Jeffery Biskup and Michael G. Conzemius Despite its present unpopularity, intraarticular (IA) repair has been researched and performed clinically since the mid‐twentieth century. A more in‐depth description of the history of IA repair can be found elsewhere, although it is interesting to note that 30 years ago, IA repairs were more common in veterinary medicine and often the preferred repair technique for certain demographics of dogs [1–4]. In 1952, an IA repair was described utilizing a lateral fascia lateral graft placed through tibial and femoral bone tunnels and anchored with suture to the soft tissue external to the tunnels [5, 6]. This original repair was modified throughout the years until the “over the top” and “under and over” techniques were developed [57–9]. The decline of IA repairs was likely a combination of personal observation of outcomes of IA repair compared to current extraarticular repairs (including tibial osteotomies and extraarticular sutures), papers with objective outcomes showing superior results with extraarticular repairs [10, 11], and studies demonstrating continued stifle instability, progressive cartilage degeneration, and donor site morbidity with IA repairs [10–17]. These findings are not surprising given the limited mechanical properties of the fascia lata and suture [18]. Despite these limitations, it is possible that the concept of an IA repair was abandoned too quickly and can better mimic the mechanical function of the native cranial cruciate ligament (CCL) compared to tibial osteotomies and extracapsular sutures. By assessing the complications of these previously described techniques, we can hopefully learn and improve future IA repairs techniques. An extensive review of human anterior cruciate ligament (ACL) repair techniques and complications is beyond the scope of this chapter but a brief review is important to help understand complications in human knee repair and what we may observe on the veterinary side. In humans, surgery continues to be considered the gold standard for high‐performance athletes and adolescent patients, with persistent instability in 75% of patients who do not undergo surgery [19]. The gold standard ACL repair technique in humans is reconstruction of an ACL by IA placement of a graft at the origin and insertion of the ACL. Currently, there is no consensus on the ideal graft nor on the method of fixation [20, 21] and new methods of ACL reconstruction, like bridge‐enhanced ACL repair, continue to be investigated. To help demonstrate the variety of repair combinations, common grafts include a variety of autografts such as the central third of the patella ligament (bone‐ligament‐bone graft), hamstring graft, quadriceps graft, allografts from similar donor sites, and prosthetic grafts. Furthermore, it is debated if the grafts should be single, double or quadruple bundled or twisted. Similarly, debate exists among surgeons regarding exact bone tunnel location, graft fixation, graft preconditioning, and graft tensioning. Last, meniscus status can play a large role in outcome, with 50.4% of patients who had a partial or total meniscectomy having osteoarthritis compared to 16.4% without a meniscectomy [22]. Due to this variety, a blanket summary about ideal repair, complication rate, and complication types in human medicine cannot be made. This section rather aims to give an overview of complication rate ranges and the common complications seen in human medicine. This may allow veterinary medicine to better anticipate possible complications after IA techniques and set goals to equal or exceed human success rates. Complications in human medicine are often characterized into donor site complications during autograft harvesting or repair complications. Donor site complications include pain or numbness (7–57%), patella fracture (7%), and patella tendon rupture (7%) [23]. Repair complications included loss of range of motion of the knee (2–50%), infection (1–2%), hardware removal (5–12%), postoperative meniscal tears (6–10%), and pain during kneeling (44%). Three recent human systemic reviews have assessed success and complications. A study by de Sa et al. compared an “all‐inside technique” and found that autografts (73.8%) continue to be more commonly used compared to an allograft (26.2%) [24]. The complication rate was 5.89% over the 13 studies assessed but the mean follow‐up time was limited to only 18 months. Complications included graft rupture (2.47%), loss of knee extension between 1° and 10° (1.14%), and cartilage or meniscal injuries (0.76%). A second recent systematic review compared an “over the top” human technique to placing the graft at the footprints of the ACL [25]. No difference was found between the two techniques, with 94% returning to sports and 69% returning to preinjury level. Another review of 20 studies compared the success of surgery for children and adults [26]. Overall, 92% of people returned to sports, with 78.6% reaching preinjury performance and no difference between groups. The major complication was graft rupture with a high rate of 14%. In high‐performance athletes, 83% were able to return to their sport 6–13 months after surgery [27]. Although some athletes did not return to preinjury level, many performed as well as athletes without an injury. A second paper with a 10.5‐year follow‐up found 98% of amateur athletes returned to sport, with 71.6% returning to their preinjury level [28]. As a comparison, after a tibial plateau leveling osteotomy (TPLO), 65–68% of agility dogs are able to return to activity, although one study found close to 50% of dogs that returned to agility competed at a lower level [29, 30]. Revision surgery is an important area of research in human medicine. Potentially relevant to veterinary medicine, high tibial osteotomies to correct posterior tibial slope during placement of a graft are becoming more common for revision surgery and contemplated for the primary surgery. In humans, an excessive tibial slope is considered to be >12° and is commonly corrected to <5° [31]. Other studies suggest that decreasing the posterior slope helps improve anterior instability and patients who undergo primary repair and have their posterior slope decreased by >5° have a better functional outcome than patients who do not have their slope lessened [32, 33]. In a systematic review of 60 revision surgeries with high tibial osteotomies, no graft rerupture was reported, with the only complication being stiffness in the knee in one case [34]. Fifty‐eight percent of these patients had osteoarthritis at follow‐up, although 51% had osteoarthritis before the revision surgery. Besides correcting tibial slope, surgeons can concurrently correct varus. A study used high tibial osteotomies on patients undergoing primary ACL repair who also had medial compartment osteoarthritis [35]. A correction from 7° varus to 1.2° valgus resulted in improved side‐to‐side instability and improvements of functional outcome measures. It may be necessary to perform similar corrections in dogs for IA repair to be consistently successful. While this may be ideal, it may also result in a procedure with an enormous financial burden to the owner and excessive surgical risks to the patient. Assessing complications of IA repair in dogs is even more difficult than assessing effectiveness as many studies were performed on a small number of research dogs where CCL transection was performed. This model does not fully reproduce the naturally occurring disease because of patient predisposition to joint disease, duration of disease prior to surgery, postoperative management, etc. Also, there is great variation in graft selection and fixation technique between studies. In addition, for an IA repair to have a chance of being successful, the graft/implant and graft fixation technique need to be strong enough to counteract forces transferred through the stifle during recovery (leash walks, sitting, standing, etc.). Very few studies assess the initial strength of the graft or fixation technique, raising the question whether the technique investigated had little chance of success. Certainly, the biology of any graft repair is important but if the graft cannot survive the mechanical environment it is in, the biology becomes irrelevant. That being said, some studies have objective outcomes such as histopathology and mechanical testing that have greatly aided our understanding of IA repairs. The most common complication reported is graft failure, with progression of osteoarthritis, infection, meniscal injury, and continued instability also described. In the following section, relevant literature will be used to further discuss the frequency of these complications. A study by Thorson et al. placed bone‐ligament‐bone auto‐ and allografts in dogs that had their CCL transected 1 month previously. In the allograft group, 3/11 dogs developed infections and an additional two dogs had no visible graft at the completion of the study (four months post‐operatively) [36]. In contrast, autografts (n = 4) were reported as viable at the completion of the study. The allografts caused more inflammatory reaction on the outside of the graft than the autografts. In a study that utilized bone‐ligament‐bone auto‐ or allografts in nine dogs, 67% of the grafts survived (9 month follow up) [37]. Despite a easonable graft survival rate, mechanical strengths of the grafts were <14% of the contralateral normal CCL. Neither of these studies reported the mechanical strength of the repair used and the results suggest that mechanical overload and a lack of biological ligamentization played a role in failure. In contrast, a study placing allograft ligaments to replace the CCL gained 90% of CCL strength by 36 weeks [38]. Additional studies have found both positive and negative ability of the graft to heal [12, 39, 40]. The effectiveness of the “over the top” and “under and over” techniques is difficult to assess as most reported outcomes were subjective, retrospective or ex vivo mechanical evaluations and did not directly compare these procedures to current repairs, although some papers had some promising results [41–43]. Unfortunately, most available clinical studies assessing the “over the top” and “under and over” techniques look at clinical outcome and success rate and not specific types or reasons for complications [11, 44]. A long‐term study looked at dogs with naturally occurring CCL disease repaired with a fascial “over the ‐top” graft and assessed outcome with an owner questionnaire and force platform analysis. With almost 1.5‐year follow‐up, owner satisfaction was very high, although gait analysis revealed significantly decreased peak vertical force [45]. Osteoarthritis appears to continue to progress in spite of surgical repair in other studies [13]. Similar results were found in 21 dogs with patella tendon/fascia lata grafts where all owners felt their dog showed significant improvement, although instability and progressive osteoarthritis were commonly documented [46]. Ongoing instability or lengthening of grafts also appeared to be a common complication [12, 47]. A study with 50‐month follow‐up of the “over the top” technique showed a trend of declining function after improved function over the first year postoperatively [3]. The same researcher found that 60% of dogs had progressive osteoarthritis after IA repair [10]. Other complications less commonly reported include excessive scar tissue at the incision and decreased range of motion [48]. Recently, a number of prospective research studies have been published, looking to learn from past mistakes and overcome the weaknesses of previous repairs. One study looked at placement of IA synthetic grafts [49, 50]. A multifilamentous ultra‐high molecular weight polyethylene terephthalate core contained within a braided porous nonexpanded polytetrafluoroethylene sheath ligament was placed through 4.5 mm femoral and tibial tunnels and secured with an interference screw and a spiked washer and screw on both the femoral and tibial tunnels. The repairs immediately eliminated cranial drawer but in vivo placement had an unacceptably high complication rate. Reported major complications included fixation failure, implant failure, infection, medial luxating patella, meniscal tear, and recurrent instability. All dogs with complications achieved satisfactory results, often with a staged explantation of the implants and a tibial tuberosity advancement. Work by Lopez et al. evaluated a hamstring autograft that was twisted to a diameter of 4.5 mm and secured on the tibia and femur with bone staples [51]. Although the study investigated only four dogs with induced CCL injury, the animals had positive outcomes using subjective and objective outcome measures. Similarly, two clinical trials evaluated an allograft technique that placed IA allografts secured with a femoral cross pin and tibial spiked washer and screw and separate interference screw in the tibial tunnel, referred to as the TransFix® technique (Arthrex Inc., Naples, FL) (Figure 4.1). Before moving forward with clinical cases, in vitro mechanical testing showed graft decellularization minimally affected graft mechanics [52] and initial graft and fixation strength was comparable to a native CCL of a similarly sized dog [53, 54]. When patients had an intact graft at 1 year, they had an excellent clinical outcome. Unfortunately, the technique had a 58% graft failure rate, combining the two studies [55, 56], which lead to a conclusion that although IA grafts can succeed, the technique described was not the solution. Given the high failure rate, it is tempting to abandon IA repairs but understanding the reason for the complications may lead to improvement. Furthermore, major complications of current osteotomy repairs can be upwards of 30%, suggesting that both techniques need improvement [57, 58]. It is suspected that failure with the TransFix® technique was mechanical, as some dogs had femoral‐tibial instability as early as the 8‐week recheck and no overt signs of graft inflammation were visualized during arthroscopy 1 year postoperatively. If the graft was rejected, it was suspected the remaining graft ends would likely cause continued inflammation. It was hypothesized that graft failure was due to surgeon error (graft damage during placement or improper fixation) or overloading during the early postoperative period. For example, in one dog, the passing wire that was used to pull the looped graft through the bone tunnel twisted and broke. On postoperative radiographs, a 5 mm piece of the wire was identified at the most proximal aspect of the bone tunnel. In humans, it has been shown that a graft weakens during the ligamentization process before regaining strength as native cells repopulate the graft. This highlights the importance of postoperative activity restriction or secondary protection of the graft. Figure 4.1 Radiograph of a stifle joint with a DDFT allograft secured by use of transverse femoral fixation (allograft looped around a femoral cross pin) and stabilized with a tibial interference screw and two spiked washers on the tibia (DDFT‐TF).The tunnels are highlighted with the white outline. In three other dogs, infections at the tibial bone tunnel exit occurred during the study period, all before the 6‐month recheck. Two cases developed draining tracts that could be controlled with antibiotics but when antibiotics were stopped, the draining recurred. Surgical explantation of the screw, washer, and any braided, nonabsorbable suture resolved the infection but the graft was not present on arthroscopy. The third case had radiographs performed that revealed bone lysis at the tibial post site and a minimally displaced tibial fracture. The patient was managed nonsurgically with a splint for 4 weeks, exercise restriction for an additional 8 weeks and antibiotics. Surgical explantation of the screw, washer, and any nonabsorbable suture was performed after the fracture had healed. Smith et al. assessed a patella bone‐tendon‐bone ligament autograft and a quadriceps tendon allograft soaked in platelet‐rich plasma with a nonabsorbable internal brace in a CCL transection model [59]. At the conclusion of the study 6 months postoperatively, all grafts were intact. The five dogs that received quadricep grafts with an internal brace had similar outcome measures to the control limbs with intact CCLs. Complications seen, more often in the patella group, included residual lameness, pain, effusion, and decreased range of motion of the stifle. Also, the patella graft group saw progression of osteoarthritis and mild fibrillation on arthroscopy. Unique to the quadriceps group, the internal brace was visible in two dogs. Both graft types showed good healing as assessed by histopathology and had similar mechanical strength to the intact CCL during biomechanical testing. This study raises the possibility that biologics, such as platelet‐rich plasma or stem cells, may enhance healing of the graft during ligamentization. Based on the available evidence, the ideal graft, graft size, graft length, and graft placement (for example, twisting the graft) are unknown. Furthermore, the ideal initial graft strength, fixation strength, and tension at the time of placement have not been determined in dogs. It is logical to assume that if the graft has similar strength to the native CCL, the strength is adequate. Certainly, that was the goal in more recent studies. However, grafts weaken during healing and ligamentization, a process that takes many months, before regaining strength. In dogs, exercise restriction after surgery for a 4‐month period can be challenging, so it may be that initial strength has to be greater than CCL strength. Initial strength has to be balanced with graft size limitations. In many studies, the maximum size graft that could be safely harvested (autograft) is placed. It is suspected that many autograft techniques have failed because the largest graft possible does not have adequate strength or was not protected (such as internal brace, osteotomy) during healing. In veterinary medicine, using a portion of the straight patella ligament or a strip of the fascia lata is the most commonly reported autograft, although skin, peroneus longus, flexor digitalis pedis longus, long digital extension, periosteum, and semitendinosus/gracilis autografts have all been described [5, 14, 51,60–64]. Some of these grafts were harvested and used as free grafts, others maintained an original attachment that may have preserved some vascular supply. For example, patella tendon autograft use, a technique modified from human medicine, allows for either bone or ligament anchor points. The distal end of the graft maintained attachment to the tibia, while the free proximal end was passed through a femoral tunnel and sutured to the gastrocnemius. Very few studies have assessed graft strength or reported dimensions. Tensile testing of the medial third, central third, and lateral third in combination with the fascia lata revealed that no graft was able to mimic the strength or stiffness of the native CCL. Although the central and lateral thirds were stronger than the medial third, it is likely that no partial patella ligament graft has the mechanical properties necessary for recovery in the dog [12, 65]. In fact, it has been reported that the entire patellar tendon is needed to reproduce the mechanical properties of the CCL [53]. The “under and over” technique utilizes the lateral third of the patella tendon and the fascia lata. Described techniques maintain the distal attachment making the graft 15–25 mm wide and 2–3 times the length of the patella‐tibial tuberosity length, depending on the method of fixation [47]. Similarly, the “over the top” technique utilizes the medial third of the patella ligament before crossing the patella (harvesting nonarticular bone in the process) and continuing into the fascia lata [66]. The fixation of these grafts varied from suturing to the gastrocnemius to securing them around a screw. Ex vivo mechanical testing of these methods suggests their mechanical characteristics are far below those of the intact CCL [18]. Again, this is not only because of the strength of the graft but because of limitations in graft fixation techniques. Harvesting a hamstring graft has been described by Lopez et al. [51]. A medial skin incision is made over the medial tibia, starting from the patella and heading distally down two‐thirds of the tibia. The insertions of the gracilis and semitendinosus muscles are protected while the tendons are dissected free of their muscular origins. The attached dense connective tissue and muscle fascia of the medial aspect of the cranial tibialis muscle are freed to two‐thirds the distance of the tibia. The benefits of using allografts include a natural extracellular matrix (ECM) scaffold with no donor site morbidity, the ability to use entire ligaments (e.g., using the entire patella ligament rather than a third of it), having the donor ligament readily available (no intraoperative collection), and increasing the options of ligaments (e.g., use of the CCL as an allograft). Although allografts have these advantages, they raise concerns about disease transmission, immunological reaction, and alteration of ingrowth characteristics. Use of allografts has been described in veterinary medicine [39, 67], with studies looking at the ideal graft size, mechanical strength, histology, and clinical outcome [68]. Proper harvesting of allografts requires an understanding of anatomy, asepsis, quality control, and quality assurance. Graft size is often chosen based on the largest tunnel that can be safely drilled in the femur and tibia. In the authors’ experience, dogs <32 kg can receive 6 mm tunnels, dogs ≥32 kg and <40 kg can have 6.5 mm tunnels, and dogs ≥40 kg can have 7 mm tunnels created. Of course, individual variability exists beyond body weight. Graft length depends on the fixation technique. For example, for the TransFix® technique, a graft has to be much longer ([length of tibia tunnel + length of femoral tunnel + native CCL length] × 2 + 2 cm for fixation) than a technique that uses a single‐strand graft secured in the tunnels. The CCL is not a simple band and the fan‐like attachment to the axial aspect of the lateral condyle and craniomedial tibia, underneath the intermeniscal ligament, makes placement of bone tunnels challenging. In humans, it has been shown that bone tunnel placement at the footprints of the ACL may not be perfectly isometric points. In dogs, it is suggested that the isometric location may be caudal to the origin of the CCL on the femur and cranial to the footprint on the tibia. Given the complexity of movement in the stifle during range of motion, it was decided to place the origin of the bone tunnels at the footprints for the TransFix® procedure. The authors found it quite straightforward to identify the footprint, measure its size, and drill a guide pin at the desired angle through the center of the footprint. However, the angle of the guide pin often had to be adjusted so the selected drill bit could be used without causing iatrogenic damage to the femoral condyle. The optimal method of graft fixation is not known in human medicine and likely depends on the type of graft utilized. Multiple techniques for securing bone‐ligament and ligamentous grafts have been described, including interference screws, pinning, suture anchors, spiked washers, and suture [69]. Interference screws are easy to use, size can be changed according to size of the graft/tunnel ratio, and they improve contact between the bone tunnel and graft. When using grafts that are folded to double the graft, such as the hamstring tendon or deep digital flexor tendon (DDFT), a cross pin technique can be used. Cross pin transfixation techniques pass a pin through the loop created at the fold in the graft (Figure 4.2). The remaining graft ends can be secured with one of the previously mentioned techniques. Suture and anchors appear to be gaining popularity for human graft fixation given the ease of use and versatility. Often a braided suture is incorporated into the graft, exited out the bone tunnel and secured to a bone anchor or similar fixation system. The main concern with this fixation is having braided material in the joint and bone tunnels. Figure 4.2 Intraoperative photograph of a DDFT allograft repair using the TransFix® system to secure the looped ligament on the femoral side and spiked washers (open arrow) and interference screw (closed arrow) to secure the ligament on the tibial side. Empirically, IA cases that mechanically fail tend to fail early in the recovery process. Failure at the fixation site is a concern due to inappropriate initial strength. A unique challenge in the dog is that initial fixation strength has to overcome daily forces generated by the patient. Often a gradual increase in weight bearing is not possible as it is in humans. For example, in cadaver canine stifles, it was shown that an entire patella ligament secured with interference screws or bone anchors was likely not strong enough to withstand daily physiological loads, although these fixation techniques are used in humans [54]. Before attempting any IA repair, it is important that the mechanical strength of the graft–fixation technique combination is determined. If there is fixation failure, it is likely that the graft is damaged and needs to be replaced. If an autograft was utilized, an allograft may need to be considered on revision. Similarly, damage to local anatomy has to be assessed to determine if a similar fixation technique can be used. The authors suspect that with a failed IA repair, an extracapsular suture or tibial osteotomy could be pursued after explantation of implants and graft. More specifically, a CORA‐based leveling osteotomy (CBLO) might be a better tibial osteotomy technique because the osteotomy site could be made below any existing proximal tunnel sites. Recheck examinations are important to assess IA graft repair as the presence of stifle instability documents that the graft is no longer functional. Nonweight‐bearing radiographs can help assess implants and bone tunnels although bone tunnels cannot be clearly identified in all cases. Similarly, weight‐bearing radiographs may help document elongation/failure of a graft. Subluxation measurements can be compared to the contralateral limb, assuming there are no abnormalities in that stifle [55, 70]. Palpation and radiographs may still miss some graft failures as direct palpation and the patient standing induce loads below physiological forces required for walking, running or jumping on the limb. Currently there are no guidelines for the acceptable amount of cranial drawer or subluxation on weight‐bearing radiographs. A normal CCL allows 2–3 mm of femoral‐tibial translation and, while patients can have good long‐term outcomes (i.e., owner survey, gait analysis) with some instability, it has been reported that after IA repair dogs without palpable instability and an intact graft generally have an excellent outcome [56]. Empirically, after IA reconstruction, patients that developed femoral‐tibial instability greater than 10 mm had lameness on a consistent basis; palpable instability less than that was not correlated to patient function. For this reason, the actual complication rate of an IA technique likely cannot be determined without documentation of graft survival. The TransFix® technique demonstrated an excellent outcome on owner questionnaire (close to 100% satisfaction), a good outcome with force plate analysis (similar to slightly better outcomes compared to TPLO results) but a poor outcome with second‐look arthroscopy for IA repair. The authors recommended that IA repairs be assessed by visualization or advanced imaging 6–12 months postoperatively to document survival. Even with these imaging modalities, true health of the graft cannot be assessed and biopsy would likely be needed (not necessarily recommended by the authors due to damage to the graft). If graft failure is detected, the correct course of action depends upon the patient, owner, and surgeon. In our experience, if we palpated femoral‐tibial instability but the dog had limb asymmetry <15% on force platform analysis and the owners had no complaints about limb function, we recommended to continue monitoring with no action taken. Practically, the goal is to resolve pain and lameness; we have not made a recommendation for surgery simply because there was palpable femoral‐tibial translation. In cases where lameness persists and/or infection is detected, complete explantation of all implants and graft is recommended. In our experience, once the graft has failed, the implants were easy to remove as the grafts tend to fail early in the recovery period before healing has occurred. A stifle stabilizing surgery after implant removal depended upon the cause (e.g., infection, lameness, and joint pain with graft failure) and the amount of femoral‐tibial instability. The experience from previous studies provides a small but important insight into IA repair failure in veterinary medicine. First, IA grafts can survive and result in an excellent patient outcome [51, 56, 59]. A remaining question is whether successful results can be obtained consistently. Second, graft survival appears to be higher when a CCL transection model is used compared to naturally occurring CCL disease. Caution should be exercised when determining the success and complications of IA repair when using an induced CCL model. Third, given the limited studies on naturally occurring CCL disease with IA repair, lack of studies using surgical techniques and/or implants that have been fully vetted and inconsistency in study designs, definitive rates and recommendations about complications are not possible. Finally, with IA repair it is important to note that the actual complication rate of a technique likely cannot be determined without documentation of graft survival. For example, an IA allograft repair demonstrated an excellent outcome on owner questionnaire, a good outcome with force plate analysis, and a poor outcome with second‐look arthroscopy [56]. This and many other studies document the importance of objective outcome measures for gauging both success and specific complications. Based on lessons learned, the authors recommend the following when evaluating IA repairs: (i) the initial biomechanical properties of the graft and fixation technique are documented, (ii) follow‐up time of at least 12 months to allow ligamentization, and (iii) graft survival is evaluated by second‐look arthroscopy (or advanced imaging as technology advances). In the following section, the authors use examples to illustrate complications, clinical consequences, and success of revision therapy.
4
Complications Associated with Intraarticular Repair Techniques
4.1 An Introduction to Intraarticular Repair in Veterinary Medicine
4.2 Intraarticular Repair Complications in Humans
4.3 Intraarticular Repair Complications in Canines
4.4 Graft Selection
4.5 Tunnel Creation
4.6 Graft Fixation
4.7 Intraarticular Repair Assessment and Revision
4.8 Conclusion
4.9 Clinical Examples
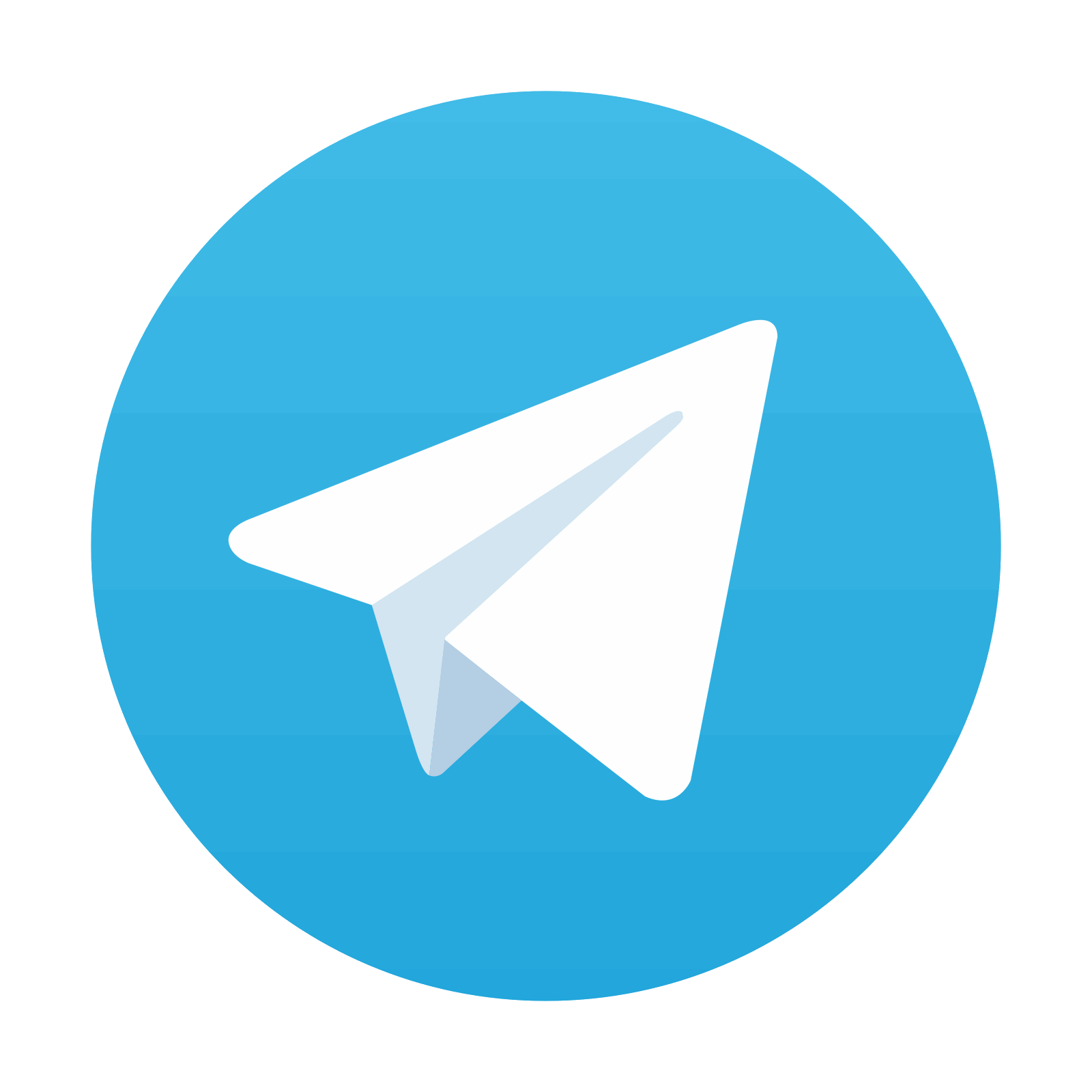
Stay updated, free articles. Join our Telegram channel

Full access? Get Clinical Tree
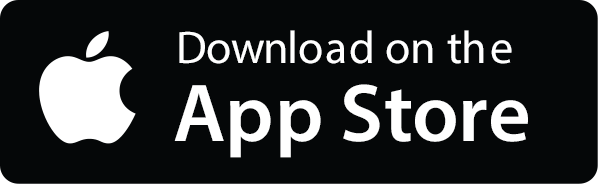
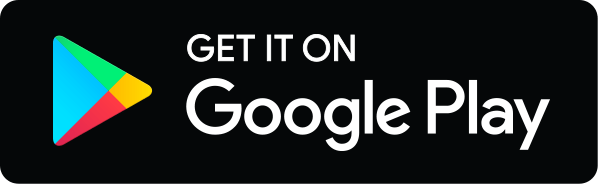