3 Niklas Bergknut, Franck Forterre, Jonathan M. Levine, Steven D. Lasser, and James M. Fingeroth There are many similarities between the canine and human spines and their vertebral components. The vertebral units comprising two adjacent vertebrae—an intervertebral disc and facet joints—are comparable in both species. The human spine averages 33 vertebrae, whereas the canine spine generally averages 50 [1]. Humans generally have 12 thoracic and 5 lumbar vertebrae, whereas dogs generally have 13 thoracic and 7 lumbar vertebrae. Intervertebral disc (IVD) degeneration and herniation are considered the main causes of acute and chronic low back pain in humans [2–6], with a lifetime prevalence of over 70% in the global population [7]. Also, dogs commonly suffer from back pain caused by degenerated IVDs subsequently herniating; it is in fact the most common cause of neurological deficits in dogs [8] and is one of the most common causes for euthanasia in dogs less than 10 years of age [9]. Moreover, the clinical presentation, macroscopic and microscopic appearance, diagnostics, and treatment of IVD degeneration and herniation are similar in humans and dogs [6, 10–12]. Decompressive surgery and spinal fusion are common treatments for IVD disease (IVDD) in both humans and dogs, with fusion less often used in the latter species. The many similarities between canine and human spines and their diseases have led to dogs being frequently used for translational studies of surgical procedures and biomechanical studies of the spine [13–18]; and recently, the dog has been proposed as a translational model for acute spinal cord injury (SCI) [19] as well as for IVD degeneration [20]. The occurrence of asymptomatic degenerated IVDs is common in both dogs and humans [21, 22]; and in general, similar pathological changes have been reported in degenerated IVDs from humans and dogs [23]. A notable difference between human and canine vertebrae is the absence of growth plates in growing human vertebrae and the thicker cartilaginous end plates in humans. Whereas in dogs most vertebral growth takes place in the growth plates, in humans, vertebral growth takes place in the junction between the vertebrae and the end plates, which may explain the thicker end plates found in humans [20, 24]. The overall appearance of both healthy and degenerating IVDs from humans and dogs has been found to be similar. All five stages of degeneration described in human IVDs by Thompson et al. have also been described in dogs [23]. The healthy IVDs of both species comprise (1) a fibrocartilaginous annulus fibrosus (AF) consisting of well-organized lamellar layers, (2) a soft gelatinous nucleus pulposus (NP), and (3) cartilaginous end plates lining the vertebral end plates. In the healthy IVDs, these structures are clearly distinguishable from each other; but once degeneration starts, the borders between these three structures are progressively more difficult to discern. Disorganization of the tissue structure, mainly with the AF showing increasing numbers of clefts and cracks, becomes visible with increasing degree of degeneration. In the later stages of degeneration of discs in both humans and dogs, a marked loss of IVD space can be noted, with new bone formation (spondylosis deformans) apparent, especially, on the ventral/anterior aspect of the intervertebral segments [20]. Although canine IVDs are generally smaller than human IVDs, the ratio of NP area/IVD area is reported to be similar in the two species. However, the ratios of IVD height/width, NP/IVD width, and end plate thickness/IVD height are reported to significantly differ between healthy human and healthy canine IVDs [20]. As in humans, the height of the canine lumbosacral IVD is reported to be greater than that of the lumbar IVDs (Table 3.1) [20]. Table 3.1 Measurements and dimensions of the midsagittal surface of canine and human lumbar intervertebral discs (IVDs) as determined by gross and histopathological examinations Published with permission from Bergknut et al. [20]. The P-values reflect significant differences between the values obtained in dogs and humans. EP, end plate; N/A, not available; NP, nucleus pulposus; SD, standard deviation. a Measurements obtained from the literature [25–27]. * Statistically significant difference between dogs and humans at P < 0.01. Contrary to the well-innervated human IVDs [28], the innervation of the canine IVD tissue itself is sparse: nerve endings have only been found in the outer lamellae of the AF and not in the NP, the transitional zone, or the inner AF [29–31]. However, soft tissue structures surrounding the canine IVDs, such as the dorsal longitudinal ligament, are profusely innervated [29, 30]. The canine spinal column is so constructed as to make the vertebrae able to resist powerful compressive forces. According to Wolff’s law, the trabecular structure of the cortical bone adapts to strengthen the bone to cope with compressive forces. By following this principle, the quadruped spine appears to be loaded mainly by axial compression in a way that is similar to the bipedal human spine [32]. Dogs and other quadrupeds have higher vertebral bone densities than humans, indicating that axial compression stress is greater in dogs than in humans [33]. The other mechanical loads are transformed by the surrounding musculature into axial compression, and eventually facet joint loads. The size of the vertebral canal is much larger in humans than in dogs. However, in both humans and dogs, the areas with the largest vertebral canal diameter (lumbar and cervical regions) are also the most mobile ones [1]. The human vertebral canal is more triangular in its vertical dimension, whereas a more oval shape in the horizontal dimension is observed in dogs. The spinal cord fills about 80% of the vertebral canal in dogs. The vertebral facet joints have two functions: the first is to enable but also to limit the segmental range of motion, and the second is to absorb the energy that results from the ventrally directed shearing forces [34]. The joints are therefore somewhat differently oriented at different levels of the spinal column. Comparing the orientation of the human articular processes with that in dogs, it becomes obvious that these processes show a high degree of adaptation to the movement patterns of each species [35]. In humans, articular surfaces have an angle of more than 60° to the frontal plane, while in quadrupeds, it is less than 30° [36]. Further, the cancellous bone of the most lateral extended parts of the cranial articular processes possesses a characteristic architecture that can be recognized as an adaptation to bending [37]. Together with ligaments, the facet joints determine the direction and degree of displacement of the vertebrae one to another. Because of their intrinsic fiber orientation, the AF, the dorsal longitudinal, interspinal, and supraspinous ligaments contribute to kinematic control, while the ventral longitudinal ligament has a very important static function [38]. Further, the lumbar ligaments, together with their vertebral joints, behave like a gear system without a constant center of rotation. As an adaptive consequence, the thickness of the ventral and dorsal parts of the AF is distributed unequally, and rotation is limited by the facet joints in conjunction with the thicker ventral part of the AF. The facet joints thus play an important stabilizing role in jumping and running [32, 35]. The canine IVDs represent about 20% of the length of the spine, while in humans, the discs represent 25% [1]. It was generally recognized that the two main functions of the IVD are the absorption of shock to the spine and the provision of flexibility to the spinal column [39]. The consistent arrangement of the trabecular bone at right angles to the dorsal/posterior and ventral/anterior surfaces of the vertebral body makes it obvious that these surfaces, under normal conditions, take on an undeviating and equally distributed axial pressure. The IVD acts not as much as a shock absorber per se but as a kind of water-filled cushion that mediates the equal distribution of pressure [35, 40]. If its hydrostatic function is impaired as a result of the loss of water, this cushioning effect is lost as well. A second disc function is related to the associated ligamentous apparatus. The aforementioned gear mechanism only works optimally when as many as possible of the ligaments and fiber bundles are under pretension. The swelling pressure of the nucleus pulposus helps maintain the separation of the contiguous vertebrae and thus creates the necessary tension for the ligamentous apparatus to work effectively [35]. The most important clinical/anatomical difference between both species is the relationship between the length of the vertebral column and the spinal cord. Usually in dogs, the spinal cord terminates between the two last lumbar vertebrae (L6–L7), while in humans, it terminates between the first two lumbar vertebrae (L1–L2). Therefore, the usual disc herniation sites in the terminal lumbar area (L3–L4, L4–L5, L5–S1) in humans result in only nerve roots of the cauda equina being affected, while the usual thoracolumbar or lumbar localization of disc herniations in dogs will directly compress the spinal cord. The spine, whether in quadrupeds or bipeds, can be regarded mechanically as a series of freely hinged vertebrae needing further support from surrounding tensile structures to control posture. During the different forms of locomotion, axial torsion, flexion–extension, and lateral bending moments are important loads that act on the spine. Tensile structures such as muscles and ligaments must be active to compensate for the applied moments, leaving the spine under considerable axial compression [32]. In the healthy IVDs from both dogs and humans, there is a clear distinction between the cell populations and the organization of the cells in the AF and NP. In the NP of healthy IVDs in dogs under 1 year of age, and in children, notochordal cells are the predominant cell type. These large, vacuolar cells clump together in large clusters in a similar unorganized fashion in both species. Notochordal cells are also common in the NP of adult nonchondrodystrophoid dogs but not in the IVDs of adult humans or chondrodystrophoid dogs, where chondrocyte-like cells are most common [30, 41]. The cells in the healthy AF of both dogs and humans are smaller fibrocyte-like cells, located in between well-organized lamellar layers of collagen fibers.
Comparisons between Biped (Human) and Quadruped (Canine/Feline) Intervertebral Disc Disease
Anatomy and gross pathology
Measurement
Dog
Human
P-value
Mean ± SD
Range
Mean ± SD
Range
Gross pathology
IVD height (mm)
3.5 ± 0.6
2.4–4.7
10a
6–14a
N/A
IVD width (mm)
15.9 ± 2.0
11.0–18.9
35a
27–45a
N/A
Ratio of IVD height/width
0.22 ± 0.03
0.14–0.28
0.29 ± 0.05
0.23–0.34
<0.01*
Ratio of NP/IVD width
0.30 ± 0.05
0.21–0.41
0.38 ± 0.05
0.31–0.44
<0.01*
Ratio of NP/IVD area
0.25 ± 0.05
0.17–0.34
0.28 ± 0.02
0.24–0.31
0.18
Histology
EP thickness (mm)
0.22 ± 0.06
0.1–0.42
1.58 ± 0.35
1.25–2.51
<0.01*
EP thickness/IVD height (%)
6
3–11
13
9–19
<0.01*
Number of cell layers in EP
5
3–8
21
18–23
<0.01*
Cortical thickness (mm)
0.90 ± 0.36
0.27–1.78
0.66 ± 0.33
0.25–1.59
0.06
Biomechanics
Histology and histopathology
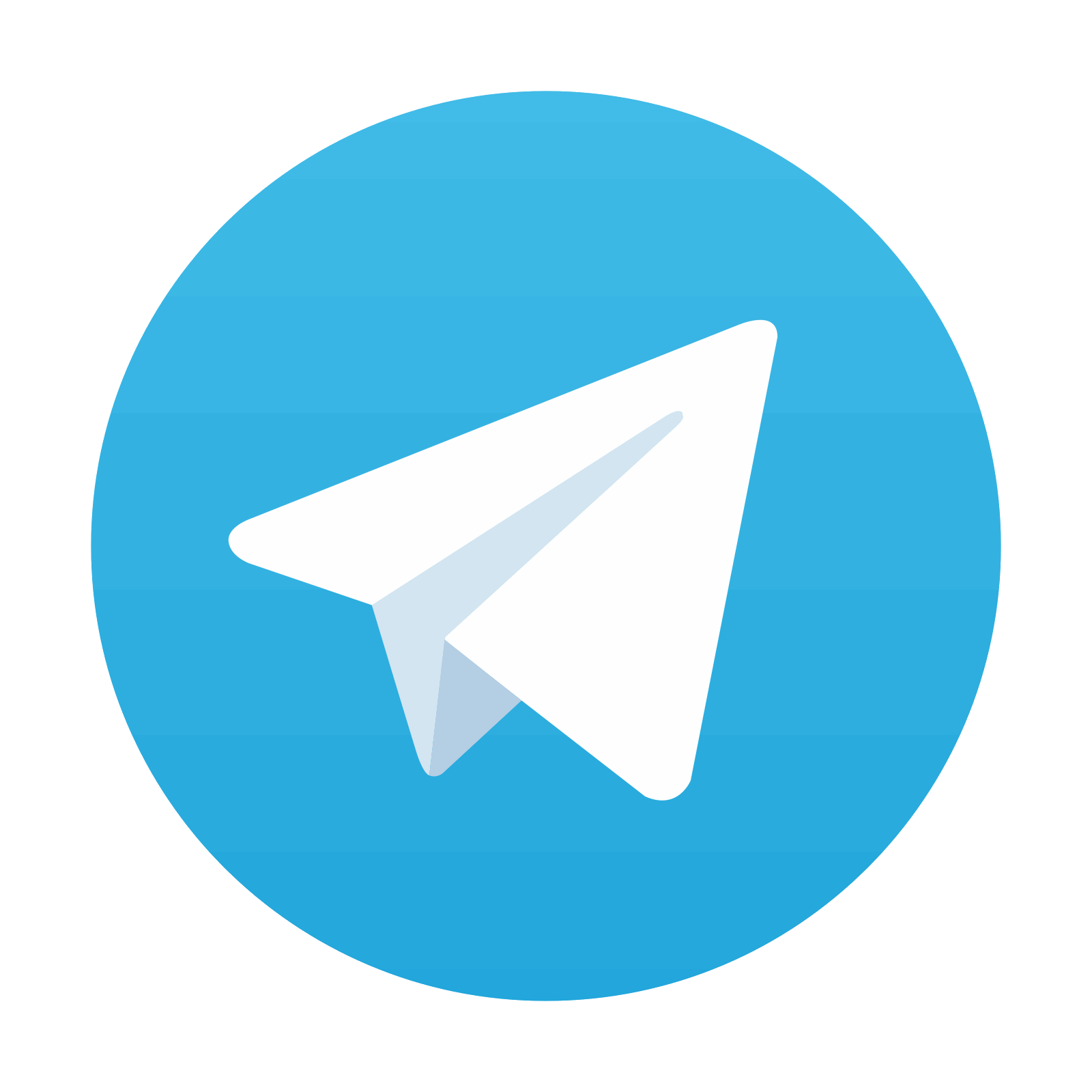
Stay updated, free articles. Join our Telegram channel

Full access? Get Clinical Tree
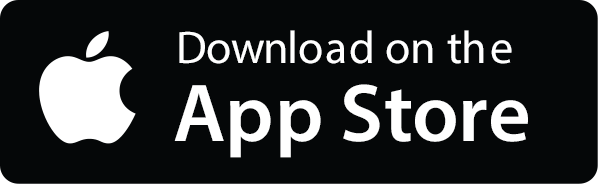
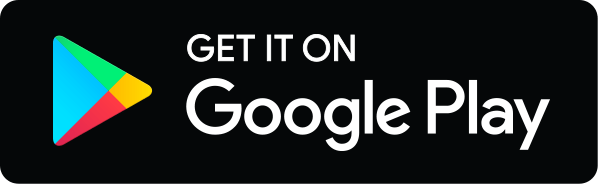