary photoreceptor degeneration (progressive rod–cone degeneration, prcd) is known to be allelic in many breeds of dog, and the underlying gene mutation has been identified. Within the photoreceptor dysplasia and degeneration groups, the diseases are subdivided still further according to the type of cell primarily affected (e.g., rod–cone dysplasia of Irish Setters and cone degeneration of Alaskan Malamutes). Clinical manifestations of the different forms of PRA are often remarkably similar, irrespective of the type of disorder present at the cellular level. Nonspecific features associated with primary photoreceptor degenerations leading to retinal atrophy are described briefly here; particular features associated with specific genetic disorders are described later in the text. PRA is always bilateral and almost always leads to blindness. The most common clinical sign of early disease is impaired vision in dim light and darkness (i.e., night blindness), since it is most often the rods that are affected first in PRA. The reduced visual capacity in darkness may often be observed by visual testing, which is performed by testing the menace reaction, using falling cotton balls in front of the dog in a dimly lit room, or using an obstacle course, or by using a specially designed forced‐choice vision testing device. In some instances, impaired day vision (day blindness) may be observed by the owner or the examiner as an initial clinical sign if the cone system is involved primarily in the disease process. The ophthalmoscopic changes for PRA are summarized in Table 13.4, and divided into early, moderate, and advanced (Figures 13.16–13.19). Secondary cataract often develops in the more advanced stages of disease. The initial changes tend to occur in the posterior cortex and include vacuolation and opacification. Often, irregular radiations are seen emanating from the posterior pole of the lens and extending to involve the equatorial cortex and, later, the entire lens. Mature cataracts, especially in certain breeds, make the evaluation of retinal function using ERG of utmost importance before cataract surgery is performed in order to rule out retinal degeneration (Figure 13.20). Table 13.4 Ophthalmoscopic changes in PRA. Figure 13.16 Moderately advanced cases of bilateral retinal degeneration (PRA) in two different canine breeds. Note the generalized change in reflectivity or hyperreflectivity and vascular attenuation in both cases. (a) A three‐year‐old Irish Wolfhound. (b) A four‐year‐old Dachshund. Figure 13.17 Fundus photographs showing progressive fundus changes in a Papillon with PRA: (a) 6 months of age; (b) 12 months of age; and (c) 24 months of age. Note the progressive retinal vasculature attenuation and initial tapetal hyporeflectivity that is apparent prior to development of generalized tapetal hyperreflectivity. Figure 13.18 An advanced case of retinal degeneration (PRA) in a five‐year‐old Tibetan Terrier. (a) The tapetal fundus is hyperreflective, and only ghost vessels are visible. (b) The nontapetal fundus is depigmented and hyperpigmented in striae and patches. Figure 13.19 Advanced prcd observed in a seven‐year‐old Miniature Poodle. (a) Central part of fundus, with severe vascular attenuation and a grayish tapetal discoloration. (b) Midperipheral fundus, with hyperreflectivity and discoloration in the peripheral part. (c) Periphery of the tapetal fundus, with a marked striation. The contours of the choroidal vascular pattern are observed underlying the atrophic neural retina. The characteristics for the retinal photoreceptor dysplasias in the dog are summarized in Table 13.5. Rod–cone dysplasia type 1 (rcd1), initially described in the Irish Setter, but also found in the Irish Red and White Setter, is an early‐onset, recessively inherited disease. It is characterized by arrested differentiation of visual cells resulting from a nonsense mutation in the β‐subunit of cyclic guanosine monophosphate (cGMP) metabolism. The defect in Irish Setters is similar to that in the retinal degeneration, rd1 mouse, in which the β‐subunit of cGMP‐PDE (PDE6B) was identified by Bowes and colleagues as being defective. The PDE6B gene was subsequently considered to be a candidate gene for the rcd1 defect. Figure 13.20 Representative dark‐adapted (a–d) and light‐adapted (to a background light of 30 cd/m2) (e–h) ERG intensity series responses from a normal control dog (indicated by the symbol +/+) (a, c, e, and g) compared to a PDE6A mutant/rcd3 dog (indicated by the symbol 2/2) at three weeks (a, b, e, and f) and seven weeks (c, d, g, and h) of age. Arrowheads indicate the onset of flash. The flash intensity is indicated in log cd s/m2. Note the difference in scales between the tracings. The vertical scale bar indicates amplitude in microvolts and the horizontal bars time in milliseconds. The rcd3 puppies do not develop normal rod‐mediated responses, and there is a rapid loss of the small amplitude response that is recordable. Much of this response is likely to represent cone responses. The light‐adapted (cone) responses are also reduced from an early age. The cone a‐wave is reduced at three weeks, and both a‐ and b‐waves at seven weeks. The disease was the first form of PRA to be described clinically in great detail, first in England and then in the United States. Night blindness is an early behavioral sign, detectable from six to eight weeks of age. Day vision is usually lost during the first year, but there is considerable variation in this respect. The first ophthalmoscopic signs are usually visible by three to four months of age, and the end stage, with generalized atrophic changes, is reached at approximately one year. The ERG is nonrecordable at approximately 18 weeks of age. A different mutation in PDE6B has been identified as a cause of PRA in Sloughi dogs. The disease in Sloughi dogs has similar clinical and morphological characteristics as in the early‐onset disease of Irish Setter dogs, and it has been suggested that it be given the gene symbol rcd1a. Mutation‐based tests are available for rcd1 for Irish Setter and for rcd1a for Sloughi dogs (see Appendix A and www.optigen.com). Rod–cone dysplasia type 2 (rcd2) has been described in the Collie and is another recessively inherited, early‐onset retinal degeneration (Figure 13.21). Based on clinical, electrophysiological, morphological, and biochemical criteria, rcd1 and rcd2 are very similar diseases. There is an equally early and rapid increase in retinal cGMP levels in the postnatal period, and the magnitude of this increase and the time course are similar. There is deficient cGMP‐PDE activity in both diseases, though in rcd2, the activity is calmodulin‐independent. The two dysplasias of Irish Setters and Collies represent mutations of different genes. With the advantage of having an early molecular diagnosis in rcd1, it was established that the rcd1 mutation was not present in rcd2. Recently, the rcd2 mutation was discovered in the C1orf36 gene (now named RD3). Mutations in the homologous gene are responsible for a comparable disease of humans and murines (retinal degeneration 3, rd3). Clinically, night blindness is the earliest sign, detectable by six weeks of age. Visual capacity is gradually reduced over time, just as in affected Irish Setters, and affected dogs become blind, usually before one year of age. Table 13.5 Characteristics of the canine retinal photoreceptor dysplasias. AR, autosomal recessive trait; ERG, electroretinography. Figure 13.21 Right eye of a Collie with both PRA and CEA. Note the choroidal hypoplasia lesion and the marked attenuation of retinal vasculature. The tapetal fundus is hyperreflective. Just as in Irish Setters, Sloughis, and Collies with the rod–cone dysplasias types 1 and 2, respectively, the disorder in the Cardigan Welsh Corgi is of early onset, leading to blindness in young adult dogs. The causal mutation is a 1‐bp deletion in codon 616 of the α‐subunit of rod cGMP phosphodiesterase (PDE6A). There is a mutation detection test available for the disorder. Ophthalmoscopic changes are present by 12 weeks of age. A recessively inherited form of retinal dysplasia was described in the Norwegian Elkhound many years ago. Subsequently, the mutant strain was lost. The disease was first described as “photoreceptor abiotrophy,” but later studies, however, showed developmental abnormalities, specifically of rods. Clinically affected dogs became night blind at six months of age, and visual problems progressed to total blindness at three to five years. Early retinal degeneration is a recessively inherited condition in the Norwegian Elkhound. Abnormalities in retinal cyclic nucleotide metabolism as well as mutations in the PDE6B gene were excluded for early retinal degeneration by breeding and molecular studies. The gene defect was mapped to a region of the canine genome that was subsequently found to be canine chromosome 27. Recently, a mutation was found in the STK38L gene, affecting a pathway important for neural and photoreceptor cell function. Clinically affected dogs are night blind by 6 weeks of age and blind by 12–18 months. Early ophthalmoscopic alterations are present by six months and progress to atrophic changes, which are observed at approximately one year. Photoreceptor dysplasia of Miniature Schnauzers is an unusual disease of the photoreceptors that, through pedigree analysis and test mating, has been shown to be inherited as an autosomal recessive trait. The disease is unusual in that it has a slow clinical progression when assessed with behavioral testing and ophthalmoscopy. When judged with histopathology and ERG, however, it is an early‐onset disorder. Clinically affected dogs show initial night blindness, followed by progressive loss of day vision. Ophthalmoscopically, changes in the fundus of affected animals are complicated by the unusual and variable appearance of the tapetal fundus in this breed. Diagnosis on the basis of ERG is possible from an early age. The mutation responsible for PRA in the Miniature Schnauzer is designated as type A PRA (www.optigen.com). There is a mutation‐based test available for this disorder. The cone–rod dystrophies are a heterogeneous group of inherited retinal degenerative disorders naturally occurring in humans and in dogs. The diseases are characterized by simultaneous involvement of cone and rod photoreceptors, the former being more severely affected than the latter, initially. So far, the disorder has been described in comparatively few canine breeds. These two forms of cone–rod dystrophy (crd) were named crd1 and crd2. crd1 is reported in the American Staffordshire Bull Terrier and crd2 in the American Pit Bull Terrier. The crd2 mutation has been identified and a DNA‐based test offered, although the mutation was not published at the time of writing (www.optigen.com). Recently, crd was identified in the Glen of Imaal Terrier and designated as crd3. Thorough clinical and laboratory studies of the Glen of Imaal Terrier have shown early fundus changes at the age of three years. In the Short‐Haired Dachshund, an early‐onset, recessively inherited crd has been described including clinical and electrophysiological findings. Already at the age of five weeks, the cone system is affected with severely reduced or nonrecordable cone‐derived ERG responses, while rod responses are either normal or only slightly reduced at this early age. A novel gene for this early‐onset crd has been described: a deletion in the nephronophthisis 4 (NPHP4) gene, also known as nephroretinin, causing simultaneous eye and kidney disease in humans, and naturally occurring crd without additional kidney disease in the canine. In the Long‐Haired Dachshund, the clinical and morphological characteristics of a rod–cone dystrophy were described. This was given the gene symbol cord1. Ophthalmoscopic changes were observed at the age of six months and included changes in the granular appearance of the fundus followed by tapetal hyperreflectivity and retinal vascular attenuation. Cone–rod dystrophy in the Miniature Long‐Haired Dachshund has been reported to be due to a mutation in the RPGRIP1 (retinitis pigmentosa GTPase regulator‐interacting protein 1) gene. These studies were based on an inbred colony of dogs. Recent studies have suggested the situation may be more complex. Some dogs homozygous for the RPGRIP1 mutation do not appear to develop retinal disease. The “classical type of PRA” that is termed progressive rod–cone degeneration (prcd) has an autosomal recessive inheritance. The defect has been known for some time to be an allelic condition in many dog breeds with late‐onset type of PRA (www.optigen.com). A mutation in a previously unidentified gene, PRCD, has been identified and expression studies showed that this gene is predominant expressed in the retina, with equal expression in the RPE, photoreceptor, and ganglion cell layers (Table 13.6). Interestingly, a human retinitis pigmentosa patient was identified with an identical mutation in the human PRCD gene. In the Toy and Miniature Poodles, structural and functional abnormalities in prcd do not become evident until after the visual cells have developed normally. The frequency of prcd in Poodles has been high over the years. Night blindness is the first behavioral sign, being observed in affected dogs usually at three to five years of age, but there is considerable variation in onset. Night blindness is followed by reduced vision in daylight and, finally, by complete blindness, which occurs at a variable age, usually between five and seven years. Development of a secondary cataract may exacerbate vision loss and therefore influence the age at which the condition first becomes apparent to the owner. Ophthalmoscopic fundus changes are usually advanced by the time owners have noticed vision problems (see Figures 13.16 and 13.19, and Table 13.6). Table 13.6 Characteristics of canine retinal photoreceptor degenerations. ERG, electroretinography. There is a variation in age of onset, rate of progression, and topographical changes in the retina between (and within) breeds that suffer from prcd. This variation is likely to eventually prove to be due, at least in part, to background genetics (presence of modifying loci). Some of these breeds include English Cocker Spaniel, American Cocker Spaniel, and Labrador Retriever. For an up‐to‐date and comprehensive list of other canine breeds affected by the prcd mutation, see www.optigen.com. These breeds include Tibetan Terrier, Tibetan Spaniel, Akita, Papillon, and English Springer Spaniel. The Schapendoes breed of dog has recently been shown to have autosomal recessive PRA caused by a mutation in a newly identified gene: coiled‐coil domain containing 66 (CCDC66). The Golden Retriever is also affected by the prcd mutation. A recent study indicated a second disease‐causing gene for PRA in Golden Retriever: a frameshift mutation in the SLC4A3 gene was shown to be implicated in the disease. A large proportion of the PRA cases still remain unexplained (44%), however, which indicates that PRA in the breed is genetically heterogeneous and that there are at least three different forms in the breed (mutations in PRCD, SLC4A3, and an as yet unidentified mutation). Hereditary retinal degeneration in Siberian Huskies has been described. There seemed to be an excess of affected male dogs with the disorder, and further studies, including test breeding schemes, showed there was an X‐linked transmission for the disease. Clinical signs of X‐linked retinal degeneration include initial night blindness and early ophthalmoscopic signs of the retinal degeneration, usually observed at two to four years of age. The same form of PRA was described in the Samoyed and has been shown to be due to a mutation in the open reading frame 15 of the RP GTPase regulator (RPGR) gene. The X‐linked PRA described in both the Siberian Husky and the Samoyed is termed X‐linked PRA type 1 (XLPRA1). A mutation‐based DNA test is available for XLPRA1. In the Old English Mastiff and in Bull Mastiff dogs, a specific retinal disease unlike those previously described was discovered. The disease displayed an ambiguous mode of inheritance and therefore outcross matings were performed in order to elucidate the specific mode of inheritance for the disorder, which was shown to follow a dominant pattern. The defect found is the first opsin mutation described for dogs. The gene defect is a T4R mutation (a point mutation that changes the coding at codon 4 from threonine to arginine), indicating a different cause of PRA than previously described in other breeds of dog. PRA has been described in more than 100 different canine breeds. The disease in all of these breeds has not been reported in detail in the scientific literature. Rubin provided a valuable summary on retinal degeneration/PRA in various breeds. Specific information about retinal diseases in the various breeds can also be obtained from the American College of Veterinary Ophthalmologists Genetic Committee (see latest edition of Hereditary Ocular Disease of Purebred Dogs) and from the ECVO Hereditary Eye Disease Committee. These breeds include Bernese Mountain dog; an early‐onset retinal degeneration has been described. The disease can be detected by ophthalmoscopy and behavioral studies at approximately one year of age. A recessively inherited disease has been described in Alaskan Malamutes that specifically affects the cones and causes congenital day blindness (originally described as hemeralopia). Clinically, behavioral signs in affected dogs are usually apparent at 8–10 weeks of age. The affected dog shows severe loss of vision in daylight and in high levels of artificial illumination, but it becomes less insecure when taken into dim lighting conditions. Recovery of vision takes several minutes, though loss of vision on returning to bright light is immediate. No funduscopic changes are observed at any stage of disease, and the PLRs are normal in both dim and bright light. The ERG of an affected dog reveals normal rod function but an absence of cone responses. In the day blind dog, the cone contribution to the ERG, including the response to rapidly flickering lights, is lost. Flicker fusion studies are particularly valuable in diagnosing day blindness: the cone branch of the bipartite flicker fusion response curve (20–70 Hz) is usually absent, whereas the rod branch (less than 20 Hz) resembles a normal response. Cone dystrophy has also been reported in the German Short‐Haired Pointer. Breeding studies showed that the disease in the German Short‐Haired Pointer is allelic with the Alaskan Malamute cone degeneration. A point mutation in CNGB3 was identified in German Short‐Haired Pointers. Gene therapy using an adeno‐associated viral vector to introduce a normal copy of the CNGB3 gene to affected Alaskan Malamutes and German Short‐Haired Pointers restored cone function. The clinical condition has also been described in the Miniature Poodle and three single cases: in a Rhodesian Ridgeback cross, an Australian Cattle Dog, and a Chihuahua. A condition was described in Belgian Shepherds as a form of photoreceptor dysplasia in which complete blindness was observed at eight weeks in the absence of ophthalmoscopic changes. By 11 weeks, a multifocal pattern of retinal folding or thickening was seen. The foci degenerated, and subsequently, there were hyperreflective lesions with a marked central pigmentation. The ERG was nonrecordable from at least four weeks of age. The term CPRA has been used for a group of conditions with ophthalmoscopic changes that can be characterized by an accumulation of irregular foci or light‐brown pigment spots in the central tapetal fundus (Figure 13.22a and b). Over time, these foci increase in size and become distributed throughout the tapetal zone. At this stage, there are also atrophic changes, such as hyperreflectivity around the pigment foci that indicate atrophy of the overlying neural retina. The nontapetal fundus shows similar foci, with hyperpigmentation and depigmented areas in between. It is the general opinion among veterinary ophthalmologists today that at least among those breeds in which the defect has been established microscopically, the term RPED is preferred. The disease has been recognized in Labrador and Golden Retrievers, Border Collies, Rough and Smooth Collies, Shetland Sheepdogs, English Cocker Spaniels, English Springer Spaniels, Chesapeake Bay Retrievers, and others. In Briards, an unusually high frequency of the disorder was reported: between 30% and 40%. In recent years, however, the condition is more rarely observed in the various breeds. It has been suggested that CPRA/RPED in Labrador Retriever is inherited as a dominant trait with variable penetrance. The reduction in incidence, which has been accomplished in Border Collies, through selective breeding is perhaps consistent with this view. A recessive mode of inheritance was thought to be likely in the Briard, but this has not been proved. Important in this context is that lesions similar to CPRA/RPED have been produced in dogs fed diets deficient in vitamin E, an antioxidant that retards the intracellular accumulation of lipofuscin pigment. Naturally acquired retinopathy resulting from vitamin E deficiency has also been described in the dog. The ERG is not diagnostic for CPRA, but there is a reduction in ERG amplitudes with disease progression. Secondary cataracts are often seen at the advanced stage. Figure 13.22 Advanced RPED with typical ophthalmoscopic changes. (a) A four‐year‐old English Cocker Spaniel with multiple light brown spots across the tapetal fundus. This dog has low circulating vitamin A levels. (b) A five‐year‐old English Cocker Spaniel with darkly pigmented spots surrounded by hyperreflective zones and severely attenuated retinal vessels. In Briards, an extremely high frequency of the disease was found in a five‐year survey. Almost one‐third of dogs examined, older than 18 months, were clinically affected. In this breed, the typical pigmentary changes were first seen toward the periphery of the tapetal fundus, temporal to the optic disc, before spreading to involve the entire tapetal zone. In the Briard dog, an interesting retinal dystrophy (with many names) has been described. The hereditary, clinical, and morphological characteristics of the disease were elucidated in groups of inbred and outcrossed dogs in Sweden. Initially, the disease was described as congenital stationary night blindness, since it affected Briards from birth; affected dogs had nonrecordable rod ERGs, and no funduscopic changes were observed until the dogs were two to three years old. Further clinical and morphological studies showed that there was a progressive component in the disorder. The neural retina and the RPE were both affected by a retinal degenerative disease with unusually slow progression. Clinically affected dogs were congenitally night blind, whereas daylight vision could vary from near normal to more or less severely impaired. A rapid, horizontal nystagmus was seen in most (but not all) affected pups, and it was obvious in some of the animals that they were congenitally day and night blind. The resting pupillary diameter was approximately twice as wide both in bright and in dim lighting conditions among affected dogs compared with the diameter in normal dogs. Ophthalmoscopically, the fundus appearance in dogs up to approximately three years of age was normal. With progression, a subtle alteration in tapetal sheen was seen in affected dogs, and in certain individuals, whitish gray spots were observed in the central and midperipheral nontapetal fundus, spreading peripherally with increasing age. Through candidate gene analysis, the defect in Briards in Sweden was discovered to be a 4‐bp deletion in the RPE65 gene. This gene defect resulted in a frameshift and a premature stop codon, which truncated the protein and resulted in no RPE65 formation in the RPE. Clinical signs of the defect in affected Briard dogs had close similarities to the human counterpart, Leber’s congenital amaurosis type II. In 2001, treating three dogs affected with the retinal dystrophy with a corrective gene construct, subretinal injections were performed using a recombinant adeno‐associated virus (AAV) carrying normal dog RPE65. Vision was improved in eyes treated subretinally as demonstrated by ERG recordings, and transmission of the retinal activity was shown by pupillometry. A novel disease has recently been described to cause retinal impairment and blindness in the Chinese Crested Dog (CCD). The disease is bilaterally symmetrical and has been observed in CCDs from northern Europe and the United States. Affected animals have been negative for the prcd mutation, which previously has been described (www.optigen.com; www.embarkvet.com) to be prevalent in the breed. Ophthalmoscopically, in three‐ to four‐year‐old affected dogs, darkly pigmented lesions, with a light center (doughnut‐like lesions) are seen in the peripheral fundus, most easily observed in the tapetal fundus, but prevalent also in the nontapetal area. The neuronal ceroid lipofuscinoses (NCL) are a group of recessively inherited progressive neurodegenerative diseases that are characterized by brain and retinal atrophy and associated with selective necrosis of neurons. The term ceroid lipofuscinosis is derived from the histochemical and fluorescent properties of accumulated pigment, which resembles those of the lipopigments ceroid and lipofuscin common to these diseases. They are also linked to brain atrophy, a defect not found to the same degree in other lysosomal storage diseases. These diseases affect both humans and several other animal species, such as bovine, ovine, caprine, feline, and canine. A spectrum of biochemical defects has been implicated in these disorders. Most forms reflect an accumulation of subunit c of mitochondrial ATP synthase, while in other forms there is sphingolipid activator protein storage in granular osmiophilic deposits, mainly in neuronal cells. The central nervous system (CNS) appears to be the main focus of the pathogenetic defect. NCLs have been described in several dog breeds, including the English Setter, Dalmatian, Border Collie, Tibetan Terrier, American Bulldog, Miniature Schnauzer, Polish Owczarek Nizinny, Longhaired Dachshund, and others (for reviews on the disease in various breeds, see www.caninegeneticdiseases.net). A common clinical manifestation in both humans and dogs is blindness, which occurs with a broad spectrum of neurological abnormalities caused by the accumulation of autofluorescent lipopigments in neurons and in some other cells. The mutation for NCL in the English Setter dog was elucidated: a mutation in the CLN8 gene was found to be responsible for the disorder. A group of American Bulldogs were revealed to have NCL and a defect in the gene for cathepsin D (CTSD) was elucidated; in the Border Collie dogs, there is a mutation in the CLN5 gene (see Appendix A). Recently, mutations in the CLN2 gene in the Longhaired Dachshund and a mutation in CLN6 in the Australian Shepherd have been identified as causes of NCL. A young Miniature Dachshund with neurological signs in keeping with NCL was found to have a mutation in the PPT1 gene. In both humans and animals, mucopolysaccharide storage diseases are caused by the inherited deficiency of lysosomal enzymes, and they represent generalized, multisystemic abnormalities, of which ocular lesions are but one component (see Appendix E). The lysosomal enzymes participate in degradation of glycosaminoglycans. Morphological studies have shown accumulation of intracellular inclusions in secondary lysosomes in the enzyme‐deficient RPE. Inflammatory lesions of the ocular fundus are not uncommon in the dog. Retinal involvement, however, is usually secondary to disease processes extending from the choroid or sometimes the vitreous, whereas primary retinal inflammation (i.e., retinitis) is uncommon. The terms chorioretinitis (i.e., starting in the choroid) and retinochoroiditis (i.e., initially involving the retina) indicate the primary site and direction of spread when both the choroid and retina are involved. There may also be concurrent involvement of the anterior uvea (also see Chapter 19) as in panuveitis (inflammation of the entire uveal tract), endophthalmitis (inflammation of the internal structures of the eye), and panophthalmitis (inflammation of the entire eyeball). The inflammatory process may accompany a systemic disease; a full physical examination is an essential part of the workup, and further investigations such as chest radiographs and abdominal ultrasound should be considered. In addition to routine laboratory investigations such as a complete blood count, serum chemistry, and urinalysis, serology for antibody titers to specific infectious agents may be indicated. Sensitive polymerase chain reaction (PCR) diagnostic tests for the presence of the DNA of pathogens in samples have been, and are being, developed. In certain instances, aspirates (e.g., from lymph nodes, aqueous humor, vitreous, and subretinal fluid) may be obtained and subjected to a variety of tests, including cytology, culture, and PCR amplification. Diagnostic testing should be fully utilized in an attempt to identify the etiology of the inflammation, particularly as the use of nonspecific anti‐inflammatory medications (such as systemic corticosteroids), although desired in idiopathic or immune‐mediated inflammation, may be contraindicated in certain infectious diseases. Chorioretinitis may have various causes, including several infectious diseases, neoplasia, foreign bodies, and trauma (Box 13.2 and Table 13.7). Infectious agents may be restricted to the eye, but signs in the ocular fundus may also be part of, or secondary to, a systemic infection. In many instances, the exact cause of the inflammatory process cannot be identified. The appearance of the lesions in the fundus may give some guidance as to the etiology, but this is seldom specific. Sampling from the choroid and retina presents certain difficulties and can be associated with undesired complications. Vitreous paracentesis to obtain material for cytology and culture can be informative, but is usually reserved for patients presenting with severe posterior segment inflammatory disease.
13
Diseases and Surgery of the Canine Posterior Segment
Section I: Diseases and Surgery of the Canine Vitreous
Development and Anatomy
Morphology
Diagnostic Procedures
Focal Light
Slit‐Lamp Biomicroscopy
Ophthalmoscopy
Diagnostic Imaging
Hyalocentesis
Therapeutic Procedures
Medical Treatment
Surgical Treatment
Vitrectomy
Anterior Vitrectomy
Pars Plana Posterior Vitrectomy
Vitreal Diseases
Developmental Disorders
Persistent Hyaloid Artery
Persistent Tunica Vasculosa Lentis
PHTVL/PHPV
Other Anomalies
Acquired Disorders
Trauma
Degenerative Vitreal Disorders and Reactions
Syneresis
Vitreous Floaters
Asteroid Hyalosis
Synchysis Scintillans
Intravitreal Membranes
Vitreal Inflammations
Cysts
Parasites
Neoplastic Disease
Vitreous in Relation to Other Ophthalmic Disorders
Lens Luxation
Retinal Detachment
Section II: Diseases of the Canine Ocular Fundus
Methods of Examination
Behavioral Testing
Testing Reflexes and Responses
Structural Visualization of the Fundus
Ophthalmoscopy
Scanning Laser Ophthalmoscopy
Optical Coherence Tomography
Adaptive Optics
Ultrasonography
Angiography
Functional Testing of the Retina
Electroretinography
Flash Electroretinography
Pattern ERG
Visually Evoked Potentials
Multifocal ERGs and VEPs
DNA‐Based Tests for Retinal Dystrophies
Normal Ocular Fundus
Tapetal Fundus
Nontapetal Fundus
Optic Nerve Head
Retinal Vasculature
Development of the Canine Ocular Fundus
Ophthalmoscopy
Functional and Morphological Development of the Retina
Developmental Anomalies
Collie Eye Anomaly
Clinical Findings
Difficulties in Diagnosis, Interpretation, and Control
Lesion
Appearance
Choroidal hypoplasia
Bilateral, pale‐to‐white area lateral (temporal) or dorsolateral (superotemporal) of the ONH, variable size; can be asymmetrical; difficult to detect in merle/subalbinoid dogs
Best to exam puppies at six to seven weeks of age
Can pigment and appear normal later (Go‐Normals)
Posterior colobomas
Involve the optic disc (i.e., papillary colobomas) or adjacent area (i.e., peripapillary colobomas)
Also called “pits” or posterior scleral ectasia
Appear as gray or pink indentations; vary from shallow depressions to more extensive excavations or “holes” up to 30 D in depth
May occur in 10–30% of eyes with choroidal hypoplasia
Retinal detachment
Partial or complete; uni‐ or bilateral; in 5–10% of CEA eyes
Appear clinically as nonfixation of the eyes, a tendency to nystagmus, or even strabismus in affected puppies
Affect both puppies and adults
Most arise adjacent to the optic disc and extend toward the peripheral fundus, often with subretinal, intraretinal, or preretinal hemorrhages
Intraocular hyphema
Occur in 5% or less of CEA eyes, often with colobomatous defects and RDs
Merle Ocular Dysgenesis
Retinal Dysplasia
Spontaneous Retinal Dysplasia
Clinical Signs
Type
Ophthalmoscopic findings
Focal or multifocal
Retinal folds and rosettes are seen as areas of reduced tapetal reflectivity, appearing as gray or green dots or linear, “V,” or “Y” streaks
Occur anywhere in the tapetal region, but most often centrally and usually above the optic disc
May appear elevated, distorting the course of the overlying vessels
Retinal folds in the nontapetal fundus appear as gray or white linear or irregular streaks
Geographic
Appear as an irregular or horseshoe‐shaped area, most often in the central tapetal fundus
May include focal retinal thinning and elevation
Usually, hyperreflective area with variable pigmentation
Complete with detachment
Usually, a completely detached neural retina floating in the vitreous, attached around the ONH
Breed (mode of inheritance)
Type of RD
Other abnormalities
Akita
Complete
Microphthalmia, cataract, posterior lenticonus
American Cocker Spaniel (AR)
Multifocal
None
Multifocal
Microphthalmia, cataracts, persistent pupillary membranes
Australian Shepherd
Complete
Iridal heterochromia, cataracts, staphylomas, RDs
(AR, incomplete penetrance)
(any breed with homozygous merling)
Beagle
Multifocal
Microphthalmia
Bedlington Terrier (AR)
Complete
Microphthalmia, cataracts, RDs
Cavalier King Charles Spaniel
Geographic
Microphthalmia, cataracts, posterior lenticonus
Chow Chow
Complete
Severe ocular defects
Doberman Pinscher (AR)
Complete
Microphthalmia, anterior segment dysplasia, aphakia, hyperplastic primary vitreous
English Springer Spaniel (AR)
Geographic
Microphthalmia, cataracts, RDs
Golden Retriever
Geographic
None
Labrador Retriever
Multifocal
None
Geographic
None
(AR)
Complete
Microphthalmia, cataracts, intraocular hemorrhage
(AD)
Complete
Cataracts; retarded growth of the radius, ulna, and tibia; separated hypoplastic anconeal and coronoid processes; hip dysplasia; delayed development of the epiphysis
Rottweiler
Multifocal
None
Sealyham Terrier (AR)
Complete
Microphthalmia, cataracts, RDs
Samoyed
Complete
Microphthalmia, cataracts, hyaloid artery persistence, and skeletal defects as in the Labrador Retriever
Yorkshire Terrier
Multifocal
None
Breed Incidence and Inheritance
Canine Oculoskeletal Dysplasia – Dwarfism with Retinal Dysplasia
Inherited Retinal Dysplasia and Persistent Hyperplastic Primary Vitreous
Induced Retinal Dysplasia
Difficulties in Diagnosis, Interpretation, and Control
Canine Multifocal Retinopathy
Inherited Retinal Degenerations/Dystrophies
Classification of Canine Hereditary Retinal Degenerations
Clinical Signs of Hereditary Retinal Degeneration/Progressive Retinal Atrophy
Stage of the disease
Ophthalmoscopic changes
Early
Slight change in tapetal reflectivity or hyporeflectivity is often seen, such as a grayish discoloration mainly in the peripheral tapetal fundus
A slight vascular attenuation and beading of retinal vessels in the midperipheral and peripheral parts of the tapetal fundus may be observed
Moderate
Color changes in the tapetal fundus become more marked and generalized, as does the vascular attenuation
Increased reflectivity, or hyperreflectivity, of the tapetal fundus, which is usually most marked in the midperipheral tapetal fundus
Slight decrease in the pigmentation of the nontapetal fundus
ONH changes include early loss of myelin, disc changes in shape to circular, and reduced number and calipers of blood vessels
Early optic disc atrophy
Advanced
Hyperreflectivity progresses to involve the entire tapetal fundus
Continued loss of the pigmentation of the nontapetal fundus
Marked decrease in the retinal vasculature with preservation of only the large and central blood vessels until late in the disease
Ghost vessels peripherally
Optic disc pallor, loss of myelin, and reduction in disc size
Early‐Onset Photoreceptor Degenerations
Rod–Cone Dysplasia Type 2
Breed
Primary defect
Mode of inheritance
Diagnosis by ophthalmoscopy
Diagnosis by ERG
Alaskan Malamute
Cone degeneration
AR
No changes
6 weeks: cone –; rod normal
Belgian Shepherd
Rod–cone dysplasia
Unknown
11 weeks
4 weeks: cone –; rod –
Collie
Rod–cone dysplasia
AR
16 weeks
6 weeks: cone ↓; rod –
Irish Setter
Rod–cone dysplasia
AR
16 weeks
6 weeks: cone ↓; rod –
Miniature Schnauzer
Rod–cone dysplasia
AR
1.5–5 years
6 weeks; cone ↓; rod ↓
Norwegian Elkhound
Rod dysplasia
AR
1–1.5 years
6 weeks: cone normal/↓; rod –
Norwegian Elkhound
Early rod degeneration
AR
9 months to 1 year
5 weeks: A‐wave dominates. Cone ↓; rod ↓
Pit Bull Terrier
Cone–rod dystrophy
Unknown
3–6 months
6 weeks: cone –; rod ↓
Short‐Haired Dachshund
Cone–rod dystrophy
AR suspected
3 years
5 weeks: cone –; rod ↓
Long‐Haired Dachshund
Cone–rod dystrophy
Unknown
6 months
6 weeks: cone ↓; rod normal
Rod–Cone Dysplasia Type 3
Rod Dysplasia
Early Retinal Degeneration
Photoreceptor Dysplasia
Cone–Rod Dystrophy
Late‐Onset PhotoreceptorDegenerations
Progressive Rod–Cone Degeneration
prcd in the Miniature and Toy Poodle
Breed
Primary defect
Mode of inheritance
Diagnosis by ophthalmoscopy
Diagnosis by ERG andchanges
Akita
Not established
AR
1–3 years
1.5–2 years: cone ↓; rod ↓
American Cocker Spaniel
Rod–cone degeneration
AR
2.5–3 years
9 months: cone ↓; rod ↓
English Cocker Spaniel
Rod–cone degeneration
AR
3–8 years
2–3 years: cone ↓; rod ↓
Labrador Retriever
Rod–cone degeneration
AR
3–6 years
1.5 years: cone ↓; rod ↓
Miniature Long‐haired
Unknown
AR
6 months
4 months: cone ↓; rod ↓
Dachshund
Papillon
Unknown
AR
1.5–5 years
9 months to 1.5 years: cone ↓; rod ↓
Portuguese Water Dog
Rod–cone degeneration
AR
3–6 years
1.5 years: cone ↓; rod ↓
Siberian Husky
Unknown
X‐linked
2 years
1 year: cone ↓; rod ↓
Tibetan Spaniel
Unknown
AR
3–5 years
1.5 years: cone ↓; rod ↓
Tibetan Terrier
Unknown
AR
1.0–1.5 years
10 months: cone ↓; rod ↓
Toy and Miniature Poodle
Rod–cone degeneration
AR
3–5 years
9 months: cone ↓; rod ↓
prcd in Other Breeds
Autosomal Recessive PRA (Non‐prcd) in Other Breeds
X‐Linked Progressive Retinal Atrophy
Dominant Progressive Retinal Atrophy
PRA in Other Breeds Not Yet Characterized
Other Generalized Retinopathies/Retinal Dystrophies
Early Retinopathy
Cone Degeneration
Photoreceptor Dysplasia
RPE Autofluorescent Inclusion Epitheliopathy/Retinal Pigment Epithelial Dystrophy
Hereditary Retinal Dystrophy/Lipid Retinopathy/RPE65 Null Mutation/Canine Leber’s Congenital Amaurosis
Pigmentary Chorioretinopathy
Neuronal Ceroid Lipofuscinosis
Mucopolysaccharide Storage Diseases
Inflammation and Infections Affecting the Ocular Fundus
Chorioretinitis
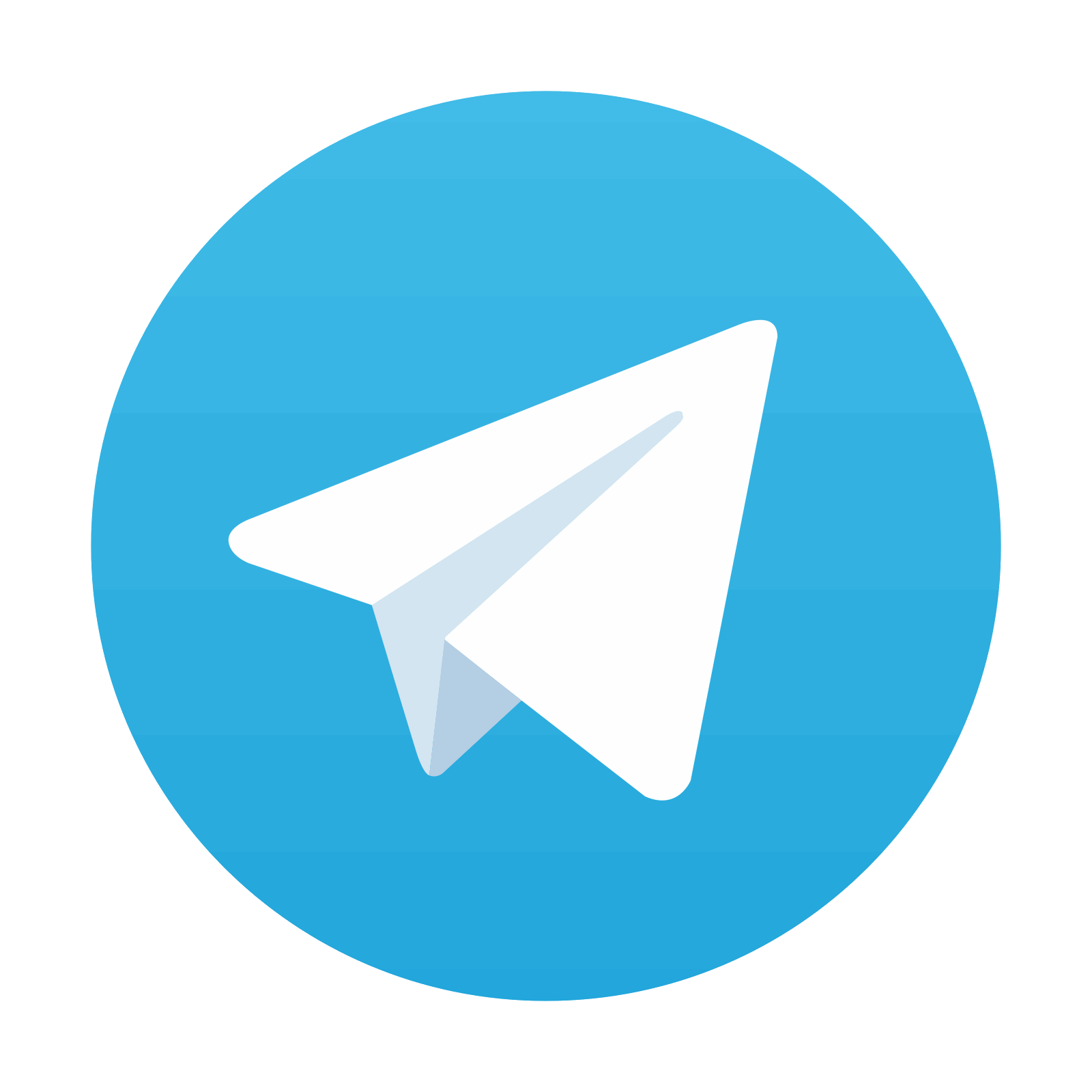
Stay updated, free articles. Join our Telegram channel

Full access? Get Clinical Tree
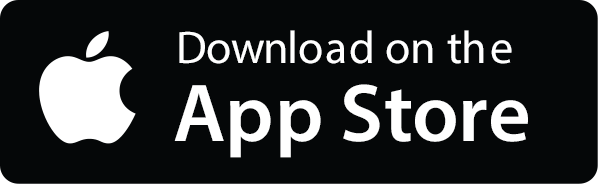
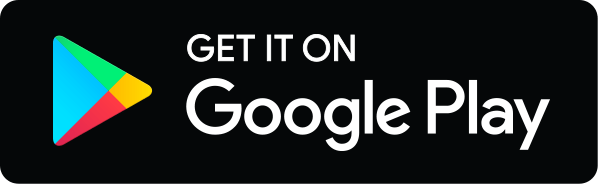