Ocular development has been investigated in some detail in rodents, the dog, and the cow, and demonstrates that the sequence of developmental events is very similar across species. When comparing these studies, one should consider differences in duration of gestation, differences in anatomical end point (e.g., presence of a tapetum, macula, or Schlemm’s canal), and when eyelid fusion breaks (during the sixth month of gestation in the human versus two weeks postnatal in the dog and cow) (Tables 1.1 and 1.2). Cellular mitosis following fertilization results in transformation of the single‐cell zygote into a cluster of 12–16 cells. With continued cellular proliferation, this morula becomes a blastocyst, containing a fluid‐filled cavity. The cells of the blastocyst will form both the embryo proper and the extraembryonic tissues (i.e., amnion and chorion). At this early stage, the embryo is a bilaminar disc, consisting of hypoblast and epiblast. This embryonic tissue divides the blastocyst space into the amniotic cavity (adjacent to epiblast) and the yolk sac (adjacent to hypoblast). Gastrulation (formation of the mesodermal germ layer) begins during day 10 of gestation in the dog (day 7 in the mouse; days 15–20 in the human). The primitive streak forms as a longitudinal groove within the epiblast (i.e., future ectoderm). Epiblast cells migrate toward the primitive streak, where they invaginate to form the mesoderm. This forms the three classic germ layers: ectoderm, mesoderm, and endoderm. Gastrulation proceeds in a cranial‐to‐caudal progression; simultaneously, the cranial surface ectoderm proliferates, forming bilateral elevations called the neural folds (i.e., future brain). The columnar surface ectoderm in this area now becomes known as the neural ectoderm. As the neural folds elevate and approach each other, a specialized population of mesenchymal cells, the neural crest, emigrates from the neural ectoderm at its junction with the surface ectoderm. Migration and differentiation of the neural crest cells are influenced by the hyaluronic acid‐rich extracellular matrix. This acellular matrix is secreted by the surface epithelium as well as by the crest cells, and it forms a space through which the crest cells migrate. The neural crest cells migrate peripherally beneath the surface ectoderm to spread throughout the embryo, populating the region around the optic vesicle and ultimately giving rise to nearly all the connective tissue structures of the eye (Table 1.3). Table 1.1 Sequence of ocular development in human, mouse, and dog. Table 1.2 Sequence of ocular development in the cow. Table 1.3 Embryonic origins of ocular tissues. It is important to note that mesenchyme is a general term for any embryonic connective tissue. Mesenchymal cells generally appear stellate and are actively migrating populations with extensive extracellular space. In contrast, the term mesoderm refers specifically to the middle embryonic germ layer. In the eye, mesoderm probably gives rise only to the striated myocytes of the extraocular muscles (EOMs) and vascular endothelium. Most of the craniofacial mesenchymal tissue comes from neural crest cell. The optic sulci are visible as paired evaginations of the forebrain neural ectoderm on day 13 of gestation in the dog (Figure 1.1). The transformation from optic sulcus to optic vesicle is considered to occur concurrent with the closure of the neural tube (day 15 in the dog). Figure 1.1 Development of the optic sulci, which are the first sign of eye development. Optic sulci on the inside of the forebrain vesicles consisting of neural ectoderm (shaded cells). The optic sulci evaginate toward the surface ectoderm as the forebrain vesicles simultaneously rotate inward to fuse. The optic vesicle enlarges and, covered by its own basal lamina, approaches the basal lamina underlying the surface ectoderm. The optic vesicle appears to play a significant role in the induction and size determination of the palpebral fissure and of the orbital and periocular structure. An external bulge indicating the presence of the enlarging optic vesicle can be seen at approximately day 17 in the dog. The optic vesicle and optic stalk invaginate through differential growth and infolding. Local apical contraction and physiological cell death have been identified during invagination. The surface ectoderm in contact with the optic vesicle thickens to form the lens placode, which then invaginates with the underlying neural ectoderm. The invaginating neural ectoderm folds onto itself as the space within the optic vesicle collapses, thus creating a double layer of neural ectoderm, the optic cup. This process of optic vesicle/lens placode invagination progresses from inferior to superior, so the sides of the optic cup and stalk meet inferiorly in an area called the optic (choroid/retinal) fissure. Mesenchymal tissue (of primarily neural crest origin) surrounds and fills the optic cup, and by day 25 in the dog, the hyaloid artery develops from mesenchyme in the optic fissure. This artery courses from the optic stalk (i.e., the region of the future optic nerve) to the developing lens. The two edges of the optic fissure meet and initially fuse anterior to the optic stalk, with fusion then progressing anteriorly and posteriorly. This process is mediated by glycosaminoglycan (GAG)‐induced adhesion between the two edges of the fissure. Apoptosis has been identified in the inferior optic cup prior to formation of the optic fissure and, transiently, associated with its closure. Failure of this fissure to close normally may result in inferiorly located defects (i.e., colobomas) in the iris, choroid, or optic nerve. Colobomas other than those in the “typical” six‐o’clock location may occur through a different mechanism and are discussed later. Closure of the optic cup through fusion of the optic fissure allows intraocular pressure (IOP) to be established. Before contact with the optic vesicle, the surface ectoderm first becomes competent to respond to lens inducers. Inductive signals from the anterior neural plate give this area of ectoderm a “lens‐forming bias.” Signals from the optic vesicle are required for complete lens differentiation, and inhibitory signals from the cranial neural crest may suppress any residual lens‐forming bias in head ectoderm adjacent to the lens. Adhesion between the optic vesicle and surface ectoderm exists, but there is no direct cell contact. The basement membranes of the optic vesicle and the surface ectoderm remain separate and intact throughout the contact period. Thickening of the lens placode can be seen on day 17 in the dog. A tight, extracellular matrix‐mediated adhesion between the optic vesicle and the surface ectoderm has been described. This anchoring effect on the mitotically active ectoderm results in cell crowding and elongation and in formation of a thickened placode. This adhesion between the optic vesicle and lens placode also assures alignment of the lens and retina in the visual axis. The lens placode invaginates, forming a hollow sphere, now referred to as a lens vesicle (Figures 1.2 and 1.3). The size of the lens vesicle is determined by the contact area of the optic vesicle with the surface ectoderm and by the ability of the latter tissue to respond to induction. Aplasia may result from failure of lens induction or through later involutions of the lens vesicle, either before or after separation from the surface ectoderm. Figure 1.2 Formation of the lens vesicle and optic cup. Note that the optic fissure is present, because the optic cup is not yet fused inferiorly. (a) Formation of lens vesicle and optic cup with inferior choroidal or optic fissure. Mesenchyme (M) surrounds the invaginating lens vesicle. (b) Surface ectoderm forms the lens vesicle with a hollow interior. Note that the optic cup and optic stalk are of surface ectoderm origin. Lens vesicle detachment is the initial event leading to formation of the chambers of the ocular anterior segment. This process is accompanied by active migration of epithelial cells out of the keratolenticular stalk, cellular necrosis, apoptosis, and basement membrane breakdown. Induction of a small lens vesicle that fails to undergo normal separation from the surface ectoderm is one of the characteristics of the teratogen‐induced anterior segment dysgenesis described in animal models. Following detachment from the surface ectoderm (day 25 in the dog), the lens vesicle is lined by a monolayer of cuboidal cells surrounded by a basal lamina, the future lens capsule. The primitive retina promotes primary lens fiber formation in the adjacent lens epithelial cells. Thus, while the retina develops independently of the lens, the lens appears to be dependent on the retinal primordium for its differentiation. The primitive lens filled with primary lens fibers is the embryonic lens nucleus. In the adult, the embryonic nucleus is the central sphere inside the “Y” sutures; there are no sutures within the embryonal nucleus. Figure 1.3 Cross section through optic cup and optic fissure. The lens vesicle is separated from the surface ectoderm. Mesenchyme (M) surrounds the developing lens vesicle, and the hyaloid artery is seen within the optic fissure. At birth, the lens consists almost entirely of lens nucleus, with minimal lens cortex. Lens cortex continues to develop from the anterior cuboidal epithelial cells, which remain mitotic throughout life. Differentiation of epithelial cells into secondary lens fibers occurs at the lens equator (i.e., lens bow). Lens fiber elongation is accompanied by a corresponding increase in cell volume and a decrease in intercellular space within the lens. The zonule fibers are termed the tertiary vitreous, but their origin remains uncertain. The zonules may form from the developing ciliary epithelium or the endothelium of the posterior tunica vasculosa lentis (TVL). The hyaloid artery is the termination of the primitive ophthalmic artery, a branch of the internal ophthalmic artery, and it remains within the optic cup following closure of the optic fissure. The hyaloid artery branches around the posterior lens capsule and continues anteriorly to anastomose with the network of vessels in the pupillary membrane (Figure 1.4). The pupillary membrane consists of vessels and mesenchyme overlying the anterior lens capsule. This hyaloid vascular network that forms around the lens is called the anterior and posterior TVL. The hyaloid artery and associated TVL provide nutrition to the lens and anterior segment during its period of rapid differentiation. Venous drainage occurs via a network near the equatorial lens, in the area where the ciliary body will eventually develop. There is no discrete hyaloid vein. Once the ciliary body begins actively producing aqueous humor, which circulates and nourishes the lens, the hyaloid system is no longer needed. The hyaloid vasculature and TVL reach their maximal development by day 45 in the dog and then begin to regress. As the peripheral hyaloid vasculature regresses, the retinal vessels develop. Spindle‐shaped mesenchymal cells from the wall of the hyaloid artery at the optic disc form buds (angiogenesis) that invade the nerve fiber layer. Branches of the hyaloid artery become sporadically occluded by macrophages prior to their gradual atrophy. Placental growth factor and vascular endothelial growth factor appear to be involved in hyaloid regression. Proximal arteriolar vasoconstriction at birth precedes regression of the major hyaloid vasculature. Atrophy of the pupillary membrane, TVL, and hyaloid artery occurs initially through apoptosis and later through cellular necrosis, and is usually complete by the time of eyelid opening 14 days postnatally. Figure 1.4 The hyaloid vascular system and TVL. The clinical lens anomaly known as Mittendorf’s dot is a small (1 mm) area of fibrosis on the posterior lens capsule, and it is a manifestation of incomplete regression of the hyaloid artery where it was attached to the posterior lens capsule. Bergmeister’s papilla represents a remnant of the hyaloid vasculature consisting of a small, fibrous glial tuft of tissue emanating from the center of the optic nerve. Both are frequently observed as incidental clinical findings. The anterior margins of the optic cup advance beneath the surface ectoderm and adjacent neural crest mesenchyme after lens vesicle detachment (day 25 in the dog). The surface ectoderm overlying the optic cup (i.e., the presumptive corneal epithelium) secretes a thick matrix, the primary stroma. Mesenchymal neural crest cells migrate between the surface ectoderm and the optic cup, using the basal lamina of the lens vesicle as a substrate. This loosely arranged mesenchyme fills the future anterior chamber and gives rise to the corneal endothelium and stroma, anterior iris stroma, ciliary muscle, and most structures of the iridocorneal angle (ICA). The presence of an adjacent lens vesicle is required for induction of corneal endothelium, identified by their production of the cell adhesion molecule, N‐cadherin. Patches of endothelium become confluent and develop zonulae occludentes during days 30–35 in the dog, and during this period, Descemet’s membrane also forms. Neural crest migration anterior to the lens forms the corneal stroma and iris stroma also results in formation of a solid sheet of mesenchymal tissue, which ultimately remodels to form the anterior chamber. The portion of this sheet that bridges the future pupil is called the pupillary membrane. Vessels within the pupillary membrane form the TVL, which surrounds and nourishes the lens. These vessels are continuous with those of the primary vitreous (i.e., hyaloid). The vascular endothelium is the only intraocular tissue of mesodermal origin; even the vascular smooth muscle cells and pericytes, which originate from mesoderm in the rest of the body, are of neural crest origin. In the dog, atrophy of the pupillary membrane begins by day 45 of gestation and continues during the first two postnatal weeks. Separation of the corneal mesenchyme (neural crest cell origin) from the lens (surface ectoderm origin) results in formation of the anterior chamber. The two layers of the optic cup (neuroectoderm origin) consist of an inner, nonpigmented layer and an outer, pigmented layer. Both the pigmented and nonpigmented epithelia of the iris and the ciliary body develop from the anterior aspect of the optic cup; the retina develops from the posterior optic cup. The optic vesicle is organized with all cell apices directed to the center of the vesicle. During optic cup invagination, the apices of the inner and outer epithelial layers become adjacent. Thus, the cells of the optic cup are oriented apex to apex. A thin, periodic acid–Schiff (PAS)‐positive basal lamina lines the inner aspect (i.e., vitreous side) of the nonpigmented epithelium and retina (i.e., inner limiting membrane). By approximately day 40 of gestation in the dog, both the pigmented and nonpigmented epithelial cells show apical cilia that project into the intercellular space. These changes probably represent the first production of aqueous humor. The iris stroma develops from the anterior segment mesenchymal tissue (neural crest cell origin), and the iris pigmented and nonpigmented epithelia originate from the neural ectoderm of the optic cup. The smooth muscle of the pupillary sphincter and dilator muscles ultimately differentiate from these epithelial layers, and they represent the only mammalian muscles of neural ectodermal origin. In avian species, however, the skeletal muscle cells in the iris are of neural crest origin, with a possible small contribution of mesoderm to the ventral portion. Differential growth of the optic cup epithelial layers results in folding of the inner layer, representing early, anterior ciliary processes. The ciliary body epithelium develops from the neuroectoderm of the anterior optic cup, and the underlying mesenchyme differentiates into the ciliary muscles. Extracellular matrix secreted by the ciliary epithelium becomes the tertiary vitreous and, ultimately, develops into lens zonules. The three phases of iridocorneal angle (ICA) maturation include (i) the separation of anterior mesenchyme into corneoscleral and iridociliary regions (i.e., trabecular primordium formation), followed by differentiation of ciliary muscle and folding of the neural ectoderm into ciliary processes; (ii) the enlargement of the corneal trabeculae and development of clefts in the area of the trabecular meshwork; and (iii) the postnatal remodeling of the drainage angle, associated with cellular necrosis and phagocytosis by macrophages, resulting in opening of clefts in the trabecular meshwork and outflow pathways. In species born with congenitally fused eyelids (i.e., dog and cat), development of the anterior chamber continues during this postnatal period before eyelid opening. At birth, the peripheral iris and cornea are in contact with maturation of pectinate ligaments by three weeks and rarefaction of the uveal and corneoscleral trabecular meshworks to their adult state during the first eight weeks after birth. Infolding of the neuroectodermal optic vesicle results in a bilayered optic cup with the apices of these two cell layers in direct contact. Primitive optic vesicle cells are columnar, but by 20 days of gestation in the dog, they form a cuboidal layer containing the first melanin granules in the developing embryo. The neurosensory retina develops from the inner nonpigmented layer of the optic cup and the retinal pigment epithelium (RPE) originates from the outer, pigmented layer. Bruch’s membrane (the basal lamina of the RPE) is first seen during this time, and becomes well developed over the next week, when the choriocapillaris is developing. By day 45, the RPE cells take on a hexagonal cross‐sectional shape and develop microvilli that interdigitate with projections from photoreceptors of the nonpigmented (inner) layer of the optic cup. At the time of lens placode induction, the retinal primordium consists of an outer, nuclear zone and an inner, marginal (anuclear) zone. This process forms the inner and outer neuroblastic layers, separated by their cell processes that make up the transient fiber layer of Chievitz. Cellular differentiation progresses from inner to outer layers and, regionally, from central to peripheral locations. Peripheral retinal differentiation may lag behind that occurring in the central retina by three to eight days in the dog. Retinal ganglion cells develop first within the inner neuroblastic layer, and axons of the ganglion cells collectively form the optic nerve. Cell bodies of the Müller and amacrine cells differentiate in the inner portion of the outer neuroblastic layer. Horizontal cells are found in the middle of this layer; the bipolar cells and photoreceptors mature last, in the outermost zone of the retina. Significant retinal differentiation continues postnatally, particularly in species born with fused eyelids. At birth, the canine retina has reached a stage of development equivalent to the human at three to four months of gestation. In the kitten, all ganglion cells and central retinal cells are present at birth with continued proliferation in the peripheral retina continuing during the first two to three postnatal weeks in dogs and cats. These neural crest‐derived tissues are all induced by the outer layer of the optic cup (future RPE). Normal RPE differentiation is a prerequisite for normal development of the sclera and choroid. The choroid and sclera are relatively differentiated at birth, but the tapetum in dogs and cats continues to develop and mature during the first four months postnatally. The primary vitreous forms posteriorly, between the primitive lens and the inner layer of the optic cup. In addition to the vessels of the hyaloid system, the primary vitreous also contains mesenchymal cells, collagenous fibrillar material, and macrophages. Primitive hyalocytes produce collagen fibrils that expand the volume of the secondary vitreous. The tertiary vitreous forms as a thick accumulation of collagen fibers between the lens equator and the optic cup. These fibers are called the marginal bundle of Drualt, or Drualt’s bundle. Drualt’s bundle has a strong attachment to the inner layer of the optic cup, and it is the precursor to the vitreous base and lens zonules. The early lens zonular fibers appear to be continuous with the inner, limiting membrane of the nonpigmented epithelial layer covering the ciliary muscle. Atrophy of the primary vitreous and hyaloid leaves a clear, narrow central zone, which is called Cloquet’s canal. Axons from the developing ganglion cells pass through vacuolated cells from the inner wall of the optic stalk. A glial sheath forms around the hyaloid artery. As the hyaloid artery regresses, the space between the hyaloid artery and the glial sheath enlarges. Bergmeister’s papilla represents a remnant of these glial cells around the hyaloid artery. Glial cells migrate into the optic nerve and form the primitive optic disc. The glial cells around the optic nerve and the glial part of the lamina cribrosa come from the inner layer of the optic stalk, which is of neural ectoderm origin. Later, a mesenchymal (neural crest origin) portion of the lamina cribrosa develops. Myelinization of the optic nerve begins at the chiasm, progresses toward the eye, and reaches the optic disc after birth. The eyelids develop from surface ectoderm, which gives rise to the epidermis, cilia, and conjunctival epithelium. Neural crest mesenchyme gives rise to deeper structures, including the dermis and tarsus. The eyelid muscles (i.e., orbicularis and levator) are derived from craniofacial condensations of mesoderm called somitomeres. The upper eyelid develops from the frontonasal process; the lower eyelid develops from the maxillary process. The lid folds grow together and elongate to cover the developing eye. The upper and lower lids fuse on day 32 of gestation in the dog. Separation occurs two weeks postnatally. The extraocular muscles (EOM) arise from mesoderm in somitomeres (i.e., preoptic mesodermal condensations). Spatial organization of developing eye muscles is initiated before they interact with the neural crest mesenchyme. From studies of chick embryos, it has been shown that the oculomotor‐innervated muscles originate from the first and second somitomeres, the superior oblique muscle from the third somitomere, and the lateral rectus muscle from the fourth somitomere. The entire length of these muscles appears to develop spontaneously rather than from the orbital apex anteriorly. A thorough understanding of normal ophthalmic anatomy is an integral part of the foundational knowledge for diagnosis and treatment of ophthalmic diseases as the majority of the ocular tissues can be visualized directly. The veterinarian can examine eyes from a wide variety of animal species, and fortunately the eye has largely retained the same basic components, but important and clinically relevant differences do exist. This chapter will primarily present the ophthalmic anatomy of dogs, cats, horses, livestock species, and occasional birds, and relates information important to the clinician. More detailed anatomical information is available in the standard veterinary and comparative anatomy texts. The orbit is the bony fossa that surrounds and protects the eye while separating it from the cranial cavity. Through numerous foramina, the orbit also provides pathways for various blood vessels and nerves involved in the function of the eye and nearby structures. The size, shape, and position of the orbit differ by species and are closely associated with time of visual activity and feeding behavior (Table 1.5). In domestic carnivores such as the cat and dog, the orbital axes are set rostrolaterally, approximately 10° and 20° from midline, respectively, to enhance binocular vision and predatory feeding behavior. In horses and ruminants, the orbits are positioned more laterally than carnivores, being approximately 40° (i.e., horses) and 50° (i.e., cattle) from midline. Monocular vision in these and other ungulate species is enhanced, providing a strong panoramic line of vision, which allows for scanning the horizon to search for potential predators. In the rabbit, the axis of each eye extends as much as 85° from the midline; this orbit placement also occurs among the majority of lizards, some snakes, and in certain fish. In these latter instances where binocular vision has become greatly reduced, there is a tendency for the eyes to protrude so that the visual axis of the eye can expand what the optic axis of the skull provides. All vertebrate orbits are one of two kinds: (i) the enclosed orbit, which is completely encompassed by bone; or (ii) the open or incomplete orbit, which is only partially surrounded by bone (Figure 1.5a and b). Among domestic animals, horses, sheep, cattle, and goats have enclosed orbits. Pigs and carnivores (i.e., dogs and cats) have open orbits. The enclosed orbit of large herbivorous prey species is theorized to be essential for protection (and sometimes horns), whereas the open orbit gives carnivores the ability to open their jaws widely during consumption of prey. The bony orbit typically consists of five to seven bones, depending on the species (see Table 1.4). The canine orbit is composed of five, and sometimes six, bones, the supraorbital ligament that extends from the frontal to the zygomatic bone, and the periosteum (Figure 1.5a). The orbital rim is formed by the frontal, lacrimal, and zygomatic bones. Laterally, the orbit is formed by the supraorbital ligament that is contiguous with a fibroelastic connective tissue sheath for much of the floor of the orbit. The orbital floor is incomplete, being partially formed by the sphenoid and palatine bones. Therefore, surgical entry into the deeper orbit is from the dorsal but primarily the lateral wall. Table 1.4 Orbital dimensions. In the feline orbit, the processes of the frontal and zygomatic bones extend a great deal more toward one another, resulting in a shortened supraorbital ligament (Figure 1.5b). There is limited orbital space in cats. In animals with enclosed orbits, closure of the temporal side of the orbit is accomplished by union of the zygomatic process of the frontal bone with the frontal process of the zygomatic bone. In the horse, the zygomatic process of the temporal bone intervenes between these two and completes the orbital rim (Figure 1.6). Within the orbit, various foramina and fissures provide osseous pathways for blood vessels and nerves to pass from the cranial cavity and alar canal into the orbital region (Table 1.5). Those foramina of rather constant position in domestic animals are the rostral alar, ethmoidal, lacrimal, orbital, ovale, optic, rotundum, and supraorbital. Other foramina closely related to the orbital structures are within the pterygopalatine region, and these are the maxillary, caudal palatine, and sphenopalatine. The orbital foramen is elongated in most domestic animals, except the horse; therefore, it is referred to as the orbital fissure. In cattle, the orbital fissure and foramen rotundum are typically fused to form the foramen orbitorotundum. The orbital fascia consists of a thin, tough connective tissue lining that envelops all the structures within the orbit, including the bony fossa itself. This fascia consists of three anatomical components: the periorbita, Tenon’s capsule or fascia bulbi, and the EOM fascial sheaths (Figure 1.7). Orbital surgery is usually confined within these fascial tissues or beneath it. Figure 1.5 (a) Canine orbit. (b) Feline orbit. Bones of the orbit: frontal (F), lacrimal (L), maxilla (M), sphenoid (S), temporal (T), and zygomatic (Z). Orbital foramina: rostral alar (A), ethmoidal (E), optic (Op), and orbital fissure (Or). Figure 1.6 Equine orbit. Bones of the orbit: frontal (F), lacrimal (L), sphenoid (S), temporal (T), and zygomatic (Z). Orbital foramina: rostral alar (A), ethmoidal (E), optic (Op), orbital fissure (Or), and supraorbital (So). The periorbita is a conically shaped, fibrous membrane that lines the orbit and encloses the globe, EOMs, blood vessels, and nerves. The apex of the periorbita is located where the optic nerve exits the orbit and continuous with the dural sheath of the optic nerve. In the orbit, it is thin, attaches firmly to the orbital bones, and forms their periosteum. In the dog, the periorbita does not always fuse with the periosteum of the frontal and the sphenoid bones. In animals with an incomplete lateral orbital wall, the periorbita is thicker laterally next to the orbital ligament. Anteriorly, in the dorsolateral part of the orbit, the periorbita separates and surrounds the lacrimal gland. At the orbital rim, it divides into one part becoming continuous with the periosteum of the facial bones and the other, that is, the septum orbitale, merging with the eyelids and becoming continuous with the tarsal plates (the fibrous sheet in the eyelids). Within the periorbital tissue of carnivores (dogs and cats), smooth muscle has been observed along the lateral wall of the orbit, portions of the roof and floor of the orbit, and next to the periosteal lining of orbital bones, and contraction of the muscle has been produced by stimulation of the cervical sympathetic nerve trunk and results in forward movement of the globe. Table 1.5 Foramina and associated nerves and blood vessels. Figure 1.7 Divisions of orbital fascia: muscle fascia, periorbita, orbital septum, and Tenon’s capsule. Tenon’s capsule (fascia bulbi) is connective tissue on the outer aspect of the sclera. Tenon’s capsule is separated from the sclera by a narrow, cleft‐like space filled with loose connective tissue, Tenon’s space. Tenon’s capsule is attached to the sclera near the corneoscleral junction (i.e., limbus), and it becomes continuous with the fascia surrounding the EOMs. The fascial sheaths of the EOMs are dense, fibrous membranes loosely attached to the muscles with fine trabeculae of connective tissue. These sheaths are continuous with, or reflections of, Tenon’s capsule, but they are not always considered part of it. Three sheets of orbital fascia are separated by orbital fat. Orbital fat fills the dead space in the orbit and acts as a protective cushion for the eye. The amount of orbital fat varies between individuals and to a greater extent between species. The color of orbital fat ranges from white to yellow. Some animals, including birds and many reptiles, have very little orbital fat. When the retractor oculi muscle contracts, orbital fat can displace the glandular tissue associated with the nictitating membrane (NM), resulting in its passive movement over the cornea. The EOMs suspend the globe in the orbit and provide ocular motility (Table 1.6). There are four rectus muscles: the dorsal, ventral, medial, and lateral recti. They originate from the orbital apex (i.e., annulus of Zinn) and insert, in the dog, approximately 5 mm posterior to the limbus medially, 6 mm ventrally, 7 mm dorsally, and 9 mm laterally (Figures 1.8 and 1.9). They move the eye in the direction of their names. The dorsal (superior) oblique originates from the medial orbital apex, continuing forward dorsomedially to pass through a trochlea located near the medial canthus and pulls the dorsal aspect of the globe medially and ventrally (intorsion). The ventral (inferior) oblique originates from the anterolateral margin of the palatine bone on the medial orbital wall and passes beneath the eye, crossing the ventral rectus tendon. The muscle divides as it reaches the lateral rectus, with the anterior portion covering the insertion of the lateral rectus and the posterior portion inserting beneath the rectus. The ventral oblique moves the globe medially and dorsally (extorsion). Table 1.6 Muscles of the eye and eyelids. The retractor oculi (retractor bulbi) muscle originates at the orbital apex and continues forward to form a cone surrounding the optic nerve, and inserting posterior and deep to the recti muscles. The retractor oculi muscle retracts the globe into the orbit. The retractor oculi muscle is ubiquitous among mammals, but it is absent in various nonmammalian groups, including birds and snakes. The dorsal, ventral, and medial recti as well as the ventral oblique muscles are innervated by the oculomotor nerve (CN III), whereas the lateral rectus and retractor oculi muscles are innervated by the abducens nerve (CN VI), and the dorsal oblique muscle is innervated by the trochlear nerve (CN IV). Figure 1.8 Arrangement of the orbital muscles of domestic animals. Annulus of Zinn, ventral oblique muscle, ventral rectus muscle, lateral rectus muscle, retractor bulbi muscle tendon attachments, medial rectus muscle, dorsal oblique muscle, and dorsal rectus muscle. Figure 1.9 Orbital apex of the dog, illustrating structures passing through the optic foramen and orbital fissure as well as the EOM attachments. The eyelids, or palpebrae, are thin folds of skin continuous with the facial skin (Figures 1.10 and 1.11). The upper (superior) and lower (inferior) eyelids meet to form the lateral and medial canthi (singular canthus). The opening formed by the upper and lower eyelids is the palpebral fissure. This fissure is prevented from assuming a circular shape by the medial (nasal) and lateral (temporal) palpebral ligaments that attach each canthus to the respective orbital wall. The medial ligament inserts into the periosteum of the nasal bones, whereas the lateral ligament inserts into the temporal fascia and bones associated with the lateral orbit. In the dog, the lateral ligament is essentially replaced by the retractor anguli oculi muscle and its tendon; this in large breeds of dogs results often in entropion. Closure of the eyelids is achieved by contraction of the orbicularis oculi muscle located deep in the eyelids. Opening the eyelids is accomplished by relaxation of the orbicularis oculi muscle and contraction of the levator palpebrae superioris muscle, which inserts into the upper tarsus. Figure 1.10 Canine eye. Medial canthus (A), lateral canthus (B), cilia (C), NM (D), ciliary zone of iris (E), pupillary zone of iris (F), and collarette (G). Inset: Arrows indicate meibomian gland openings. Figure 1.11 Equine eye. (a) Medial canthus (A), lateral canthus (B), cilia (C), NM (D), lacrimal caruncle (E), ciliary zone of iris (F), pupillary zone of iris (G), and granula iridica (H). (b) Arrows indicate vibrissae. The upper eyelid has two to four rows of eyelashes (i.e., cilia) that usually begin near the medial quarter or third and either extend across to the lateral canthus or end shortly before the canthus (Figure 1.12). The lower eyelid has no cilia and has a hairless region approximately 2 mm wide adjacent to the eyelid margin extending the length of the lower eyelid and around the lateral canthus. The medial canthus, unlike the lateral canthus, has variable amounts of facial hair. In the cat, neither lid has cilia, but the leading row of hair from the medial third laterally on the upper eyelid is distinct enough in most cats to be considered cilia (accessory cilia or eyelashes). In the horse, a protuberance of variable size and pigmentation (i.e., the lacrimal caruncle) is present at the medial canthus. The lateral canthus is more rounded than that of the dog, and small amounts of bulbar conjunctiva and sclera are visible both medially and laterally. The exposed lateral conjunctiva is often pigmented. The cilia are well developed on the upper eyelid but absent on the lower eyelid. The facial hair is sparse adjacent to the lower eyelid margins at both the medial and lateral canthi and often at the medial upper eyelid. Horizontal folds are present in both the upper and lower eyelids. Vibrissae (long, specialized tactile hairs) are present on the base of the lower eyelid and on the medial aspect of the upper eyelid. Figure 1.12 Photomicrograph of the eyelid of a dog. Hair follicle (HF), cilia follicle (CF), palpebral conjunctiva (PC), tarsal gland (TG), skin (S), and orbicularis oculi muscle fibers (O). The eyelids protect the eyes from light, produce part of the tear film, spread the tear film across the cornea, and remove debris from the cornea and conjunctival surfaces. Through closure in a “zipper‐like” fashion from lateral to medial, the eyelids also direct the preocular tear film toward the nasolacrimal drainage system. Histologically, the eyelids consist of four parts: (i) the outermost layer contiguous with adjacent skin, (ii) the subjacent orbicularis oculi muscle layer, (iii) followed internally by a tarsus and stromal layer, and lastly (iv) the innermost layer, the palpebral conjunctiva (see Figure 1.12). The outer layer of the eyelid is skin covered by a dense coat of hairs with associated sebaceous and tubular glands. In dogs and cats, the hair follicles might be compound. Tactile hairs (pili supraorbitales), similar to the eyebrows of humans, may be present on or near the upper eyelids. Bundles of smooth muscle fibers, arrectores ciliorum, extend from the follicles of the eyelashes toward the tarsus. These muscle bundles are absent in carnivores and humans, but they are common in ruminants. The roots of the large cilia are in close association with prominent sebaceous glands (glands of Zeis) and modified apocrine sweat glands (glands of Moll, ciliary glands). These apocrine glands may provide host defense at the margin of the eyelids and possibly in the tears.
1
Development and Morphology of the Eye and Adnexa
Section I: Development of the Eye and Adnexa
Gastrulation and Neurulation
Human (approximate post‐fertilization age)
Mouse (day post‐fertilization)
Dog (day post‐fertilization or P = postnatal day)
Developmental events
Month
Week
Day
1
3
22
8
13
Optic sulci present in forebrain
4
24
9
15
Optic sulci convert into optic vesicles
10
17
Optic vesicle contacts surface epithelium
Lens placode begins to thicken
26
Optic vesicle surrounded by neural crest mesenchyme
2
5
28
10.5
Optic vesicle begins to invaginate, forming optic cup
Lens pit forms as lens placode invaginates
Retinal primordium thickens, marginal zone present
32
11
19
Optic vesicle invaginated to form optic cup
Optic fissure delineated
Retinal primordium consists of external limiting membrane, proliferative zone, primitive zone, marginal zone, and internal limiting membrane
Oculomotor nerve present
33
11.5
25
Pigment in outer layer of optic cup
Hyaloid artery enters through the optic cup
Lens vesicle separated from surface ectoderm
Retina: inner marginal and outer nuclear zones
11.5
29
Basement membrane of surface ectoderm intact
Primary lens fibers form
Trochlear and abducens nerves appear
Lid fold present
6
37
12
Edges of optic fissure in contact
12
30
TVL present
Lens vesicle cavity obliterated
Ciliary ganglion present
41
12
32
Posterior retina consists of nerve fiber layer, inner neuroblastic layer, transient fiber layer of Chievitz, proliferative zone, outer neuroblastic layer, and external limiting membrane
17
32
Eyelids fuse (dog)
7
Anterior chamber beginning to form
12.5
40
Secondary lens fibers present
48
14
32
Corneal endothelium differentiated
8
51
Optic nerve fibers reach the brain
Optic stalk cavity is obliterated
Lens sutures appear
Acellular corneal stroma present
54
30–35
Scleral condensation present
9
57
17
40
First indication of ciliary processes and iris
—
EOMs visible
Eyelids fuse (occurs earlier in the dog)
10
45
Pigment visible in iris stroma
Ciliary processes touch lens equator
Rudimentary rods and cones appear
45–1P
Hyaloid artery begins to atrophy to the disc
3
12
—
Branches of the central retinal artery form
4
51
Pupillary sphincter differentiates
Retinal vessels present
—
56
Ciliary muscle appears
—
Tapetum present (dog)
5
40
Layers of the choroid are complete with pigmentation
6
—
Eyelids begin to open, light perception
1P
Pupillary dilator muscle present
7
1–14P
Pupillary membrane atrophies
1–16P
Rod and cone inner and outer segments present in posterior retina
10–13P
Pars plana distinct
9
16–40P
Retinal layers developed
14P
Regression of pupillary membrane, TVL, and hyaloid artery nearly complete
Lacrimal duct canalized
Ocular part or event
Gestational size (mm)
Lens
Optic vesicle
6
Lens placode
6
Optic cup and lens placode
10
Separation of lens vesicle from surface ectoderm
10
Primary lens fibers
15
Lens vesicle cavity disappears
24
Completion of lens capsule
50
Secondary lens fibers
58
Perilenticular vascular mesoderm
Extension of primary vitreous (hyaloid artery) to lens
15
TVL
33
Disappearance of posterior lenticular vascular network
410
Disappearance of TVL
410
Iris
Major arterial circle of iris
90
Iris reaches front of lens
200
Pigment in stroma
200
Sphincter muscle
410
Dilator muscle
410
Ciliary body
Ciliary processes
125
Ciliary processes touch lens equator
230
Pars plana (distinct)
200
Pars plana fully developed
410
Choroid
Choroidal net in posterior pole
33
Choroidal net throughout
50
Outermost large choroidal vessels
40
Choriocapillaris
90
Pigmentation of choroid
90
Retina – posterior third
Inner and outer nucleated zones
10
Multilayer outer cup of optic vesicle forms single cells
20
Nerve fiber layer
20
Optic nerve well formed
24
Inner/outer neuroblastic layers
14
Transient layer of Chievitz
14
Inner plexiform layer
180
Retinal vessels
180
Tapetal cells
410
Neural ectoderm
Neural crest
Neural retina
Stroma of iris, ciliary body, choroid, and sclera
RPE
Ciliary muscles
Posterior iris epithelium
Corneal stroma and endothelium
Pupillary sphincter and dilator muscle (except in avian species)
Perivascular connective tissue and smooth muscle cells
Striated muscles of iris (avian species only)
Bilayered ciliary epithelium
Meninges of optic nerve
Orbital cartilage and bone
Connective tissue of the extrinsic ocular muscles
Endothelium of trabecular meshwork
Surface ectoderm
Mesoderm
Lens
Extraocular myoblasts
Corneal and conjunctival epithelium
Vascular endothelium
Lacrimal gland
Schlemm’s canal (human)
Posterior sclera (?)
Formation of the Optic Vesicle and Optic Cup
Lens Formation
Vascular Development
Development of the Cornea and Anterior Chamber
Development of the Iris, Ciliary Body, and Iridocorneal Angle
Retina and Optic Nerve Development
Sclera, Choroid, and Tapetum
Vitreous
Optic Nerve
Eyelids
Extraocular Muscles
Section II: Morphology of the Eye and Adnexa
Introduction
Orbit
Dimension
Feline (mm)
Canine (mm)
Bovine (mm)
Equine (mm)
Width
24
29
65
62
Height
26
28
64
59
Depth
—
49
120
98
Distance between orbits
23
36
151
173
Orbital Fascia
Foramen or fissure
Species
Associated nerves and vessels
Alar, rostral
Canine, equine, feline
Maxillary artery and nerve
Ethmoidal (one or more)
All species
Ethmoidal vessels and nerve
Orbital
Canine, equine, feline
Abducens, oculomotor, ophthalmic, and trochlear nerves
Orbitorotundum
Bovine
Cranial nerves III–IV, retinal and internal maxillary arteries
Optic
All species
Optic nerve, internal ophthalmic artery
Rotundum
Canine, equine, feline
Maxillary nerve
Supraorbital
Bovine, canine, equine (feline variable)
Supraorbital vessels and nerve
Caudal palatine
All species
Major palatine vessels and nerve
Maxillary
All species
Infraorbital vessels and nerve
Sphenopalatine
All species
Sphenopalatine vessels and pterygopalatine nerve
Extraocular Muscles and Orbital Fat
Muscle
Function
Nerve supply
Dorsal (superior) rectus
Rotates globe upward
Oculomotor
Ventral (inferior) rectus
Rotates globe downward
Oculomotor
Medial rectus
Rotates globe medially
Oculomotor
Lateral rectus
Rotates globe laterally
Abducens
Dorsal (superior) oblique
Rotates dorsal part of globe medially and ventrally
Trochlear
Ventral (inferior) oblique
Rotates ventral part of globe medially and dorsally
Oculomotor
Retractor oculi (bulbi)
Retracts globe
Abducens
Levator palpebrae superioris
Raises upper eyelid
Oculomotor
Orbicularis oculi
Closes palpebral fissure
Facial
Retractor anguli oculi
Lengthens lateral palpebral fissure
Facial
Eyelids
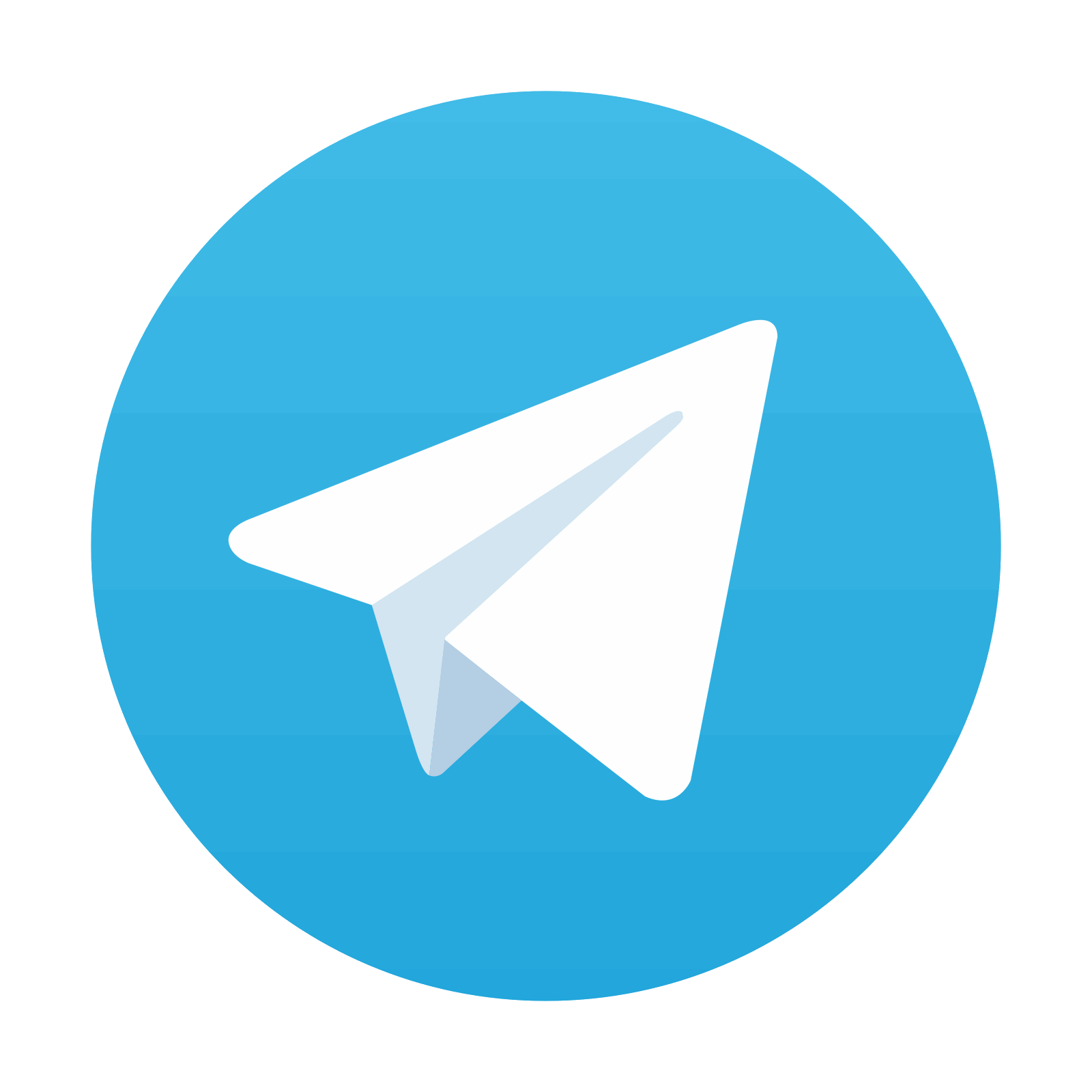
Stay updated, free articles. Join our Telegram channel

Full access? Get Clinical Tree
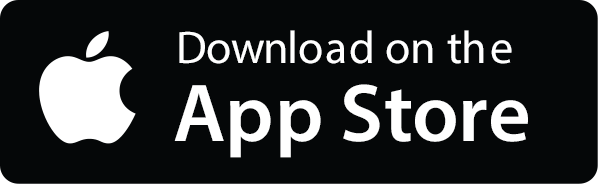
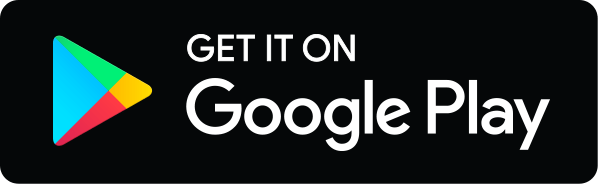