7 Michel R. Popoff Clostridium botulinum is defined as a Clostridium sp. that produces one or several neurotoxins inducing flaccid paralysis. These are termed botulinum neurotoxins (BoNTs) and are responsible for botulism, a rare but often severe disease with a high lethality rate. In contrast, Clostridium tetani synthesizes a related neurotoxin called tetanus toxin (TeNT), which causes a dramatic spastic paralysis. Both BoNTs and TeNT exert neurotoxicity by blocking the release of neurotransmitters. While BoNTs inhibit the evoked release of acetylcholine at the neuromuscular junctions causing the aforementioned flaccid paralysis, TeNT impairs neuroexocytosis at central inhibitory interneurons, resulting in prevention of the inhibitory pathways and, thus, inducing the noted spastic paralysis. BoNTs and TeNT are the most potent toxic compounds and are responsible for severe, often-fatal paralysis in humans and domestic animals. Clostridia capable of producing BoNTs display heterogeneous bacteriological characters and are divided into several species and groups. The taxonomic position of the C. botulinum species was originally based on only one phenotype; the BoNT non-toxic variant strains, genetically related to C. botulinum, were assigned to different species, such as Clostridium sporogenes and Clostridium subterminale. It soon transpired that BoNTs are, in fact, seven different protein neurotoxins that are immunologically distinct and have been designated by the letters A to G. A novel type, BoNT/H, which seems to be a hybrid between BoNT/F and BoNT/A, has been reported but awaits further characterization. More recently, sequence analysis has permitted scientists to distinguish subtypes within the BoNT types (see below and Table 7.1). However, all of them cause the typical flaccid paralysis of botulism in experimental animals, similar to that observed in humans and domestic and wild animals suffering from botulism. Physiological differences among C. botulinum strains have been identified, but the production of the different BoNT types does not necessarily correlate with C. botulinum strain phenotypes. The species has been divided into six physiological groups (Table 7.1): Table 7.1 Groups and main properties of botulinum neurotoxin producing Clostridia, botulinum neurotoxin types and subtypes according to (Peck 2006, Peck 2009, Peck et al. 2011, Mazuet et al. 2012, Raphael et al. 2012, Hill and Smith 2013, Diao et al. 2014, Raphael et al. 2014, Weedmark et al. 2014, Kull et al. 2015, Mazuet et al. 2015) aThermoresistance of spores in phosphate buffer at pH7 according to (Peck et al. 2011). bThermoresistance of spores in liquid medium at pH 7 according to (Diao et al. 2014). ND: no data available. Group IV, which also includes non-toxic strains previously identified as C. subterminale and C. hastiforme, is metabolically distinct from the other groups and has been assigned to a different species called C. argentinense. Genetic analysis using various techniques (23s and 16S rRNA gene sequencing, amplified fragment length polymorphism (AFLP), pulse-field gel electrophoresis (PFGE), multilocus sequence typing (MLST)) supports the view that the different groups of botulinum neurotoxin-producing clostridia correspond to distinct species. Phenotypic properties, DNA/DNA homology, and 16S rRNA analysis of all strains in each group, regardless of toxin type, are closely related but not strictly identical. Atypical toxigenic C. butyricum and C. baratii strains are phenotypically and genetically related to the type strains of these species and not to the other BoNT-producing clostridia. The genetic diversity of the botulinum-neurotoxin-producing clostridia versus non-neurotoxigenic clostridia is well illustrated by the analysis of 16S rRNA gene sequences. The six groups of botulinum-neurotoxin-producing clostridia are located on distinct phylogenetic branches as individual Clostridium spp. Whole genome sequences of representative Clostridium botulinum strains are available and confirm the distribution of botulinum-neurotoxin-producing clostridia in various genetic groups. An overall comparison between complete C. botulinum genomes revealed strong similarity between genomes from group I strains, and their great distance from group II strains. Most proteins (81–86%) of group I strains share a protein identity of over 90%, but only 2–3% of proteins from group II genomes have orthologs encoded by group I genomes with such a high protein identity. However, several lineages can be differentiated among group I strains which likely represent strain adaptation to environmental or clinical niches. Indeed, nordic C. botulinum type B strains from group I belong to two homogeneous clusters, which differ by their resistance to toxic compounds such as cadmium and arsenic. Since a 100-fold higher concentration of arsenic occurs in certain areas of Finland compared to other European sites, the acquisition of arsenic resistance possibly represents an adaptive response to growing in specific environments. Moreover, whole-genome phylogenic single-nucleotide-polymorphism (SNP) analysis revealed five distinct lineages among C. botulinum strains of group I according to type/subtype and/or botulinum locus organization with ha or orfX operon (see below). Although monitoring with a DNA microarray shows that group II C. botulinum strains are genetically closely related, two subsets can be individualized, one encompassing type B and F strains and a second containing the type E strains. The strains of the two subsets share common physiological properties such as minimal growth temperature and inhibitory NaCl concentration, but differ by their profile of carbohydrate metabolism. The strains of this group are characterized by proteolytic and lipase activity and by no or weak acidification of carbohydrates (Table 7.1). Glucose is acidified weakly. The cell wall contains glucose as sugar. The optimal temperature for growth is 37 °C. The strains of group I usually do not grow and do not form toxins at 10 °C or below. However, a large inoculum and long incubation period might result in sufficient growth and toxin formation at low temperatures such as 12 °C within 3–4 weeks. Growth of group I strains is inhibited by acid pH (< 4.6) and NaCl concentrations ≱ 10%. The thermoresistance of the spores is usually high (above 120 °C), but varies according to strain and the heating of the buffer and recovery culture medium. Botulism was a major problem in the early days of the canning industry and, since its inception, the thermotolerance of C. botulinum strains has been extensively investigated in order to recommend safety rules for heat treatment. The most heat-resistant strains require heating at 121 °C for 0.21 min to reduce the number of viable spores by a factor of 1012 (12-D or D value). Therefore, for commercial and low-acid canned foods, heating at 121 °C for 3 min has been recommended. Group II strains acidify various carbohydrate substrates (amygdalin, dextrin, fructose, galactose, glucose, glycogen, maltose, ribose, sorbitol, sucrose, and trehalose but not lactose, mannitol, melibiose, or salicin). They mainly produce butyric and acetic acids from trypticase–yeast extract–glucose broth. They hydrolyze gelatin, but are non-proteolytic. The strains within this group contain glucose and galactose as cell-wall sugars. They have a lower optimal temperature of growth (around 25–30 °C) and can grow and produce toxins at very low temperatures (Table 7.1). Growth and toxin production have been reported to occur at temperatures as low as 3.0–3.3 °C in 5–7 weeks. However, most of the strains grow poorly below 5 °C. Spores are only moderately resistant to heat and do not survive 10 min at 90 °C. The highest temperature resistance of these spores in a phosphate buffer was reported to be 82.2 °C. Spores of group II strains are lysozyme-dependent for their germination. Lysozyme diffuses in the spore coat and induces peptidoglycan hydrolysis in the cortex, permitting the first step of germination. Lysozyme also increases spore recovery after heat treatment and thus increases heat resistance. In the presence of lysozyme, the heat resistance time at 82.2 °C is increased by a hundred-fold, from 2.31 min to 231 min. BoNT produced by group II strains, mainly type E, is not fully activated by endogenous protease. Trypsinization enhances the toxicity of these cultures. In addition, strains from group II are more sensitive than proteolytic C. botulinum strains to NaCl (inhibitory concentration 5% and above) and to low pH, with no growth below pH 5. The organisms within this group are either non-proteolytic or only very slightly so. They ferment glucose, glycerol, inositol, ribose, and xylose. Their cell wall does not generally contain sugar but may contain traces of glucose. The main end products of metabolism are acetate and butyrate. They grow at higher temperatures than the other C. botulinum strains, with the optimal temperature for growth being 37–40 °C. Most strains within this group grow well at 45 °C, with the minimal growth temperature being approximately 15 °C. This group is heterogeneous according to some biochemical properties (the fermentation of several sugars and production of indole and hydrogen sulfide). Division into four subgroups has been proposed. The strains of this species are proteolytic, hydrolyze gelatin, and do not ferment any of the usual carbohydrates. Unlike strains within the other C. botulinum groups, C. argentinense strains do not produce a lipase. They do, however, produce acetate, butyrate, iso-butyrate, iso-valerate, and phenylacetate as end products of metabolism. The optimal temperature of growth is 30–37 °C. The spores exhibit a similar heat resistance to group III strains. Phenotypic differences have been observed between toxigenic and non-toxigenic C. argentinense strains by using cellular fatty analysis and multilocus enzyme electrophoresis. C. argentinense strains are phenotypically and genetically closely related to C. subterminale strains that are non-neurotoxigenic. Toxigenic C. butyricum strains are phenotypically and genetically related to the typical strains of the C. butyricum species. They are non-proteolytic nor do they hydrolyze gelatin, but they strongly acidify various carbohydrates including glucose, cellobiose, fructose, galactose, glycogen, lactose, maltose, mannose, melibiose, raffinose, ribose, salicin, starch, sucrose, trehalose, xylose, and pectin. They do not produce lipase or lecithinase. They are mesophilic bacteria with an optimal growth temperature of 30–37 °C. The lowest pH for growth and toxin synthesis was found to be 4.8. Spores of neurotoxigenic C. butyricum are less resistant to heat (D100 °C < 0.1 min) than non-toxigenic strains (D100 °C = 4.7 min) . C. butyricum strains producing type E neurotoxin have been isolated from cases of infant botulism and from young people in Italy, as well as from cases associated with the consumption of fermented soybean in China and in India and Japan. Based on the toxin gene sequence, toxigenic C. butyricum strains from Italy and China are divided into two distinct subtypes, termed E4 and E5, respectively, indicating independent evolution of bont/E after transfer into C. butyricum. Toxigenic and non-toxigenic C. baratii strains display similar morphological and biochemical characteristics. C. baratii is phenotypically closely related to C. perfringens. Both species are non-motile and appear as thick, straight rods (0.5–1.9 x 1.6–15 μm). Strains sporulate poorly in an anaerobic culture medium. They produce lecithinase but not lipase. C. baratii is readily differentiated from C. perfringens by the inability of C. baratii to hydrolyze gelatin. The optimal growth temperature is around 30–45 °C, with a minimum at around 10–15 °C. C. baratii acidifies culture medium containing various carbohydrates. Neurotoxigenic C. baratii strains are genetically related to their non-toxigenic counterparts. Botulinum neurotoxin type F gene sequences from C. baratii form a cluster distinct from that containing strains of C. botulinum type F. Neurotoxigenic C. baratii type F is involved in a limited number of human cases, mainly due to intestinal colonization of infants or adults. C. botulinum is widespread in soils as well as in lake and sea sediments in most parts of the world. However, the different toxinotypes of C. botulinum are not equally distributed, some of them being restricted to particular ecological areas. The factors responsible for the geographical distribution of the different toxinotypes are poorly understood. In general, toxinotypes A, B, E, F, and G seem to have their principal habitat in soil, and sea- and fresh-water sediments. Toxinotypes A and B occur more frequently in soil, and the regional distribution of these two toxinotypes is different. Toxinotype E is more predominant in sea or lake sediments and fish than in soil. Toxinotypes C and D appear to be obligate parasites of birds and of other animals. Cadavers of animals or birds dead from botulism, or healthy carriers are the main sources of these organisms. They are seldom encountered in soil samples, except in the areas where the incidence of animal botulism is high. C. botulinum is not usually found in the digestive tract of healthy humans, but it can be found in that of animals, particularly C. botulinum C and D in regions where botulism is frequent. Numerous investigations have surveyed the prevalence of C. botulinum, particularly in the USA. Types A and B were generally found in neutral to alkaline soil samples poor in organic matter, and much more rarely in aquatic sediment. C. botulinum type A is predominant in the western part of the United States (west of the Missouri and Mississippi rivers), in soil that is neutral to alkaline (average pH 7.5) with a lower than average organic content. In contrast, type B prevails largely in the eastern part of the USA. This toxinotype was recovered in slightly more acidic soil samples (average pH 6.25) with a higher level of organic matter and mainly from cultivated soils (pastures and fields). Other investigations reported the prevalence of type B in cultivated soil samples, but noted that this type is rare in soils contaminated with manure or animal feces. Fertilization was not considered a significant factor responsible for the frequent presence of C. botulinum type B in cultivated soils. The incidence of C. botulinum A and B is very low in aquatic (sea- or fresh-water) sediments and soil samples in the northern part of North America (Alaska, Canada). Fewer investigations have been performed in Central and South America. Surveys of soil samples from Argentina, Brazil, and Paraguay demonstrated the presence of C. botulinum A and B, with a greater prevalence of type A. C. botulinum type B is the most common type from soil and sediment samples in central and southern Europe (Great Britain, Ireland, Netherlands, France, Switzerland, and Italy), and from soil samples in Denmark. In Great Britain and Ireland, C. botulinum is much more frequent in lake and loch sediments than in soil samples, and type B is predominant. C. botulinum A and B occur broadly in Asia, including the countries of the former USSR, China, and Taiwan. Contamination levels and type detection vary from region to region and depend upon factors including temperature, moisture, organic substance content, and other unidentified factors. Soil from southern regions with warm climates or subtropical and tropical areas showed the highest incidence of C. botulinum A and B. Highly populated regions are much more contaminated than desert areas. Distribution of types A and B varies locally and does not correspond to large geographical areas, as in North America. There are few reports on the incidence of C. botulinum in Africa. In Kenya, soil samples seemed to be heavily contaminated, with a predominance of type A. In contrast, the contamination in South Africa was low, with C. botulinum B identified in three soil samples out of 102. In Australia and New Zealand, C. botulinum A and B have been found, but their presence in the environment is low, consistent with a low incidence of human botulism in these areas. The distribution of type E is more regional than other types. Type E is mainly found in northern areas of the Northern Hemisphere. This includes the northern part of North America (Alaska, Canada, northern United States), northern Europe (Ireland, Greenland, Denmark, Norway, Sweden, and the coast of the Baltic Sea), and North Asia (northern part of the countries of the former USSR, the Caspian Sea, and northern Japanese islands, including Hokkaido and the northern part of Honshu). The ability of C. botulinum E to grow at a very low temperature is reflected in its prevalence in areas with cold temperatures. Its frequency decreases considerably in southern areas with warm weather. This type is mainly found in the aquatic environment (sediment and soil from the shores of lakes, seas, and rivers). Other factors such as organic matter content, salinity, and other unidentified factors influence the distribution of type E, which varies greatly from one region to another. Thus, this organism is commonly found in the Great Lakes in the northern United States, but more frequently in Lake Michigan than in any of the other lakes, and in Lake Michigan it was found more frequently in Green Bay than elsewhere. Type E is also very common in the Pacific Northwest of North America. Sediment samples of Lake Washington near Seattle contained 18 to 25 C. botulinum E per g. These particular areas seem to represent the principal habitat of C. botulinum E, where it can grow and multiply. A particularly high prevalence of C. botulinum E occurs in the Baltic Sea. This bacterium is most frequent and spore counting more abundant in sea- than in fresh-water sediment samples. Low oxygen content, low salinity, the presence of biomass, and depth seem to be more important factors than temperature controlling the propagation of type E in the aquatic environment. Contamination of raw fish in Finland ranges from 10 to 40% depending on the fish species. Type E has also been detected in fish roe (4–14% of the samples), vacuum-packed (5%) and air-packed (3%) fishery products, and vacuum-packed hot-smoked whitefish (10%). Investigations on Finnish trout farms revealed C. botulinum type E in farm sediment samples (68%), fish intestinal contents (15%), and fish skin samples (5%). A recent investigation in northern France showed a prevalence of C. botulinum of 16.5% from 175 sea fish samples, but the predominant toxinotype was type B (72%) followed by types A (24%) and E (4%). Only one sediment sample in 25 contained C. botulinum E. C. botulinum types C and D are widespread throughout the world. C. botulinum C is mainly found in mud and sediments of marshes, ponds, and seashore where botulism in waterfowl is endemic. Outbreaks of botulism in birds and the presence of C. botulinum C in their environment have been reported in the United States, Great Britain, Denmark, Netherlands, France, and Japan. Intestinal contents and cadavers of susceptible birds seem to be the principal habitat of C. botulinum C. This type has also been detected in soil from warm areas such as in Indonesia, Bangladesh, and Thailand. C. botulinum D is more frequently associated with botulism in animals (ruminants, horses, and others). Carcasses of these animals and also from small animals (rodents and others) constitute the most common source of this organism. C. botulinum D can also be identified in soil samples where animal botulism is common (south and central Africa, Australia, America, and Europe). Types F and G are much less frequently encountered than other types. Since the first identification of C. botulinum type F from a homemade liver paste responsible for a human botulism outbreak on the Danish island Langeland, this type has been demonstrated in marine sediment of the west coast of the United States, and in marine and fresh-water sediments in Brazil, Venezuela, and Indonesia. C. botulinum G was first isolated from a soil sample in Argentina, and was identified from necropsy specimens in cases of unexplained death in adults and infants, and from five soil samples out of 41 in close association with cultivated land in Switzerland. C. butyricum is a widespread bacterium in the environment, including soil, fresh-water and marine sediments, cheese, the rumen of healthy calves, animal and human feces, and more rarely in clinical specimens (blood, urine, respiratory tract, pleural cavity, abdomen, wounds, and abscesses). C. butyricum has been involved in cases of necrotizing enterocolitis in newborns. Artificial feeding and intestinal stasis support abundant proliferation of C. butyricum in the intestinal content. Bacterial overgrowth is accompanied by large-scale production of hydrogen and organic acids (mainly butyric acid) which have been recognized as the main virulence factors of C. butyricum in neonatal necrotizing enterocolitis. The first two neurotoxigenic C. butyricum strains were isolated from infant botulism in 1985–1986 in Rome (Italy). Extensive studies have been carried out in the vicinity of Rome, and no strains producing BoNT/E were isolated; only C. botulinum A and B strains were found in 9.6% of 52 soil samples. Ten years later, two additional cases of toxico-infection with neurotoxigenic C. butyricum strains were described in young people in Italy. These isolates were genotypically and phenotypically identical to the former Italian strains. In 1994, several cases of food-borne botulism were reported in China. The implicated food consisted of salted and fermented paste made of soybeans and wax gourds. C. butyricum type E was isolated from soil samples around the patients’ houses. The bont/E gene sequences from 11 Chinese C. butyricum strains were identical and differ from those of C. botulinum E (96.9% identity at the amino acid level) and the Italian C. butyricum strain BL6340 (95% identity). PFGE, Southern blot hybridization, and randomly amplified polymorphic DNA permitted distinction of three clones among the neurotoxigenic C. butyricum strains: two corresponding to the Chinese isolates, one of which was responsible for the food-borne botulism outbreak, and one to the Italian strains. This indicates that the neurotoxigenic C. butyricum strains are clonally distributed across vast areas. In contrast, C. botulinum type E strains from fish and fish products showed a wide biodiversity (62 different subtypes among 92 isolates). C. baratii is isolated from soil, sediments, normal human and rat feces, and occasionally from war wounds, peritoneal fluid, and infection of the eyes, ear, and prostate. The source of the toxigenic C. baratii producing a BoNT/F responsible for one case of infant botulism and cases of adult botulism in the United States, Spain, and France has not been elucidated. BoNTs are produced by neurotoxigenic strains of clostridia, together with several associated non-toxic proteins (ANTPs). BoNTs and ANTPs associate to form large complexes of various sizes, also known as progenitor toxins. ANTPs encompass a non-toxic, non-hemagglutinin component (NTNH) and several hemagglutinin components (HAs) or OrfX proteins. The genes encoding the neurotoxins and ANTPs, which associate with BoNT to form the botulinum complexes, have been cloned and sequenced in representative clostridial strains of each BoNT type. The BoNT and ANTP genes are clustered in close vicinity and constitute the botulinum locus. They are organized into two operons. The operon localized in the 3′ part of the botulinum locus contains bont, which is immediately preceded by ntnh, which encodes the NTNH component. The ntnh–bont operon is conserved in all C. botulinum strains. In C. botulinum types E and F and certain C. botulinum A strains, this operon contains an additional gene called p47, encoding a 47 kDa protein. The second operon consists of the hemagglutinin (HA) genes or OrfX genes and is localized upstream of the ntnh–bont operon. The ha or orfX operon is transcribed in the opposite orientation to that of the ntnh–bont operon and shows more strain variation than the ntnh–bont operon, which is highly conserved in all C. botulinum strains. The ha operon consists of three genes (ha70, ha17, and ha33) in C. botulinum B, C, D, and some A strains. The ha genes of C. botulinum G only comprise ha17 and ha70. The ha genes are missing in the non-hemagglutinating toxinotypes A1, A2, A3, A4, E, and F and an orfX operon (orfX1, orfX2, orfX3) instead of an ha operon lies upstream of the ntnh–bont operon. It is noteworthy that a bont gene can be inserted into an HA or OrfX locus. However, bont/A1 is the only gene that has been found in either of the two types of botulinum locus. A gene (bontR, previously called orf21 or orf22), encoding an alternative sigma factor involved in the regulation of botulinum locus gene expression, is present in different positions in C. botulinum strains except in C. botulinum E and toxigenic C. butyricum. Usually, one clostridial strain produces only one type of neurotoxin and the botulinum locus is present in a single copy in the genome, as suggested by Southern blotting of DNA fragments separated by pulsed-field gel electrophoresis. However, some rare strains synthesize two different BoNTs: BoNT/A–BoNT/B, BoNT/A–BoNT/F, and BoNT/B–BoNT/F producing strains have been isolated. The A–B strain contains two bont genes related to those of C. botulinum A2 and proteolytic C. botulinum B, respectively. In such strains, the two BoNTs are usually produced in different proportions. Thus, in Ba and Bf strains, BoNT/B is produced ten times more than BoNT/A and BoNT/F. Some clostridial strains contain silent neurotoxin genes. Several C. botulinum A strains isolated from food-borne and infant botulism contain a silent bontB gene. These strains are denoted A(b). The characterization of strain NCTC2916 shows that it has two loci, A and B, which are 40 kbp distant within the chromosome. The botulinum B locus consists of bont/B, ntnh, bontR/B, ha33, and ha11 genes. bont/A is identical to the gene in C. botulinum A1 strains, but the organization of the botulinum A locus is similar to that of C. botulinum A2 and F strains. The BoNT/B nucleotide sequence is related to that of C. botulinum B strains (97% identity), but it has a stop mutation in position 128 and two base deletions (positions 2839 and 2944), resulting in reading frameshifts and multiple stop codons. Silent bont/B is also evidenced in non-toxigenic C. subterminale strains. The strain C. botulinum 667 also contains two loci, A and B, 40 kbp distant within the chromosome. The genetic organization is the same as that in strain NCTC2916 and the bont/B gene is silenced by mutations and deletions. The genes encoding for the different types of BoNT are on different genetic elements, including chromosomes, plasmids or phages depending on the species and strain of clostridia. In C. tetani and C. argentinense, the neurotoxin genes are present on a large plasmid (51 and 76 MDa respectively). Plasmids of various sizes and bacteriophages have been found in C. botulinum A, B, E, and F and previous works have shown that toxigenicity was not associated with the presence of these genetic elements. Therefore, the genes encoding for these neurotoxins were assumed to be located on the chromosome. However, it has been found that in some strains such as Loch Maree strain (subtype A3), 657Ba (type Ba and subtype A4), Okra (subtype B1), and Eklund 17B (type Bnp or B4), the botulinum neurotoxin genes are harbored by large plasmids (47 to 270 kb) (Table 7.2). Plasmid location of neurotoxin genes is common in C. botulinum type B strains, mainly in subtype B1, bivalent, and non-proteolytic strains. In the bivalent strain Ba657, the two botulinum loci, locus A and locus B, are located on the same plasmid and are separated by approximately 97 kbp. Similarly, the neurotoxin genes, bontB and bont/f, from one Bf strain are located on the same plasmid (pBf), which is closely related to pCLJ from the Ba657 strain. Interestingly, none of the botulinum plasmids show synteny to C. tetani plasmid pE88, which contains the tent gene. In C. botulinum type E and neurotoxigenic C. butyricum strains, bont/E is located mainly on the chromosome (Table 7.2). In three of 36 C. botulinum E strains, bont/E1 is located on a large plasmid. In C. botulinum C and D, it has been clearly evidenced that BoNT is encoded by genes on bacteriophages. Table 7.2 Genomic localization of botulinum loci in botulinum neurotoxin-producing Clostridia according to (Sakaguchi et al. 2005, Hill et al. 2009, Skarin et al. 2011, Hill and Smith 2013) (Franciosa et al. 2009, Brüggemann et al. 2011, Dover et al. 2013, Zhang et al. 2013, Hosomi et al. 2014, Raphael et al. 2014) ND: no data available The location of the botulinum locus within a chromosome or plasmid seems to occur not at random but at specific sites. Indeed, five specific sites of botulinum locus integration have been identified in strains from group I or II, whose genome sequencing is available. orfX–bont/A2, orfX–bont/A1, and orfX–bont/F loci are located in the ars operon, which contains three to five genes involved in arsenic reduction. orfX–bont/A1 and orfX–bont/F loci share a similar integration site at the 5′ end of the ars operon, whereas the orfx–bont/A2 locus is inserted between two copies of arsC. The ha–bont/A1 and ha–bont/B loci, which contain a recombinant ntnh gene from type A and type B strains, are found in the oppA/brnQ operon encoding for extracellular solute-binding protein and branched-chain amino acid transport proteins, respectively. This operon is lacking in non-proteolytic C. botulinum type B and C. butyricum type E strains. The third integration site is the rarA gene in group II and V strains, which contains the orfX–bont/E locus in C. botulinum type E and C. butyricum type E strains. rarA encodes a resolvase protein involved in recombination or insertion events of transposons. Interestingly, the botulinum E locus is inserted in the same codon (102) of rarA in both C. botulinum type E and C. butyricum type E strains, and the inserted botulinum locus contains an additional intact rarA gene. The trivalent strain A2f4f5 contains the orfX–bont/A2 and orfX–bont/F4 loci located in the chromosome at arsC and pulE (type II secretion system protein E), respectively. In C. botulinum F, the orfX–bont/F6 locus has been found in a new chromosomal integration site, topE. Two specific sites of botulinum locus location have been identified on plasmids from group I strains: one contains orfX–bont/A3, orfX–bontT/A4 from the Ba strain, or orfX–bontF from the Bf strain, and the second harbors the ha–bont/B locus from C. botulinum B1 strains or bivalent Ba4 or Bf strains. The ha–bont/B4 locus in non-proteolytic strains is located on a plasmid different from those of group I strains. However, the downstream flanking region of the HA–npB locus contains an IS element, a transposon-associated resolvase, and a site-specific recombinase. It is noteworthy that C. botulinum plasmids harboring bont genes such as pCLJ, pCLL, and pCDC-A3 (related to pCLK) are transferable by conjugation into a group I C. botulinum strain. Genetic analysis by 16S RNA gene sequence comparison or DNA/DNA homology has shown that C. botulinum strains form four distinct clusters which correspond to the physiological groups I to IV. AFLP and PFGE analysis also confirm the classification of proteolytic types A, B, and F strains in group I and the non-proteolytic types B, E, and F strains in group II, but can differentiate individual strains into each group. These methods have been used in epidemiological studies and are useful tools to investigate relatedness between strains isolated from patients and food. For example, among proteolytic C. botulinum strains, PFGE analysis differentiates the toxinotypes A, B, and F at an 83–86% similarity level, and enables discrimination of most individual strains. A greater diversity was observed among type A strains than among type B strains. These studies also indicate that each C. botulinum group is heterogeneous at the genome level. A high level of similarity was observed between strains from group I by using DNA hybridization with a DNA microarray including 94% of the coding sequences from strain Hall. Two type A strains share 95–96% of the strain Hall coding sequences, and seven other proteolytic strains have 87–91% common coding sequences. A larger investigation reported that 58 C. botulinum strains from group I share 63% of coding sequences with those of strain ATCC3502. Interestingly, two C. sporogenes strains (physiologically related to C. botulinum group I but non-toxigenic) are significantly similar to strain Hall and share 84–87% of the coding sequences. In another microarray study, three C. sporogenes strains show approximately 63% common coding sequences with C. botulinum A ATCC3502. The BoNT gene has been sequenced from a large number of strains, and sequence comparison has permitted identification of sequence variations in each toxinotype. Thereby, botulinum toxinotypes are divided into subtypes, which are defined as toxin sequences differing by at least 2.6% at the amino acid identity level. BoNT genes from type A strains show 92–95% nucleotide identities, corresponding to 84–90% amino acid identities, and are divided into subtypes termed A1 to A8 (Table 7.1). Subtypes A1 to A8 also differ in the botulinum locus composition. Type B genes differ from 2 to 4% at the nucleotide level and 3 to 6% at the amino acid level. They are classified into several subtypes: B1, B2, B3, B5, B6, B7, bivalent B, and non-proteolytic B. BoNT genes from non-proteolytic type B strains form only one subtype, whereas those from proteolytic strains show a greater variation, leading to a four-subtype division (Table 7.1). Sequences of neurotoxin genes in type B show less variation overall than those of type A, but a greater sequence variation is observed within members of each type B subtype compared to bont/A. BoNT/E sequences from C. botulinum type E (group II) fit into ten subtypes (E1, E2, E3, E6, E7, E8, E9, E10, E11, E12) sharing 99% nucleotide identity and 97–99% amino acid identity; they are more distantly related to BoNT/E sequences from C. butyricum strains which fall into two subtypes (E4, E5) with 97–98% nucleotide and 95–96% amino acid identities between sequences from both Clostridium species. Gene diversity has also been evidenced in the other parts of the genome, as tested by MLST and AFLP analysis, but most strains of C. botulinum E are conserved in the same clade. Subtype variation in C. botulinum E strains seems to result from recombination events rather than random mutations. Large differences (up to 25%) have been found in nucleotide sequences of BoNT/F, mainly in the region encoding the light chain, and five subtypes have been identified in proteolytic C. botulinum F versus one subtype in non-proteolytic C. botulinum F. The BoNT/F sequence from C. baratii (F7) forms a different cluster of those from C. botulinum F. The low number of strains of types C, D, F, and G that were analyzed does not permit significant evaluation of neurotoxin gene diversity. In group III, mosaic genes between BoNT genes of types C and D can be distinguished from classical strains of types C and D. The significance of sequence diversity in each toxinotype is not yet well known, but could be important in the development of diagnostic tests and therapeutic agents such as those based on immunotherapy. Therefore, BoNT/A1 and BoNT/A2, which differ by 10% at the amino acid sequence level, have large differences in monoclonal-antibody-binding affinity. Among six monoclonal antibodies that bind to BoNT/A1 with high affinity, three show a marked decrease in binding affinity (500 to more than 1000 fold) to BoNT/A2. Only combinations of monoclonal antibodies that bind tightly to toxin subtypes potently neutralize the corresponding toxin in vivo
Clostridium botulinum and Clostridium tetani neurotoxins
Introduction
Clostridia producing botulinum neurotoxins
Neurotoxin producing-Clostridium
Group I
Group II
Group III
Group IV
Group V
Group VI
Toxin type
A,
proteolytic B, F
E,
non proteolytic B, F
C, D
G
E
F
Sub type
A1, A2, A3, A5, A6, A7, A8
B1, B2, B3, B6, B7
F1, F3, F4, F5
bivalent A1(B), B5a4, Bf2, A2f4, A2f5
trivalent A2f4f5
B4 (or Bnp)
E1, E2, E3, E6, E7, E8, E9, E10, E11, E12
F6
C, D, C/D, D/C
G
E4, E5
F7
Proteolysis
+
–
–
+
–
–
Lipase production
+
+
+
–
–
–
Growth temperaturea
Optimum
Minimum
30–40 °C
10–12 °C
25–37 °C
2.5–3 °C
37–40 °C
15 °C
30–37 °C
30–37 °C
12 °C
30–45 °C
10–15 °C
Minimum pH for growtha
4.6
5
5.1
ND
4.8
3.7
Minimum water activity for growtha
0.96
0.97
0.97
0.94
ND
ND
NaCl concentration preventing growtha
10%
5%
ND
6.50%
ND
8.5
Spore thermoresistancea
D121°C = 0.21 mina
D121°C = 0.19 minb
D82.2°C = 2.4–231 min
D104°C = 0.1–0.9 min
D104°C = 0.8–1.12 min
D100°C < 0.1 min
ND
Botulism
Human
Animal (rare)
Human
Animal (rare)
Animal
Human (very rare)
ND
Human
Animal?
Human
Animal?
Related non botulinum toxin producing Clostridium
C. sporogenes
ND
C. novyi
C. haemolyticum
C. subterminale
C. proteolyticus
C. schimacherense
C. butyricum
C. baratii
Physiological properties
Group I (C. botulinum A and proteolytic strains of types B and F)
Group II (C. botulinum E and glucidolytic strains of types B and F)
Group III (C. botulinum C and D)
Group IV (C. botulinum G, also referred to as C. argentinense)
Group V (C. butyricum)
Group VI (C. baratii)
Geographical distribution of botulinum-neurotoxin-producing clostridia
C. botulinum A and B
C. botulinum E
C. botulinum C and D
C. botulinum F and G
C. butyricum
C. baratii
The botulinum locus
Genomic localization of the botulinum locus
Chromosome
Group
Type/Subtype
Botulinum locus
Insertion site
Group I
A1(B)
orfX-bont/A1
arsC operon
A2
orfX-bont/A2
ND
F
orfX-bont/F
ND
A1
ha-bont/A1
oppA/brnQ operon
A1(B)
ha-bont/B
ND
A5(B)
oppA/brnQ operon
B1, B2
ha-bont/B
ND
A2f4f5
orfX-bont/A2
orfX-bont/F4
arsC operon
pulE gene
F4
orfX-bont/F4
pulE gene
Group II
E1, E3, E9
orfX-bont/E1, 3
rarA operon
F6
orfX-bont/F6
topB
Group V
E4
orfX-bont/E4
rarA operon
Plasmid
Group
Type/Subtype
Botulinum locus
Plasmid
Size (bp)
Group I
A3
orfX-bont/A3
pCLK
266785
B5A4
ha-bont/B5
orfX-bont/A4
pCLJ
270346
B1
ha-bont/B1
pCLD
148780
B5f2
ha-bont/B5
orfX-bont/F2
pBf
ND
A2b5
ND
plasmid strain CDC1436
ND
A2f4f5
orfX-bont/F5
pCLQ
246124
B2
ha-bont/B2
pCB111
265575
F5
orfX-bont/F5
plasmid
~242000
Group II
B4 (or npB)
ha-bont/B4
pCLL
47642
B4 (or npB)
ha-bont/B4
pCB17B
47689
B4 (or npB)
ha-bont/B4
pCDC3875
58175
B4 (or npB)
ha-bont/B4
pIFR05/025
60574
E1
orfX-bont/E1
plasmid (strainCB11/1-1)
ND
E1
orfX-bont/E1
plasmid
~146000
Phage
Group
Type/Subtype
Botulinum locus
Plasmid
Size (bp)
Group III
C
ha-bont/C
C-st
185683
D, C/D, D/C
ha-bont/D, C/D, D/C
D-phage, other phages
ND
Genetic diversity of Clostridium botulinum strains and botulinum neurotoxin gene variation
Stay updated, free articles. Join our Telegram channel

Full access? Get Clinical Tree
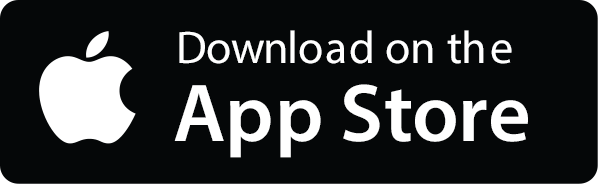
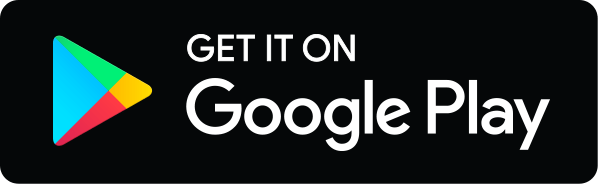