Henri G.M.J. Bertrand1 and Aurélie A. Thomas2 1 Agenda Veterinary Services, Kingston Upon Hull, East Riding of Yorkshire, UK 2 Clinical Pharmacology and Safety Sciences, AstraZeneca, Cambridge, UK With up to 100 million animals used in research annually worldwide, including 1 million animals anesthetized annually in the United Kingdom alone [1], laboratory animal medicine and anesthesia are an important area requiring veterinary involvement. With the implementation of the principles of the 3Rs – Reduction, Replacement, Refinement [2] – and increased awareness of the importance of the public’s perception of the use of animal models for research, the involvement of veterinary anesthetists in this field has been steadily increasing. There is now a general acceptance of the need to refine experiments, that is, to reduce to a minimum the pain and distress that might be experienced when animals are used in research. However, a 2009 survey indicated that less than 25% of laboratory rodents received postoperative analgesics, while less than 40% were anesthetized with agents likely to have some analgesic properties [3]. Encouraging greater use of postoperative analgesics and implementing improvements in anesthesia and intraoperative care require veterinary anesthetists to become aware of the unique challenges posed by working in a research environment. In addition to working with less familiar species, under constraints that are specific to the research project and the local legislative framework, the anesthetist may also be asked to work with agents that are not usually encountered in clinical practice (e.g., chloralose and urethane), to advise researchers on the most appropriate anesthetic and analgesic protocols in light of study goals, to accommodate animals with appropriate anesthesia lasting as short as a few seconds or as long as a few days, and to provide expertise on the ethical aspects of research projects. Although dealing with these issues may seem daunting, the involvement of veterinary anesthetists is essential to help promote improvements in anesthesia and perioperative care as well as enhancing the standards of animal welfare. Commonly used drug doses for laboratory animals are listed in Tables 54.1–54.3. Unless specified below, the reader should assume that the drugs’ pharmacokinetics in laboratory animals are broadly similar to those described in companion animals. Some particularly relevant properties of commonly used sedative, anesthetic, and analgesic agents are described in this section. Inhalants (mainly isoflurane and sevoflurane) continue to be popular in laboratory animal anesthesia for a number of reasons. Their adverse effects are well documented, they can be administered to all commonly used laboratory rodents, and one agent (isoflurane) undergoes virtually no biotransformation [10]. Consequently, isoflurane represents a good option for most commonly used research protocols when avoidance of metabolic confounding factors is required. Second, using inhalants allows rapid induction and easy adjustment of the anesthetic depth to a standard, predetermined level, therefore reducing the variation between subjects and subsequently minimizing the number of animals to be used in a particular study. Third, inhalants are commonly used for induction of small mammals in an anesthetic chamber (Fig. 54.1), a simple and easy method of anesthesia induction. Finally, recovery from isoflurane and sevoflurane anesthesia is usually rapid compared to injectable anesthetic combinations given by subcutaneous (SC) or intramuscular (IM) injection. Table 54.1 Commonly used drugs and dosages in commonly encountered small mammals. IM, intramuscular; IP, intraperitoneal; IV, intravenous; SC, subcutaneous; ??, unknown. Table 54.2 Commonly used drugs and dosages for selected primate species. IM, intramuscular; IP, intraperitoneal; IV, intravenous; SC, subcutaneous; ??, unknown. Table 54.3 Neuromuscular junction blocking agents and dosages for selected laboratory animal species. IV, intravenous; ??, unknown. Figure 54.1 Induction chamber for use with small (< 100 g) mammals. The chamber size is appropriate to the size of animal, allowing the concentration of anesthetic agent to be increased rapidly without the use of high fresh gas flows. Minimum alveolar concentration (MAC) values in smaller mammals are broadly similar to those in other species, with relatively little variation across rodent inbred strains or genotypes [11]. Although modern inhalant agents are widely considered to be both effective and non‐irritant [12,13], they vary in their pungency, and this may affect an animal’s willingness to breathe normally [14,15]. Apneic episodes can occur in rabbits with all of the commonly used agents, and this, coupled with possible catecholamine release, could increase the anesthetic risk and also introduce some intersubject variability. When inducing anesthesia in rabbits with a face mask, briefly removing the mask if an episode of apnea occurs and replacing the mask when respiration resumes will avoid the risks associated with prolonged breath holding. Some degree of aversion to volatile agents has been demonstrated in rats and mice, with isoflurane being more aversive than sevoflurane or halothane [16], and sevoflurane less aversive than isoflurane [17]. This aversion increases with repeated exposure to volatile anesthetics [18,19]. Isoflurane appears particularly irritant to guinea pigs, triggering pronounced ocular and nasal discharge. In the authors’ experience, sevoflurane appears less irritating but still causes lacrimation. Halothane is much less irritating in guinea pigs, but this needs to be balanced against the risk of other adverse effects [20,21]. The manufacture of halothane was discontinued in most Western countries such as the Unites States; however, it remains available from specialist sources for use in defined research projects. In all species, stress associated with induction of anesthesia can be minimized by use of preanesthetic medication. Inhalants anesthetic agents are fluorocarbons and act like greenhouse gases, contributing to global warming. Isoflurane has a 100‐year Global Warming Potential (GWP100) of 510, and vaporization of one 250 mL bottle of isoflurane has a similar global warming effect as 190 kg of CO2 [22]. The environmental impact of inhalants can be mitigated by using low‐flow anesthesia techniques (with a precise rodent flowmeter) and combining the use of inhalants with injectable agents for their “MAC‐sparing” effects. Ketamine remains the agent most widely used for anesthetizing laboratory rodents. It is most frequently used in combination with sedatives or sedative–analgesics, because when administered as the sole agent to rodents, it does not produce even light anesthesia. In combination with xylazine and acepromazine, ketamine produces a safe, long‐lasting (e.g., 54 ± 6 min (mean ± SEM)), and stable surgical plane of anesthesia in mice [23]. When ketamine is combined with either medetomidine or dexmedetomidine, the depth of anesthesia is less consistent than in other non‐rodent species, but this is still a useful combination in many mouse strains [23,24]. The most reliable combination for producing a surgical plane of anesthesia in rats and most other rodents is ketamine in combination with an α2‐adrenergic receptor agonist [4]. Unlike in other species, ketamine used alone in non‐human primates (NHPs) produces heavy sedation (10 mg/kg) to light surgical anesthesia (25 mg/kg) [4], with good relaxation of skeletal and laryngeal muscles. Repeated use of ketamine in NHPs, however, raises a few concerns. First, ketamine has been shown to be associated with long‐term cognitive impairment in NHPs if administered during a sensitive period for brain development (in utero or during the first week of life) [25,26]. This is a significant concern given that most NHPs are used as animal models for neuroscience studies. Repeated ketamine administrations are associated with anesthetic tolerance. Anesthetic doses administered for three consecutive days are sufficient to increase the time to recumbency by approximately 35% [27], and this is comparable with human data. Ketamine has also been reported to cause local myotoxic effects in New World primates [28], such that other injectable agents such as alfaxalone may be preferred for IM injection in these species. Alternatives to ketamine use in primates (using α2‐adrenergic receptor agonists, benzodiazepines, and/or opioids) have been described [29–31]. Although the magnitude of sedation may be unsuitable for surgical procedures, a combination of fentanyl–midazolam–medetomidine (10 μg/kg, 0.5 mg/kg, and 20 μg/kg, respectively) administered IM provides reliable sedation and can be easily reversed with atipamezole–naloxone (0.2 mg/kg and 10 μg/kg, respectively), providing rapid and good quality recovery [31]. It is of interest that NHPs are notably resistant to sedation with benzodiazepines. Doses as high as 3 mg/kg, administered orally, merely produce light sedation and anxiolysis, without inducing recumbency [30]. Most injectable anesthetic agents have similar properties in small rodents and companion animals and, therefore, are not discussed in depth here. One noteworthy difference relating to the use of injectable anesthetics in small rodents is the difficulty of obtaining intravenous access. This results in anesthetic combinations being administered as a single injection by the intraperitoneal (IP), SC, or IM route, rather than intravenously, to effect. Although this is a simple and rapid means of producing anesthesia, it has inevitable consequences in relation to the safety of certain anesthetic agents, especially those with a narrow safety margin. Since there is considerable variation between different strains of rodents in their response to injectable anesthetic agents, anesthetic combinations that have a broad safety margin or are wholly or partially reversible are preferred. If a neuroleptanalgesic combination is used (typically, fentanyl combined with either medetomidine/dexmedetomidine or acepromazine), fentanyl can be partially antagonized with small doses of buprenorphine, butorphanol, or even nalbuphine instead of complete reversal with naloxone [32]. By doing so, the respiratory depressant effects and some of the sedative effects induced by the potent μ‐opioid receptor agonist are reversed, but some analgesia is preserved for the immediate recovery period. Note that the formulation Hypnorm® (fentanyl–fluanisone) is not readily commercially available anymore. As in other species, the potent, short‐acting opioids (e.g., phenylpiperidines including alfentanil, sufentanil, and remifentanil) are effective agents for multimodal anesthesia in laboratory animals and can significantly reduce required inhalant anesthetic concentrations. However, their respiratory depressant effects may necessitate respiratory support (i.e., intubation and ventilation), which can be more challenging in rodents. When suitable apparatus and expertise are available, their use combined with medetomidine can constitute a refinement to more classic injectable protocols in rats [33]. Alfaxalone, a neurosteroid, has attracted attention in laboratory animal anesthesia. Laboratory mice and rats administered alfaxalone alone IP or SC never reached a surgical plane of anesthesia and the addition of opioids and/or α2‐adrenergic receptor agonists is necessary to facilitate invasive procedures. It is important to note that doses and effects of alfaxalone vary greatly between sex and rodent strain and pilot studies are highly recommended to refine the anesthesia protocol before initiating the experiment [5,34–36]. In NHPs, similar effects of a single dose of alfaxalone have been observed, and co‐administration with other anesthetics is necessary to induce deep sedation or general anesthesia [9,37,38]. Alfaxalone does not produce muscle damage, as does ketamine, and this can be very beneficial in small primates [39]. Some injectable anesthetic agents remain in research settings due to scientific reasons or ease of use but are no longer used in veterinary clinical practice. Urethane is an ethyl carbamate producing long‐lasting and stable (6–10 h) surgical anesthesia with few adverse cardiovascular or respiratory effects [40,41]. Urethane is still used for non‐recovery procedures involving recording of certain central nervous system (CNS) responses and for neuropharmacology studies. Spinal reflexes are depressed, but it has virtually no depressant action on the brain itself (EEG comparable to sleep patterns), and most autonomic reflexes are well preserved [42]. It is a water‐soluble compound metabolized by the liver into ethanol and carbamic acid before renal excretion. Despite initial studies suggesting that urethane mainly acts on GABAA receptors, it is now established that only 23% of urethane’s action is GABAA mediated. Urethane interacts at a multitude of receptors including the α1‐glycine receptor (agonist, 33%), the NMDA and AMPA receptors (antagonist, 10% and 18%, respectively), and possibly acetylcholine receptors, but with only weak effects at each target [43]. When administered IP, it has profound endocrine and metabolic effects, producing peritonitis, necrosis of the abdominal contents, and massive leakage of plasma into the peritoneal cavity [41], so must only be used for non‐recovery procedures. Urethane is a carcinogen and protective equipment should be worn when it is handled [44]. α‐Chloralose is an anesthetic that has numerous dose‐dependent CNS effects, both excitatory and inhibitory. It provides long‐lasting light anesthesia (8–10 h), with minimal cardiovascular side effects. However, induction and recovery are very prolonged and accompanied by excitement, so the agent is usually restricted to terminal procedures after induction of anesthesia with another agent (typically an inhalant). A further disadvantage of chloralose is that anesthetic depth is not usually sufficient for surgical manipulations to be performed, and it has poor analgesic properties. Chloral hydrate is used occasionally in rodent anesthesia for neuropharmacology studies. Surgical depth of anesthesia can be achieved [40], but in some strains of rats, chloral hydrate causes postanesthetic ileus that can be fatal [45]. Using a dilute solution of chloral hydrate may help reduce the incidence of ileus. Tribromoethanol is used primarily in mice although its popularity has declined markedly because of undesirable side effects. If incorrectly prepared or stored, administration is associated with high mortality. This can also occur when freshly prepared solutions are used [46]. The incidence of adverse effects varies greatly in different research facilities, perhaps due to the agent itself not being supplied as a commercial pharmaceutical product. For this reason, decisions as to whether to use the agent should be made on a case‐by‐case basis. When used successfully, tribromoethanol produces 15–20 min of surgical anesthesia, with reasonably rapid recovery. Despite growing emphasis on animal welfare and refinement of animal models, less than 25% of laboratory rodents undergoing surgical procedures are given analgesic drugs (35% of those that are, receive buprenorphine [3]). This might be partially explained by a number of largely unfounded concerns related to potential interactions of opioids and other analgesics and specific types of research projects. For example, despite a lack of convincing clinical data in humans, opioids are commonly associated with immunodepression in critical care patients. This has led to concerns that use of these agents in a research setting could cause immunomodulation, and this could introduce confounding factors in some research models. In laboratory animals, this phenomenon is subject to major interstrain variability [47], and immunodepression is only evident when animals receive doses that are significantly greater than those used for postoperative pain relief (e.g., 300 mg/kg morphine) for prolonged periods of time [48–50]. Opioids have also been shown to influence tumor growth and metastasis, and this has been considered a contraindication for their use when implanting or transplanting tumors for cancer research studies. Published data are conflicting in this area, suggesting that morphine can either promote or slow down tumor growth and metastatic spread. The mechanisms involved remain poorly understood and, in addition to their potential immunomodulating effects, opioids may impact tumor cell aggressiveness and angiogenesis [51]. In each of these examples, a proper appraisal of the available literature in consultation with the researchers involved should lead to an evidence‐based decision as to whether to use opioids or whether an alternative analgesic strategy should be adopted. When making these decisions, it is also important to include a consideration of the various effects of unalleviated pain. In the authors’ experience, there are almost no circumstances in which an analgesic regimen of some type cannot be implemented and untreated pain can be a greater confounder of scientific results [52,53]. Among the opioids, buprenorphine remains the analgesic agent most frequently administered to laboratory animals, both to rodents [3] and to larger species [54]. Buprenorphine is 35 times more potent than morphine when administered IM in rats [55] and has a long duration of action (3–5 h in mice, 6–8 h in rats). Its poor oral bioavailability (5–10%), coupled with a significant hepatic first‐pass metabolism, renders oral administration of buprenorphine of limited value in rodents as its duration of action decreases to 1–2 h (0.5 mg/kg, rats) [56], although this may still provide sufficient analgesia following some surgical procedures [57]. Although use of buprenorphine in palatable oral formulations remains popular, we recommend it is only given by this route when effective methods of pain assessment are being used. Buprenorphine’s ceiling effect has been well‐described in the literature and occurs only when doses exceeding about 3 mg/kg are administered in rats [58]; therefore, it is of little concern when using this agent for postoperative pain relief. Clinically relevant adverse effects of opioids are similar to those in other animal species. Interestingly, studies in rats showed that even at large IV doses, buprenorphine failed to produce clinically significant respiratory depression [59,60]. An unusual effect of opioids in rodents is the production of pica behavior. This is thought to be analogous to vomiting in other species and has been reported following use of buprenorphine in rats [61]. The incidence of pica appears to be low, but, if noted, analgesia should be provided using a non‐opioid analgesic. Other opioids can be used in laboratory animals. Methadone, for instance, has proven antinociceptive properties in mice and rats [62] and was shown to attenuate signs of neuropathic pain for 2 h in mice (3 mg/kg SC) [63]. This effect may be due to μ‐opioid receptor agonist actions on peripheral opioid receptors [62], in combination with antagonism of NMDA receptors. Although species‐specific studies documenting the efficacy of this agent in alleviating postsurgical pain are lacking, methadone has been used by the authors in NHPs (0.3–0.5 mg/kg IM) when the surgical procedure renders the animal at risk of experiencing chronic or neuropathic pain. Compared to other full μ‐opioid receptor agonists, methadone seems to produce less sedation and inappetence. Managing pain in laboratory rodents can be difficult because of the large numbers of animals that may need to be treated (e.g., 20–30 animals all undergoing surgery in one day). In addition, the relatively short action of some analgesics requires repeated handling and injections, which in itself can be stressful. Possible approaches to these problems are the use of slow‐release preparations and transdermal delivery (e.g., cutaneous patch) technology. Fentanyl patches or transdermal solutions can be used in larger laboratory animals, with the same concerns and indications for use as in other species. It is important to securely attach the patch to the animal to reduce the risk of ingestion. Ingestion of a patch can be fatal since fentanyl is readily absorbed by the oropharyngeal mucosa [64]
54
Comparative Anesthesia and Analgesia – Laboratory Animals
Introduction
Anesthetics and analgesics
Inhalant anesthetics
Mice
Rats
Guinea pigs
Rabbits
Comments
References
Atropine
0.04 mg/kg SC
0.05 mg/kg IP, SC
0.05 mg/kg IM, IP
50–250 μg/kg IM, SC
[4]
Glycopyrrolate
??
0.5 mg/kg IM
??
0.1 mg/kg IM, IV
[4]
Acepromazine
2–5 mg/kg IP, SC
2.5 mg/kg IM, IP
0.5–1 mg/kg IM
1 mg/kg IM
[4]
Xylazine
5–10 mg/kg IP
1–5 mg/kg IP
5 mg/kg IP
2–5 mg/kg IM, SC
[4]
Medetomidine
30–100 μg/kg SC
30–100 μg/kg SC
0.5 mg/kg IM, IP
0.1–0.5 mg/kg IM, SC
[4]
Dexmedetomidine
15–50 μg/kg SC
15–50 μg/kg SC
0.25 mg/kg IM, IP
50–250 μg/kg, IM, SC
[4]
Diazepam
5 mg/kg IP
2.5–5 mg/kg IP
2.5 mg/kg IP, IM
1–2 mg/kg IM
[4]
Midazolam
5 mg/kg IP
5 mg/kg IP
5 mg/kg IP
0.5–2 mg/kg IV, IM, IP
[4]
Alfaxalone
80 mg/kg IP
2–5 mg/kg IV,
20 mg/kg IP
40 mg/kg IM, IP
9–12 mg/kg IM
[4–6]
Ketamine
100–200 mg/kg IM
50–100 mg/kg IM, IP
40–100 mg/kg IM, IP
25–50 mg/kg IM
[4]
Ketamine plus
acepromazine
100 mg/kg,
5 mg/kg IP
75 mg/kg,
2.5 mg/kg IP
100 mg/kg,
5 mg/kg IP
50 mg/kg,
1 mg/kg IM
Light anesthesia
[4]
Ketamine plus
dexmedetomidine
100 mg/kg,
0.25 mg/kg IP
60–75 mg/kg,
0.125–0.25 mg/kg IP
40 mg/kg,
0.25 mg/kg IP
15 mg/kg,
0.125 mg/kg SC
Surgical plane of anesthesia, but more variable effects in mice
[4]
Medetomidine plus
midazolam plus
fentanyl
0.5 mg/kg,
5 mg/kg,
50 μg/kg SC
0.15 mg/kg,
2 mg/kg,
5 μg/kg SC
0.2 mg/kg,
1 mg/kg,
25 μg/kg SC
0.2 mg/kg,
1 mg/kg,
20 μg/kg IM
[4]
Alfaxalone plus
dexmedetomidine
30 mg/kg,
0.3 mg/kg IP
30 mg/kg,
0.05 mg/kg IP
??
6 mg/kg,
0.2 mg/kg IM
[4,7]
Urethane
??
1000 mg/kg IP
1500 mg/kg IV, IP
1000–2000 mg/kg IV
Non‐recovery procedures only
[4]
Macaque spp
Marmosets
Comments
References
Atropine
0.04 mg/kg SC
0.05 mg/kg IP, SC
[4]
Glycopyrrolate
??
0.5 mg/kg IM
[4]
Diazepam
5 mg/kg IP
2.5–5 mg/kg IP
[4]
Xylazine
5–10 mg/kg IP
1–5 mg/kg IP
[4]
Medetomidine
30–100 μg/kg SC
30–100 μg/kg SC
[4]
Dexmedetomidine
15–50 μg/kg SC
15–50 μg/kg SC
[4]
Ketamine
5–25 mg/kg IM
15–50 mg/kg IM
Immobilization
[4,8]
Ketamine plus
dexmedetomidine
5–10 mg/kg,
0.01–0.03 mg/kg IM
5 mg/kg,
0.05 mg/kg IM
Light to moderate anesthesia
[4]
Ketamine plus
xylazine
10 mg/kg,
0.15–0.5 mg/kg IM
10 mg/kg,
0.15–0.5 mg/kg IM
Light to moderate anesthesia
[4]
Alfaxalone
1–3 mg/kg IV (after initial ketamine sedation)
10 mg/kg IM, 2–5 mg/kg IV
Immobilization (IM), light–medium anesthesia
[4]
Medetomidine plus
midazolam plus
alfaxalone
0.02 mg/kg,
0.3 mg/kg,
2 mg/kg SC
??
[9]
Medetomidine plus
midazolam plus
fentanyl
0.02 mg/kg,
0.5 mg/kg,
10 μg/kg SC
??
[4]
Mice
Rats
Guinea pigs
Rabbits
Non‐human primates
References
Alcuronium
??
??
??
0.1–0.2 mg/kg IV
??
[4]
Atracurium
??
??
??
??
0.3–0.6 mg/kg IV
[4]
Pancuronium
??
2 mg/kg IV
0.06 mg/kg IV
0.1 mg/kg, IV
0.08–0.1 mg/kg IV
[4]
Vecuronium
??
1 mg/kg IV
??
??
0.04–0.06 mg/kg IV
[4]
Injectable anesthetics
Opioids
Stay updated, free articles. Join our Telegram channel

Full access? Get Clinical Tree
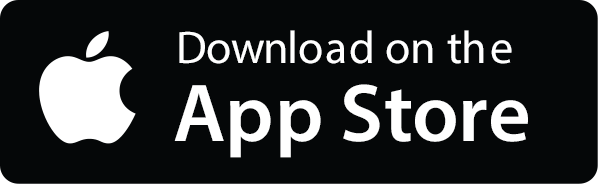
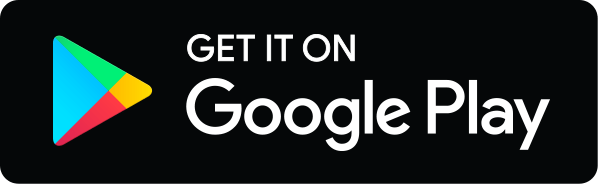