Fig. 8.1
Model of the elephant ovarian cycle, showing relationships among the secretion of estradiol, progestagens, LH, FSH, prolactin and inhibin throughout a follicular phase preceding and following a luteal phase. Schematic shows relationships among the two follicular waves that each culminate in an LH surge (first surge, LH1; second surge, LH2), and the development of follicles and corpora lutea (CL), both ovulatory (ovCL) and accessory (acCL). Prolactin is presented for African elephants only, as it is not influenced by stage of the cycle in Asian elephants. Adapted from Brown (2000), Hildebrandt et al. (2006) and Lueders et al. (2010, 2011)
Elephants are not obligatory seasonal breeders, but conceptions can be influenced by rainfall and resource availability, as noted in African elephants (Sukumar 2003). Observations of elephants in zoos support a seasonal trend under some conditions; for example, a group of African elephants housed indoors because of extreme cold weather showed prolonged non-luteal phases before re-initiating normal ovarian cycles in the spring (Schulte et al. 2000). Seasonal effects on Asian elephant reproduction, if any, are more subtle. Glaeser et al. (2012) found no seasonality in ovarian cycle lengths in a study of nine females over a 20-year period, whereas a detailed study of elephants in Thailand showed females cycled year round, but exhibited slightly longer follicular phases during the rainy season (Thitaram et al. 2008). Specifically, it was the duration between the progestagens drop and LH1 that varied seasonally, averaging 33 days during the rainy season, compared to 22 days in the winter and 19 days during the summer seasons. By contrast, the interval between LH1 and LH2 was consistent at 19 days, similar to other studies. Thus, seasonal variation in estrous cycle length may be mediated by events during the period leading up to LH1 (Thitaram et al. 2008), perhaps due to more variation in the completion of the first follicular wave. Variability in duration of the follicular phase also tends to be greater than that of the luteal phase, at least in Asian elephants (Thitaram et al. 2008; Glaeser et al. 2012). In those two studies, luteal and follicular phase durations were negatively correlated, suggesting a possible regulatory role of the follicular phase in maintaining relatively consistent cycle duration within individuals. In the study of Glaeser et al. (2012), Asian elephants were highly resilient to numerous major life events (births, deaths, transfers in and out, changes in herd structure), which had a minimal effect on cycle dynamics. That Asian elephants maintain consistent cycles despite a variety of management changes agrees with about 20 years of unpublished data on over 60 Asian females evaluated at SCBI (J. Brown). By contrast, data on over 80 African females monitored at SCBI provide numerous examples of events related to temporary or permanent suppression of estrous cyclicity (e.g., translocations, changing blood collection frequency, altered herd dynamics, keeper changes). This suggests that Asian and African elephants may differ in responsiveness to changes in the captive environment, something that deserves further investigation in the context of optimizing conditions based on species-specific needs.
An elegant series of studies (Lueders et al. 2010, 2011) combined transrectal ovarian ultrasound and hormone measurements in Asian elephants to develop a novel theory for the double LH surge in relation to dominant follicle selection and luteal development. One enigma has been the observation of multiple corpora lutea (CL) during each cycle despite elephants being monovular. During the follicular phase, two distinct waves of follicles develop, each of which is terminated by an LH surge. During the first wave, multiple follicles develop, but none ovulate. Originally it was believed these all regress after LH1 (Hermes et al. 2000); however, serial transrectal ultrasound examinations revealed that some of these follicles do in fact luteinize, and are referred to as accessory CL (acCL) (Lueders et al. 2012). During the second wave, multiple follicles develop, but only one becomes dominant, ovulates and forms the ovulatory CL (ovCL). Thus, there appears to be two modalities for the development of acCL and ovCL. Follicle luteinization is apparent within 10 days of each LH surge (Lueders et al. 2010), but the acCLs remain dormant and do not secrete progestagens, possibly due to lack of 3β hydroxysteroid dehydrogenase activity in the lutein cells (African: Stansfield and Allen 2012). After ovulation, the acCLs reach a maximum diameter within 30 days, whereas the single ovCL attains a significantly larger size 10–15 days later (Lueders et al. 2010). All CLs are visible throughout the follicular phase, with some of the larger ones remaining in subsequent luteal periods. By repeatedly forming two distinct types of CLs during every reproductive cycle, elephants may have developed a mechanism to ensure there is sufficient luteal capacity for maintaining a 22-month gestation should conception occur, at least in Asians (Lueders et al. 2010).
Simultaneous with luteinized follicle formation after LH1, immunoreactive (ir) inhibin concentrations increase, preceding progestagens by about 2 weeks in both species (Brown et al. 1991; Kaewmanee et al. 2011a; Lueders et al. 2011; Yamamoto et al. 2012a) (Fig. 8.1). Immunohistochemistry has shown that inhibin α and β subunits are present in granulosa cells of antral follicles in Asian elephants, as are high concentrations of immunoreactive and bioactive inhibin in the follicular fluid (Kaewmanee et al. 2011a), similar to other species (Medan et al. 2007; see Kaewmanee et al. 2011a). However, in the study of Lueders et al. (2011), ir‐inhibin never increased before LHI, even though large follicles were present and attained diameters within the range of ovulatory follicles. In other species, both small and large estrogenic follicles are a significant source of ir-inhibin (Campbell et al. 1991), so if inhibin is derived solely from follicles in elephants, there should be measurable levels prior to LH1 because estrogens are detectable before both LH peaks (Czekala et al. 2003). It is puzzling then, that ir‐inhibin increases only after follicle luteinization post LH1, about 9 days before LH2 (Lueders et al. 2011; Kaewmanee et al. 2011a). The investigators theorize that rather than granulosa cells, it is the cells of luteinized follicles and acCLs that are the source of inhibin (Lueders et al. 2011). The absence of luteinized follicles before LH1 would explain why inhibin concentrations are low, and also why there is a significant correlation between luteinized follicle/acCL diameter and increasing ir‐inhibin concentrations. Dominant follicle deviation begins about 5–6 days before ovulation when inhibin concentrations are high. From these observations, authors further propose that inhibin itself may be important for dominant follicle selection. Although inhibin was not measured, a study investigating the follicular response to GnRH would appear to support this concept (Thitaram et al. 2009). When administered at different times during the follicular phase, GnRH always stimulated LH release (i.e., LH1); however, a spontaneous secondary surge ~20 days later (i.e. LH2) only occurred if LH1 was induced between days 13–42 of the follicular phase. By contrast, no LH2 occurred when GnRH was administered before day 12. So, if LH1 is induced too early, follicles are not mature enough to luteinize and produce inhibin. As a result, there is no dominant follicle selection during the second follicular wave. Alternatively, rather than directly affecting follicle selection, increased inhibin concentrations may simply indicate that follicles have reached a level of maturity that permits deviation and subsequent ovulation.
In all likelihood, inhibin’s role in follicle selection is through its control over FSH secretion. In both species, the FSH secretory pattern is protracted and inversely related to inhibin (Brown et al. 1991; Brown et al 1999; Kaewmanee et al. 2011a) (Fig. 8.1). FSH is highest towards the end of the luteal phase and throughout the first follicular wave when inhibin is low, then decreases to nadir concentrations before LH2 as inhibin is rising. Comparatively, the FSH pattern in elephants differs somewhat from other mammals, where concentrations typically are elevated coincident with the pre-ovulatory LH surge, with a secondary FSH surge sometimes occurring after ovulation (Downey 1980). Instead, FSH secretion in the elephant is more like that of the horse, where concentrations are highest at the end of the luteal phase and decrease progressively towards ovulation (Ginther 1992). However, the follicular phase in other mammals, including horses, is considerably shorter (<1 week) than that in the elephant, so these comparisons may not be relevant. Nevertheless, because FSH plays a key role in follicle recruitment and growth in other species, a prolonged stimulation may be necessary for two successive follicular waves to occur in the elephant. As in other species, the reduction in FSH likely facilitates dominant follicle deviation, with the transition of follicles from an FSH-dependent to an independent state being key to ovulatory selection (Baird 1983). FSH concentrations are low after ovulation and then rise during the latter part of the luteal phase, just behind the decline in inhibin (Brown et al. 1991; Kaewmanee et al. 2011a). This inhibin is of luteal origin, as no antral follicles are present post-ovulation (African: Hermes et al. 2000; Asian: Lueders et al. 2010), and immunohistochemical staining has localized inhibin α and β subunits to the lutein cells of CLs, similar to that observed in primates (Yamoto et al. 1991, 1992).
Prolactin is folliculogenic in several species (Freeman et al. 2000; Frasor and Gibori 2003), and in African elephants is elevated during the nonluteal phase of the cycle, inversely related to progestagens, and phase-shifted by about 4 weeks (Yamamoto et al. 2010; Dow and Brown 2012) (Fig. 8.1). However, in Asian elephants, prolactin concentrations are unvaried throughout the cycle and remain at baseline concentrations, representing a major species difference (Brown et al. 2004a). As discussed below, it may be significant that ovarian cycle problems associated with abnormal prolactin secretion are common in African, but not Asian elephants (Dow and Brown 2012).
2.2 Pregnancy and Parturition
Once again, transrectal ultrasound and endocrine monitoring have been key to better understanding the physiology of pregnancy in elephants, which have the longest gestation period, lasting 20–23 months on average. The placenta is chorioallantoic, zonary endothelialchorial; implantation is central and superficial, with a mesometrical orientation of the yolk sac; the embryo is antimesometrial in location (see review, Allen 2006; Hildebrandt et al. 2006). Although the placenta itself is endocrinologically inert, the fetal gonads, which enlarge during the second half of gestation, synthesize 5α-dihydroprogesterone and other 5α-pregnane derivatives from cholesterol and pregnenolone (Allen et al. 2005; Allen 2006; Stansfield and Allen 2012). Placentation occurs during the second to third month of gestation (Drews et al. 2008). Based on longitudinal transrectal ultrasound monitoring, both species exhibit an initial period of comparatively slow embryonic development that has been compared to delayed implantation (Hildebrandt et al. 2006; Drews et al. 2008). The embryonic vesicle is visible at ~8 weeks post-conception, which is much smaller (~10 mm) than that of other species at that stage (cattle, 40 mm; sheep, 70 mm; horse, 40 mm). Likewise, time of implantation is estimated to be ≤20 days in human, dog and sheep, but ≥50 days in the elephant. In other delayed implanters (e.g., mustelids, bears, roe deer), a significant rise in serum progestagens occurs at implantation, which is observed in elephants at 6–8 weeks post-conception (Meyer et al. 2004). The embryo then doubles in size between the fourth and fifth month, increasing from 60 mm to 120 mm (Drews et al. 2008). Organogenesis is completed by about 110–120 days, when the end of the embryonic period is reached. It is possible to sex the fetus after about a year of gestation with near 100 % accuracy by measuring circulating maternal testosterone concentrations, at least in Asian elephants (Duer et al. 2002; Brown et al. 2004b). Presumably elevated testosterone is of fetal testicular origin, although the CL could also be a source (Castracane et al 1998). Interestingly, this technique has proven less accurate for African elephants (J. Brown, unpubl).
A model for the endocrinology of pregnancy is depicted in Fig. 8.2. Diagnosis and monitoring of pregnancy is easily done by longitudinal analysis of 5α-reduced pregnanes in the bloodstream or the relevant metabolites in urine or feces (see reviews, Brown 2000; Hildebrandt et al. 2006). Elephants and horses share some gestational traits, such as the presence of multiple large CL in the maternal ovaries (reviewed by Stansfield and Allen 2012). However, there are notable differences. In the elephant, acCLs are produced throughout the follicular phase of preceding cycles (Lueders et al. 2010, 2011), and there are no additional CLs produced during gestation (Lueders et al. 2012). By contrast, the mare produces one CL at ovulation, with additional CLs formed as the result of ovulations after conception (Squires and Ginther 1975). Gross examination and histology of African elephant ovaries indicates acCL form by luteinization of follicles, with or without ovulation; stigmata are clearly visible on some pregnancy CLs (Stansfield and Allen 2012). By contrast, ultrasound examinations of pregnant Asian elephants suggest acCLs are the result of luteinization of unruptured follicles only (Lueders et al. 2010, 2011). It is not clear if this is a species difference. In both Asian and African elephants, acCLs and the ovCL begin to regress about 5–6 weeks after conception (the normal luteal phase lifespan), but then rebound and grow significantly larger than those in a non-conceptive luteal phase (Lueders et al. 2012), commensurate with the marked secondary rise in progestagen concentrations in the maternal circulation after the second gestational month (see Meyer et al. 2004; Lueders et al. 2012). In the pregnant mare, acCLs develop equally on both ovaries due to the LH-like activity of equine chorionic gonadotropin (eCG), which causes ovulation/luteinization of mature Graafian follicles (Urwin and Allen 1982). By contrast, mature follicles never develop in pregnant elephants (see Lueders et al. 2012), nor is there evidence of gestational gonadotropin-like activity in serum or placental extracts (Meyer et al. 2004; Allen 2006).
After 4–7 months of gestation, prolactin immunoactivity (ir-prolactin) increases up to 100-fold, peaks at 11–14 months and remains high until birth in both species (Brown and Lehnhardt 1995; Meyer et al. 2004; Yamamoto et al. 2011, 2012b). Prolactin and placental lactogens are luteotrophic in other species, and enhance CL progestagen production (Freeman et al. 2000; see Takahashi 2006). This would be important for elephants because the placenta is steroidogenically inactive (African: Allen et al. 2002). The source of high ir-prolactin during gestation is primarily placental (Yamamoto et al. 2011), similar to lactogenic hormones in other species (Forsyth and Wallis 2002). Whereas placental lactogens are derived from the desidua in humans and rats (Ben-Jonathan et al. 2008), ir-prolactin in elephants is immunolocalized in the trophoblast cells of both species (Yamamoto et al. 2011). Measurement of serum ir-prolactin past 7 months of gestation is a reliable pregnancy test, even on a single sample, unlike progestagens, which require longitudinal sampling. Unfortunately, ir-prolactin has not been detected in urine, so noninvasive pregnancy diagnosis by endocrine means has so far not been feasible (Brown et al. 2010). Serum relaxin also can be used diagnostically in both species (Meyer et al. 2004; Niemuller et al. 1998), as concentrations are elevated after 5 months of gestation. Levels peak at about 10 months and then gradually decline until a few weeks before birth, when a sharp rise occurs just before parturition. The CL of pregnancy is a main source of relaxin in many species, but in others the decidua also produces considerable amounts (MacLennan 1981). The source of relaxin in elephants is not known, but it may play a role in parturition similar to that in other species by facilitating a softening of the cervix and loosening of pelvic ligaments, and ensuring synchrony in uterine muscles after labor begins (MacLennan 1981).
In a large comparative study, there was a broad range of individual variation in gestation length (Asian, 623–729 days; African, 640–673 days) (Meyer et al. 2004). That study also identified several notable species differences in gestational hormone patterns. While overall mean progestagen concentrations were similar, temporal profiles differed. Concentrations were higher in African elephants during the first half of gestation, but then declined to levels below those observed in Asian elephants (Fig. 8.2). There was a fetal gender effect in Asian, but not African elephants, with progestagen concentrations being higher in Asian cows carrying male calves as compared to those carrying females. It is curious that significant fetal gender differences in maternal steroids (androgens and progestagens) are observed only in Asian elephants, suggesting a species difference in gonadal and/or placental function. Both species have zonary placentation; however, far more information is available on African elephant placental function, and detailed comparative studies at the level needed to identify species differences in steroidogenic activity have not been conducted. A fetal sex difference in progestagens may also be related to testicular steroid production. During sexual differentiation, progesterone produced by fetal Leydig cells is converted to testosterone to complete male duct system development (Lejeune et al. 1998), but why this would occur in Asian, but not African elephants is not known. Comparatively, overall prolactin concentrations were higher in Asian than in African elephants between 8 and 15 months of gestation, but there was no species difference in the secretory patterns of relaxin (Meyer et al. 2004). In both species, the observation of significant surges in serum cortisol between 8 and 11 days before parturition, and again on the day of parturition (Meyer et al. 2004), suggests an important role in the initiation of parturition (Liggins and Thornburn 1993).
In many species, particularly primates, inhibin produced by follicles, CLs and/or the placenta (Knight 1996), is believed to be involved in the establishment and maintenance of pregnancy (Florio et al. 2010). In the elephant, whereas CLs are a major source of inhibin during the estrous cycle (Kaewmanee et al. 2011a; Yamamoto et al. 2012a), they do not appear to produce inhibin during gestation, as overall concentrations are low (Yamamoto et al. 2012a) (Fig. 8.2). On closer inspection, inhibin in fact is increased for the first 8 weeks post-conception, mimicking a normal luteal phase increase. Thus, both progestagens and inhibin are increased immediately post-conception and then decrease at 7–8 weeks. After that, progestagens rebound to even higher concentrations on average, whereas inhibin concentrations continue to decline. This pattern suggests a shift in luteal cell function during early gestation, and a deviation in the secretory ability of CLs between cycling and pregnant elephants. Taken together, a role for inhibin in elephant pregnancy seems unlikely. A related protein, activin A, may be worth investigating as it is secreted by stromal endometrial cells and is involved in implantation in other species (reviewed by Florio et al. 2010). It also enhances cytotrophoblast differentiation indirectly by increasing the expression of other molecules involved in embryo implantation, such as matrix metalloproteinases and leukemia inhibitory factor. A local derangement of the activin A pathway has been implicated in some human pregnancy disorders (incomplete and complete miscarriages, recurrent abortion, and ectopic pregnancy), and so may be worth investigating in older elephants that are more susceptible to poor pregnancy outcomes.
2.3 Reproductive Challenges
Transrectal ultrasonography techniques have become instrumental in monitoring reproductive tract health, including in elephants (Hildebrandt et al. 2003). In the U.S., nearly half of Asian and African elephant females in AZA-accredited zoos have had an ultrasound examination (Dow et al. 2011a). Of these, the majority exhibit one or more reproductive tract pathologies of ovarian or uterine origin. As reviewed by Hildebrandt et al. (2006), vestibular cysts occur in both species, whereas vestibular polyps are observed only in Africans, with an incidence of about 70 % in females >30 years of age. In both species, vaginal cysts and neoplastic formations may be extensive and fill the vaginal lumen, blocking semen flow after mating and causing discomfort during estrus and mating. Periodic vaginal discharge containing mucus and clotted blood is a symptom of this condition. Asian and African elephants both develop endometrial hyperplasia, whereas Asian elephants develop multiple benign uterine leiomyomas. Ovarian cysts also occur more frequently in zoo African (~15 %) than Asian (~5 %) elephants compared to wild females (<1 %). In general, reproductive tract pathologies are more prevalant in older (>30 years of age) nulliparous cows, and those where reproduction has not occurred within 10–15 years. In a recent survey, more than half of zoo females with documented tract pathologies had no previous breeding history, either through natural mating or AI (Dow et al. 2011a). The occurrence of urogenital pathologies in older females is termed ‘asymmetric reproductive aging’ (Hermes et al. 2004), and believed to be the result of continuous ovarian cyclicity of non-bred females. Repetitive remodelling and exposure of the endometrium to ovarian steriods likely has a negative and cumulative effect on reproductive health (Hermes et al. 2008). In the wild, most females are either pregnant or lactating and thus experience comparatively few reproductive cycles in their lifetime. Consequently, these pathologies are not common in wild elephants (Hildebrandt et al. 2006; Freeman et al. 2008).
As Asian and African elephants in zoos age, the risk of developing pathologies increases (Aupperle et al. 2008), so a new treatment to slow or stop their development based on the use of GnRH vaccines is being explored (Boedeker et al. 2012). These vaccines stimulate the production of anti-GnRH antibodies that block the binding of endogenous GnRH to gonadotrope receptors in the pituitary gland (Conforti et al. 2008). This action inhibits the release of FSH and LH from the anterior pituitary, thereby causing the cessation of ovarian steroidogenic activity and reproductive cyclicity. In one case study, a CpG motif-based adjuvant in a recombinant GnRH vaccine (Repro-BLOC, Amplicon Vaccine, LLC, Pullman, WA) suppressed ovarian cycle activity and resolved hemorrhage and anemia associated with a vascular reproductive tract tumor in a 59-year-old Asian elephant (Boedeker et al. 2012). Synthetic oligodeoxynucleotides (ODNs) containing unmethylated CpG motifs directly stimulate B cells and plasmacytoid dendritic cells, thereby promoting the production of cytokines and the maturation/activation of antigen-presenting cells (Klinman 2006). These activities enable CpG ODNs to act as immune adjuvants, accelerating and boosting antigen-specific immune responses by 5- to 500-fold over traditional Freund’s adjuvants. Six years after initial vaccination, the elephant continues to lack distinct ovarian cycles and is healthy. In addition to resolving these problems in older females, there is interest in using GnRH vaccines as contraceptives for wild elephants to modulate population growth and mitigate human-elephant conflict and protect resources in limited habitats (Bertschinger et al. 2007; Botha et al. 2008).
Of considerable concern is the tendency for older, first-time mothers to experience dystocia and/or stillbirths. Over 50 % of dystocias occur in nulliparous females >20 years of age or after prolonged barren periods (Hermes et al. 2008). As a management recommendation, nulliparous females in captivity are considered post-reproductive after 25–35 years of age because of this increased risk (Hermes et al. 2004; Hildebrandt et al. 2006); over two thirds of Asian elephants in U.S. zoos are now above this age. Causes of calving problems include: large calves; malposition or an anterior position; loss of flexibility in the pelvic region; muscle fatigue; hypocalcemia; intact hymen obstructing calf passage; edema of the vestibule narrowing the birth canal; elephant endotheliotropic herpes virus infection; and cysts, polyps and/or tumors of the urogenital tract (Hermes et al. 2008). Many of these can be linked not only to the female being older, but to being overweight and lacking in physical fitness. To date, caesarean section has not been successful in resolving dystocia in elephants, although several have been tried (Hermes et al. 2008). The only surgical option to correct a dystocia is vestibulotomy and if necessary a subsequent fetotomy (Schaftenaar 2013). However, these are only possible if fetal parts have already entered the cervix. These problems make tracking gestation progress with daily progestagen analysis critical to ensure staff is prepared to take proactive steps if needed (e.g., oxytocin to enhance labor after opening of the birth canal has been confirmed by ultrasound; Brown et al. 2004b; Hermes et al. 2008). It is now clear that prolonged non-reproductive periods in elephants are associated with serious reproductive issues, making it imperative to breed females soon after puberty and regularly throughout their reproductive lifespan to avoid compromising health and well-being.
2.4 Ovarian Acyclicity
Factors limiting the number of breeding female elephants vary by species. For Asian elephants, the main issue is advancing age. For African elephants, a primary cause of poor reproduction is a high rate of ovarian acyclicity. Based on a 2008 reproductive survey of elephants in AZA-accredited zoos, 46 % of African elephant females exhibited abnormal ovarian cycles, and 31 % did not cycle at all. Most importantly, the majority of ovarian cycle problems occurred in reproductive age females (Dow et al. 2011a). By contrast, only 11 % of Asian elephants were acyclic, and these were mostly post-reproductive females (>40 years of age).
Despite the recognition of this problem in African elephants for over two decades, the etiology of ovarian acyclicity remains a mystery. There is considerably more known about what does not cause it than what does. Several conditions known to be associated with infertility in other species have been examined, such as ‘stress’ (e.g., increased cortisol; Brown et al. 2004a), hyperandrogenism (Mouttham et al. 2011), hyperestrogenism (Prado-Oviedo et al. 2013) and thyroid dysfunction (Brown et al. 2004a), but none were found to be related to ovarian cycle problems. Similarly, concentrations of LH, FSH (Brown et al. 2004a) and inhibin (J. Brown and J. Hoffman, unpubl) are within normal baseline ranges in acyclic females, although none fluctuate as in cycling elephants. One theory was that acyclic zoo elephants may exhaust their supply of ovarian follicles; i.e., undergoing a premature ‘menopause’, due to constant cycling. In most mammals, the ovarian reserve is high at birth and undergoes a steady loss through natural attrition and ovulation during pre- and post-pubertal life (Gosden 1987). Ultimately, the reserves become depleted and reproductive senescence ensues in individuals that reach a maximum lifespan (Cohen 2004). In elephants too, ovarian histology of females culled in southern Africa revealed a significant decline in follicle reserves with age, which in a few individuals were depleted by the 7th decade (African: Stansfield et al. 2012). However, this is well beyond the normal lifespan, so the authors concluded that the elephant ovary is capable of supplying oocytes for ovulation right up to the time of death in most individuals. Still, there are questions about whether the continuous cyclicity of non-bred zoo elephants might accelerate this depletion. Thus, a study was conducted to quantify anti-müllerian hormone (AMH) (Dow et al. 2011b), which is produced by granulosa cells and serves as a marker for the number of morphologically healthy oocytes within the follicular reserve (Rico et al. 2009; Grynnerup et al. 2012). It is used clinically to confirm the state of menopause in women. Results showed that AMH concentrations were not different between cycling and noncycling elephants (Dow et al. 2011b), which can be viewed as good news, as it suggests the ovaries should be responsive to follicular and ovulation induction therapies (e.g., LH, FSH, eCG, hCG). Then again, without detailed histological studies of zoo elephant ovaries similar to those of wild elephants, it will be difficult to eliminate primary hypogonadism as a cause of ovarian acyclicity, at least in some individuals.
Of particular concern is the association between ovarian cycle problems in African elephants (but not Asians) and a hormonal imbalance – hyperprolactinemia (Brown et al. 2004a; Yamamoto et al. 2010; Dow and Brown 2012). In a comprehensive endocrine study (Brown et al. 2004a), a third of noncycling African elephant females had elevated concentrations of prolactin compared to cycling females. In a follow-up study 8 years later, 71 % of acyclic African elephant females were diagnosed with this condition, 45 % of which were of reproductive age (Dow and Brown 2012). This increase was due primarily to elephants switching from a normal to a hyperprolactinemic condition between studies, and so it appears to be a growing problem. Prolactin is produced in lactotroph cells in the anterior pitutary and is under inhibitory control by dopamine (Schuff et al 2002; Melmed 2003; Bachelot and Binart 2007). A common cause of hyperprolactinemia in women is a prolactinoma, the most common type of pituitary tumor (Melmed 2003). Prolactinomas retain intact trophic control, so they may develop in an environment with reduced dopamine concentrations, reduced dopamine sensitivity, or as a result of vasculature isolation that prevents dopamine from reaching the lactotrophs (Schuff et al 2002; Melmed 2003). There are no data on the incidence of prolactin-secreting tumors in African elephants, as pituitary histopathology is rarely performed at necropsy; however this could be a possibility and so should be explored. In other species, hyperprolactinemia is associated with infertility (Aron et al. 1985; Yuen 1992; Zacur 1999), and in women it is the most common disorder of the hypothalamic-pituitary axis; up to 40 % presenting with secondary amenorrhea are hyperprolactinemic (Serri et al. 2003; Wang et al. 2012). The negative effects of chronic elevated prolactin secretion on reproductive function generally involve inhibition of hypothalamic GnRH release and subsequent suppression of pituitary LH and FSH secretion, resulting in anovulation (Bachelot and Binart 2007). Whether that is the case for elephants is not known, nor is it clear if changes in prolactin actually precede acyclicity. Understanding if acyclicity is a direct or indirect effect of hyper prolactin production will be key to the development of more targeted fertility treatments.
There is convincing evidence that prolactin in elephants is controlled through dopamine negative feedback, as in other species, based on findings that a dopamine agonist (cabergoline) decreases and an antagonist (domperidone) increases prolactin secretion (Ball and Brown 2004; J. Brown and T. Dow, unpubl). Thus, it is of interest to develop treatments that modulate prolactin secretion as a means of correcting ovarian dysfunction. Cabergoline, a dopamine agonist, is an effective treatment for hyperprolactinemia-induced infertility in women (Verhelst et al. 1999), so a clinical trial was conducted to treat hyperprolactinemic, noncycling African elephant females (1–2 mg twice weekly oral cabergoline for 4–12 months; n = 8). Cabergoline resulted in a significant and immediate reduction in prolactin during the treatment period; however, no females resumed cycling and prolactin increased to levels as high or higher after treatment withdrawal (Ball and Brown 2004; Morfeld et al. in press). Perhaps increasing the dose or extending the treatment period would be more effective, but until such trials are conducted, it is not clear if ovarian acyclicity can be corrected merely by reducing prolactin.
In addition to hypothalamic inhibition by dopamine, other factors have been identified as stimulants of prolactin synthesis or have a suppressive effect on dopaminergic tone: vasoactive intestinal polypeptide, estradiol, serotonin, oxytocin, thyrotropin releasing hormone and vasopressin (Freeman et al. 2000). With the exception of estradiol, which showed no relation to elevated prolactin (Prado-Oviedo et al. 2013), none of these potential stimulators of prolactin have been evaluated in elephants. Given the growing problem of hyperprolactinemia in the African species, these investigations appear to be warranted. Another factor that has not been examined is the role of ‘stress’ in excess prolactin secretion and ovarian inactivity, as prolactin is sometimes considered a ‘stress hormone’ (Matteri et al. 2000; La Torre and Falorni 2007). Human studies have shown that stressors such as social conflict, a new job, death of a loved one, divorce, separation from a parent during childhood, and academic pressures increase the secretion of prolactin and may predispose individuals to hyperprolactinemia and infertility (Assies et al. 1992; Sobrinho et al. 1984; Sobrinho 2003; Sonino et al. 2004). A consequence of the stress response can be reduced dopamine secretion and its tonic inhibition of prolactin, or an up regulation of prolactin stimulating factors (Calogero et al. 1998). Thus, it would be beneficial to examine how individual elephant temperaments, social relationships and life events relate to prolactin secretion and reproductive status, and if management or husbandry changes could help elephants better cope with the captive environment, similar to that described for other species (Wielebnowski 1998; Wielebnowski et al. 2002a, b; Mellen 2005; Carlstead 2009).
Whereas over two-thirds of noncycling African elephants exhibit elevated prolactin, the other third has low, baseline levels with no cyclic fluctuations. As described above, in normal cycling African elephants prolactin concentrations increase during the follicular phase and reach maximum levels immediately preceding ovulation (Bechert et al. 1999; Brown et al. 2004a). For elephants with chronically low prolactin, a trial was conducted to stimulate prolactin using domperidone (Equidone®), based on its ability to augment follicular development and improve fertility in mares (Panzani et al. 2011; Paccamonti 2012). However, although domperidone was able to increase prolactin within days of oral treatment (n = 6), continuous treatment for a 6-month period resulted in no resumption of cyclicity (J. Brown and T. Dow, unpubl), with the exception of one female that exhibited fluctuating prolactin during treatment and did start cycling. This female subsequently conceived, delivered a healthy calf and continues to cycle normally. In a follow-up trial, to mimic natural fluctuations in prolactin during the cycle, domperidone was administered in a 1 month on, 2 months off regimen for 1 year (n = 5). However, despite stimulating a cyclic pattern of prolactin, progestagen concentrations remained at baseline in all females (J. Brown and T. Dow, unpubl). Suggested next steps are to further mimic natural hormone patterns by interspersing domperidone with progestagen administration, perhaps including injections of GnRH to simulate the double LH surge; however, such drastic efforts will only be worthwhile if cyclicity continues after treatment withdrawal.
One suggested cause of reproductive problems in zoo elephants is obesity (Clubb and Mason 2002), as a high body mass index (BMI) has been correlated with acyclicity in Africans (Freeman et al. 2009). Compared to wild counterparts, captive African elephants are ~27 % heavier (Ange et al. 2001), and together these observations raise questions about whether reproductive problems may be caused in part by metabolic derangements associated with excessive body fat (Clubb et al. 2009; Mason and Veasey 2010). This is plausible given studies in horses and humans showing obesity can lead to metabolic changes that impair fertility (Vick et al. 2006; Miller et al. 2008; Jungheim and Moley 2010). For example, obese mares experience an extended interval between successive ovulations, and amenorrhea is common in obese women, not unlike the irregular cycles observed in elephants (Brown 2000). Stillbirths and dystocias also are common in obese women and horses, and are a major cause of calf mortality in elephants (Clubb et al. 2009). Such evidence suggests that elephants may be experiencing fertility problems associated with obesity, including ovarian acyclicity. To determine if obesity and related metabolic conditions exist in zoo-managed African elephants, body condition scores (BCS; 5-point scale with 1 = thinnest, 5 = heaviest) (Morfeld et al. 2014), and insulin, glucose, and leptin levels were compared between breeding-aged cycling and non-cycling elephants (N = 23 each; Morfeld 2013). Overall, 72 % had a BCS of 4 or 5, whereas none had a score of 1. The percentage of cycling females was >90 % for a BCS of 2 or 3, but only ~50 % for a BCS of 4 or 5. Perhaps more significant was the finding that leptin and insulin concentrations were higher in non-cycling as compared to cycling elephants. Using “non-cycling” as the outcome variable in regression models, and BCS, leptin, insulin, and the glucose:insulin (G:I) ratio as predictors, all but leptin were predictive of a non-cycling status, with BCS showing the strongest predictive power. Thus, these screening tools may be clinically useful for identifying at-risk elephants and developing targeted management interventions to improve body condition and insulin sensitivity with the goal of reinitiating ovarian activity. Of comparative interest, zoo Asian elephants also appear to be heavier than wild counterparts, yet ovarian acyclicity is not associated with being overweight in that species. Assessments of metabolic factors have not been conducted in that species yet, so it would be of interest to determine if they are altered in females with higher BCS as is the case for African elephants.
Finally, another factor associated with ovarian cycle problems in zoo African elephants appears to be behavioral, not physiological, with ovarian inactivity being associated with a high social dominance rank (Freeman et al. 2004, 2010). In the wild, the largest, oldest female in a herd is the matriarch and is crucial to their survival. In captivity, dominance is still important for maintaining social harmony; therefore, the energy that goes into peace keeping within a captive herd of unrelated, and sometimes incompatible females may be compromising ovarian function (Freeman et al. 2004). In mammals, reproductive inhibition can occur through the suppressive effects of primer pheromones, such as urinary chemosignals when population densities are too high, or alternatively, a dominant individual may use behavior to induce stress and shut-down reproductive mechanisms in subordinates (Wasser and Barash 1983; Creel and MacDonald 1995). While no two species use the exact same strategy, most use either behavioral or chemical suppressive mechanisms to improve their own reproductive success. Reproductive suppression is a natural strategy for many species in the wild; however, when it occurs in captivity it could be indicative of suboptimal situations (Wielebnowski 1998). Thus, research efforts are focused on determining if there are socio-management factors associated with ovarian suppression, and how it might be related to dominance status. Testosterone is known to affect aggression and dominance behaviors in many species, including bull elephants (Giammanco et al. 2005; Brown et al. 2007; Adamafio 2009), while cortisol can inhibit gonadal function through direct and indirect means (see reviews, Dobson and Smith 2000; Moberg 2000). So far, neither serum cortisol (as an index of stress) nor testosterone (as an index of dominance) have been linked to differences in social or cyclicity status (Proctor et al. 2010; Mouttham et al. 2011), so these do not appear to be driving forces in the apparent socially-mediated suppression. Presence of a bull was associated with slightly higher cyclicity rates (by 11 %) in African females; however, there were many facilities with cycling females that did not house a bull, and vice versa, so it certainly is not an absolute requirement (Dow et al. 2011a). Rather it could be due to the purposeful distribution of viable females to facilities with breeding bulls, rather than bull exposure directly. Last, a relationship between dominance and ovarian cyclicity status has not been observed in Asian elephants (e.g., Glaeser et al., 2012; J. Brown, unpubl), representing a significant species difference in how sociality or other behavioral factors affect reproductive functioning. As stated above, African elephants appear to be more sensitive to environmental and management change than Asian elephants with respect to ovarian activity. This would appear to be true for social interactions as well.
Clearly, we need to understand why so many elephant females are not cycling normally, otherwise the population collapse predicted for the U.S. will be inevitable (Faust and Marti 2011a). Prolonged acyclicity does not appear to occur in wild African elephants based on physiological studies that show females can cycle into their 50’s, although capacity declines with age (Freeman et al. 2008, 2011). It is unlikely that any one management factor is responsible, as 52 % of zoos house both cycling and non-cycling females (Freeman et al. 2009). Some elephants even alternate between cyclic and non-cyclic periods (Brown 2006). Rather, there likely are multiple etiologies, so it will be key to ascertain if problems are of physical or behavioral origin, and what is the best approach to ameliorate them (e.g., exercise programs for overweight elephants, altered social groupings, creation of multi-generational herds, increased space, etc.). Obviously, it is important to develop targeted treatments for these conditions, but given the complexity of trying to control endocrine function, it is even more important to identify underlying causes so that mitigating steps can be taken to prevent problems from occurring in the first place.
2.5 Male Physiology
Similar to females, there is a shift in the onset of sexual maturity between captive and wild bulls, especially for Asians. Successful mating has been recorded in captive males as young as 6 years of age (Keele et al. 2010; Olson 2011), whereas wild bulls generally do not breed until they are at least 25 years of age (Sukumar 2003). There also is an age difference between wild and captive bulls in the occurrence of musth—the period of heightened aggressive and sexual behavior associated with increased temporal gland secretions (TGS), urine dribbling (UD) and elevated androgen (e.g., testosterone, dihydrotestosterone, androstenedione) production (Yon et al. 2008; Ganswindt et al. 2002). In the wild, musth occurs annually in sexually mature bulls (Sukumar 2003), whereas in captivity, TGS and increased testosterone secretion have been observed in bulls as young as 7 years of age (Asian: Cooper et al 1990). Musth-like changes often are irregular in captive bulls and can occur several times a year, with or without UD, and in fact some Asians appear to be in a continual state of temporal drainage and hyper testosterone secretion (Brown et al. 2007). These patterns probably do not reflect true musth, however, which by definition refers to the competitive state in sexually active male elephants, with the presence of UD being the defining physical signal (Ganswindt et al. 2005). As with females, captive African elephant bulls appear to reach sexual maturity later and they do not exhibit musth as early as Asian bulls (Rasmussen et al. 1984; Cooper et al. 1990; Brown et al. 2007; J. Brown, unpubl). There also appears to be a species differences in elephant bulls’ responses to social factors, not unlike that observed for females. For instance, musth generally occurs in most males in multi-bull Asian groups regardless of dominance status, whereas in African groups, it is pronounced only in the dominant bull (Ganswindt et al. 2005; Brown et al. 2007; J. Brown, unpubl).
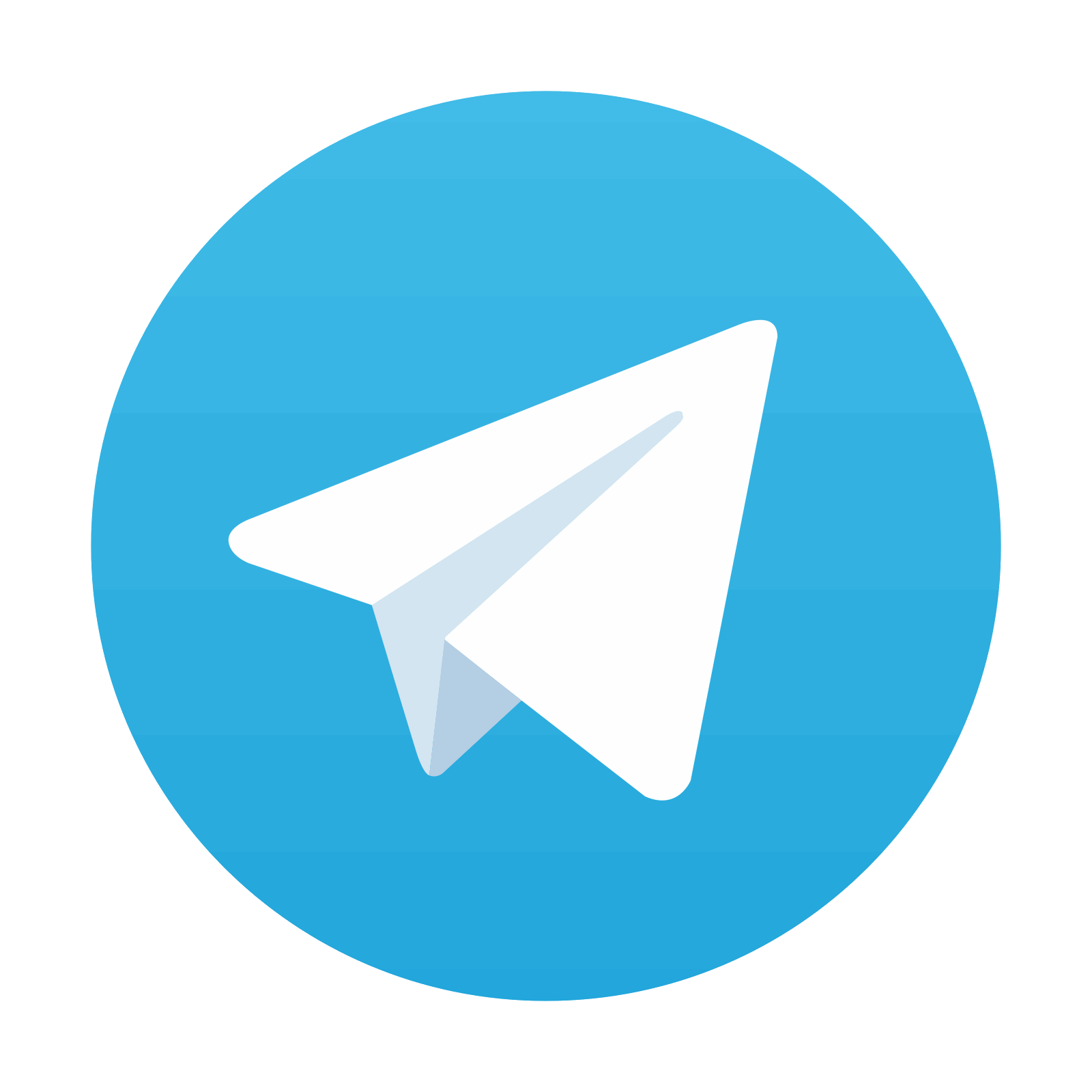
Stay updated, free articles. Join our Telegram channel

Full access? Get Clinical Tree
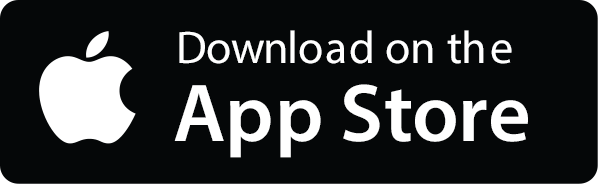
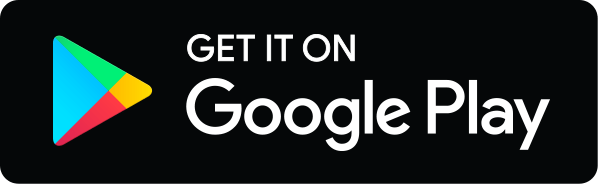