15 James M. Fingeroth, Franck Forterre, and Jonathan M. Levine When confronted with a patient with signs thought to be attributable to displacement of an intervertebral disc (IVD), one has to consider a range of prognostic and therapeutic possibilities. It can be helpful for clinicians to consider what is transpiring at the level of the spinal cord and how the interaction of the displaced disc with the spinal cord might influence the observed clinical signs and the utility/urgency of possible interventions. For the purposes of simplification, we can consider compressive spinal cord injury (SCI) to represent spinal cord deformation and shifting from its normal position within the vertebral canal. Contusive SCI, on the other hand, is the actual disruption of parenchyma (neuronal cell bodies, axons, glial cells, myelin, and microvasculature) within the substance of the spinal cord. A simplified formula summarizes the primary (mechanical) events that occur in disc-associated SCI: where x and y are the relative contributions of mechanisms to SCI and x + y = 100%. While there is clearly going to be some overlap between these processes when an IVD displaces and contacts the spinal cord, it can be instructive to consider them as somewhat distinct entities for the purposes of clinical decision making. It is important to recognize that following compression or contusion, a series of secondary biochemical processes occurs including inflammation, oxidative stress, vascular events, and neurohormonal changes. Surgical intervention is best thought of as a means to relieve ongoing compressive SCI, which may in turn reduce the progression of these secondary mechanisms. Seminal experimental studies by Tarlov and others demonstrated how slowly applied compression (such as inflation of an epidurally placed balloon catheter) would result in a predictable pattern of neurologic dysfunction and how, equally or more importantly, deflation of such catheters and thus “decompression” resulted in a predictable pattern of neurologic recovery [1]. We have long recognized this clinically, with many literature references establishing how paralyzed dogs will first recover normal nociception followed by recovery of motor function in the affected limbs and finally fine motor skills and coordination [2, 3]. These experimental studies and clinical experience demonstrate that discomfort may be the first or only sign of disc displacement, followed by proprioceptive loss and ataxia, then paresis or paralysis, and finally loss of nociception. The main deleterious effect of spinal cord compression (deformation/displacement) is demyelination, without transection of neurons or blood vessels [4]. Compression causes relative ischemia, and this ischemia, in turn, is responsible for the failure to maintain myelin coating on neurons [5, 6]. The axons and cell bodies themselves are more resistant to this relative level of ischemia, so are less affected. While dwell time (the duration of compression) can be a factor in the severity of signs and the eventual development of intraparenchymal lesions beyond demyelination, we know that patients with even long-standing spinal cord compression, when it is slowly applied, can have complete and often rapid recovery once decompression is achieved [4, 7, 8]. Myelin serves as an “insulator” that helps speed electrical impulse conduction along the axon. After demyelination, conduction block might occur leading to paresis or even paralysis. Recovery occurs rapidly (within a few weeks) by restoration of continuous conduction prior to the return of saltatory conduction. This may be best illustrated in the clinical setting in animals with slow growing, extramedullary spinal cord tumors. In some cases, the spinal cord can be compressed down to a thin crescent, yet patients may have modest neurological deficits, and rapid improvement once the tumor has been excised. So, the take-home message is that pure compression can be tolerated to an extraordinary degree by the spinal cord (especially when applied slowly), either producing minimal clinical signs (such as discomfort alone—usually due to nerve root compression/ischemia) or a predictable and gradual loss and then recovery of function. Contusive SCI, on the other hand, is internal disruption of the parenchyma with or without any associated compression. In addition to myelin disruption, there is actual compromise of the structural integrity of the spinal cord, including necrosis. Since the repair capabilities of the spinal cord are limited (see Chapter 25), such damage implies more severe neurological deficit and a slower, perhaps less complete recovery (or no recovery at all) regardless of treatment. When discs displace, they can obviously cause a combination of compression and contusive SCI. Even with advanced imaging, we cannot know with precision the degree of each and thus will always have some uncertainty with respect to appropriate intervention. However, there are clues we can discern based on the history, clinical signs, and imaging studies that allow us to at least decide whether compression or contusion predominates, and thus help decide on a treatment strategy and prognosis. For example, it is intuitive and likely that a patient that develops signs gradually (over hours, days or longer) and, where those signs fit the pattern of initial discomfort followed by ataxia or proprioceptive loss and finally weakness, is much more likely to be suffering the effects of compression than parenchymal injury. Conversely, a patient that becomes suddenly paraplegic or tetraplegic within seconds or minutes may be more likely to have some degree of spinal cord contusion. The key tool for discrimination is spinal cord imaging (see Chapter 16) whereby the amount of compression (or lack thereof) can be ascertained, and that information is added to the clinical features (severity of deficits and onset of signs) to posit a mental construct of what is occurring at the level of the spinal cord. MR signal changes within the spinal cord (increased T2 signal) may be associated with contusion and have been shown to be a negative prognostic indicator in the setting of IVD herniation independent of physical examination based evaluation. Interestingly, no correlation between compressive indices and long-term outcome has been identified [9]. Clinicians must always bear in mind that surgery for IVD herniation is, regardless of the nuances of particular technique, generally limited in therapeutic value to spinal cord decompression. And, equally important, such decompression is only achieved when a deforming mass (disc, tumor, bone fragment, etc.) is effectively removed and the dural tube (with the enclosed spinal cord) is returned to its normal position and configuration. As noted in Chapters 9, 13, 28, and 33, there is minimal to no decompressive or other positive therapeutic effects to simple bone removal or durotomy when there is internal SCI. We cannot yet repair spinal cords with surgery. So, the basis for recommending surgery is the demonstration of spinal cord compression and the belief that the contusive component is not so severe as to preclude a beneficial effect from decompression. The latter is not always knowable (especially when clinical deficits are very severe, such as patients with apparent loss of nociception caudal to their lesion), but unless there is at least some degree of compression present, it is fair to conclude that surgery would not likely be helpful for a patient where imaging discloses no macroscopic extradural compressive lesion. What determines the relative degrees of compression and contusion when a disc herniates? The herniation of an IVD is governed by the Newtonian laws of physics. The herniated portion of the disc has mass, and it impacts the spinal cord with certain velocity. The kinetic energy delivered to the spinal cord can thus be defined as ½ mV2, where m is the mass and V the velocity (or the force of the herniation can be defined as mA, mass times acceleration). We can further conclude that the greater the bolus of kinetic energy or force delivered to the spinal cord, the greater the risk for and degree of parenchymal injury [10, 11]. Therefore, when one considers the patient presented with signs attributable to suspected IVD herniation, one should consider the mass and velocity components of the equation, bearing in mind that the velocity component predominates because its value is squared. The clinical implications of this concept are profound. For example, if one imagines a large mass of disc that herniates with close to zero velocity/acceleration, the resultant kinetic energy and force will also be close to zero. A patient so affected might be expected to have very modest signs (perhaps only some discomfort due to nerve root compression) and no other deficits despite severe spinal cord compression. This is exactly analogous to the prior examples of slowly applied compression from a balloon or tumor (“slowly” being the semantic equivalent of a low velocity). This concept is important to bear in mind as it demonstrates that there may be no correlation between the size of the mass (i.e., degree of compression) and the severity of signs in dogs. It is often seen in the clinical setting where patients with, say, severe and chronic neck pain are treated for prolonged times with conservative, nonsurgical methods because the clinician was under the misapprehension that such modest signs indicated that there was minimal compression to be concerned about and thus no justification for considering imaging or surgery. This is also seen in thoracolumbar disc herniations where the patient is ambulatory and has minimal neurologic deficits, but imaging demonstrates marked spinal cord compression. Conversely, a minute fragment of disc (low mass) ejected at high velocity will deliver a huge bolus of energy and force to the spinal cord (perhaps resulting in focal myelomalacia), and yet there will be no compressive element for which surgery could play a role in treatment. These are so-called noncompressive discs described elsewhere (Chapters 4, 6, 9, and 13). Further, the degenerative state of the disc may also play a role in the pathophysiology of the lesion. It can be expected that a nondegenerated disc in which higher pressures are exerted will mainly lead to a severe contusion when extruding. Its high water content will also lead to its rapid distribution within the spinal canal without severe spinal cord compression. It is also apparent how two patients, presented with identical neurological examinations and deficits, can have very different outcomes regardless of treatment. In conclusion, prognostication and therapeutic recommendations for patients with suspected IVD herniation can largely be based on a careful consideration of the onset of clinical signs, the severity of those signs, the degree of spinal cord compression (and bearing in mind that the latter can only be defined by imaging, and not based on the severity of signs), consideration of estimated mass and indirect signs indicating high velocity of the disc herniation (high T2 signal with minimal compression), and theoretical envisioning of the degree to which spinal cord compression vs. spinal cord contusion is contributing to the observed signs. In recent studies, a supplementary epidural inflammatory role has been attributed to the herniated disc itself. This finding would modify the initial formula to
Compressive and Contusive Spinal Cord Injury Secondary to Intervertebral Disc Displacement: A Clinical Perspective
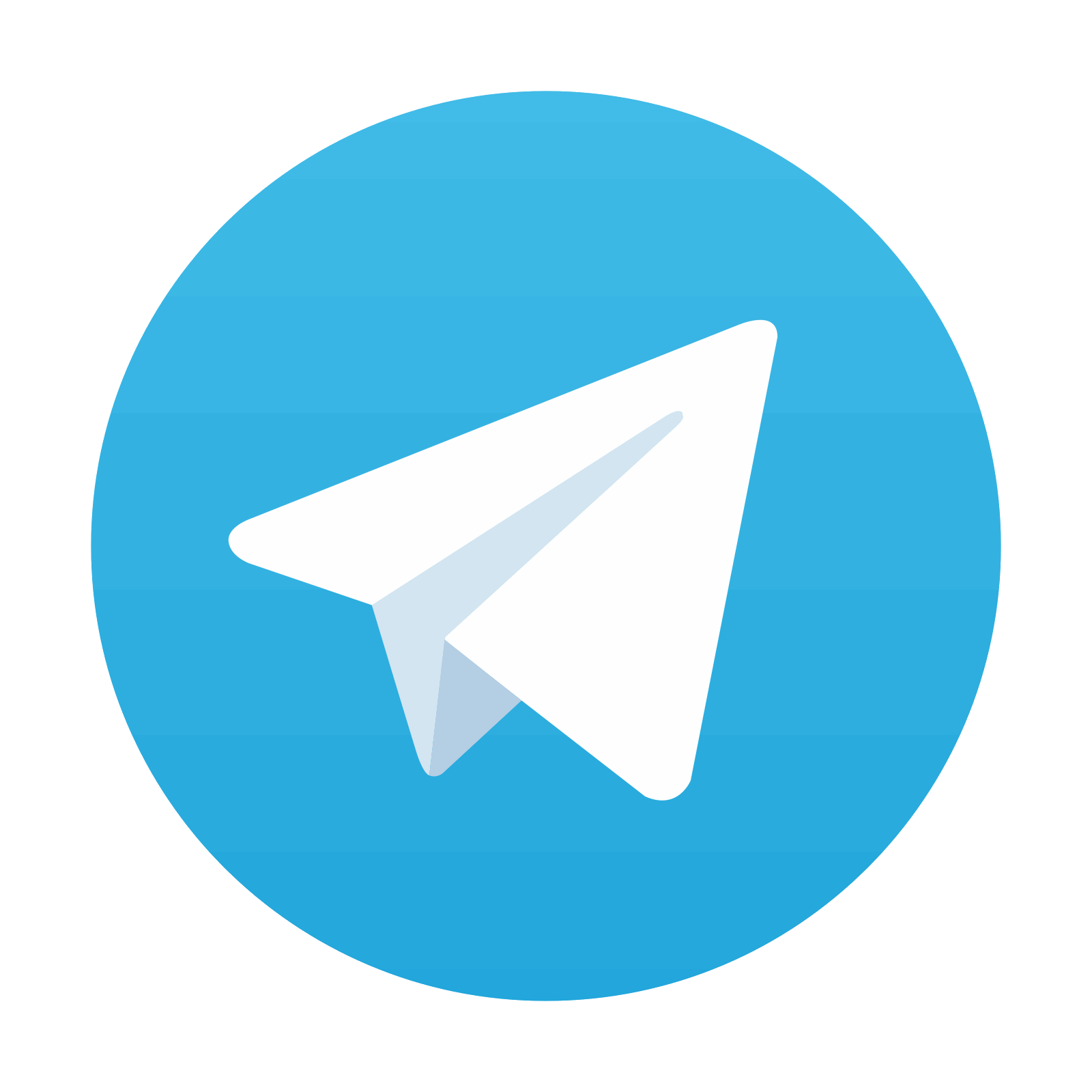
Stay updated, free articles. Join our Telegram channel

Full access? Get Clinical Tree
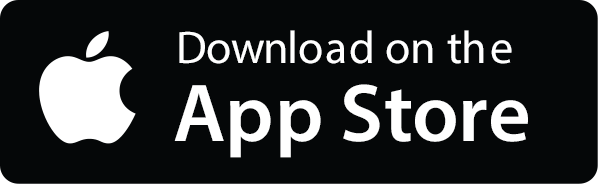
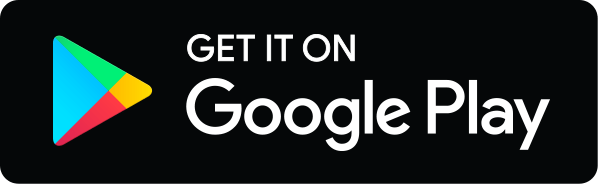