Fig. 19.1
Thin sections of mammoth tissues: (a) skin (×100), (b) muscle (×400), (c, d) bone and bone marrow (c ×100 and d ×400) samples stained by hematoxylin-eosin double staining method. There were many cell nuclei in the muscle (Fig. 19.1b). In the medullary cavity of the bone marrow there were many foam shaped structures (Fig. 19.1c) and blood cells or epithelial cells in the bone (Fig. 19.1d) (Reproduced with permission from Kato et al. 2009)
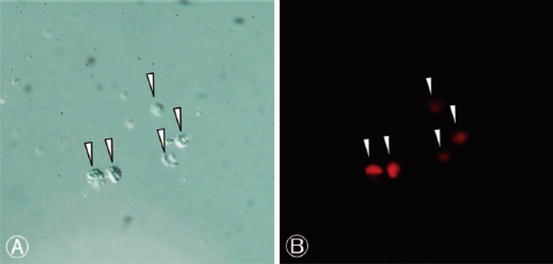
Fig. 19.2
Nuclei isolated from mammoth tissue. A – bright field; B, P.I. fluorescence. From Kato et al., 2009, with permission
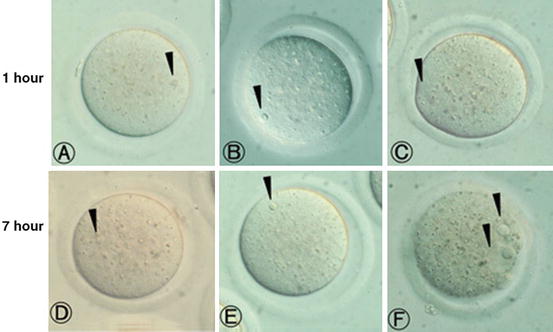
Fig. 19.3
(a–c) Mouse oocytes injected with nuclei derived from mammoth skin (a), mammoth muscle (b) and mouse bone marrow (c) at 1 h after nuclear injection. Injected nuclei were visible (arrows). (d–f) Mouse oocytes injected with nucleus derived from mammoth skin (d), mammoth muscle (e) and mouse bone marrow (f) at 7 h after nuclear injection. In d and e, injected nuclei without any change were still visible (arrows). Meanwhile, oocytes injected with mouse bone marrow derived nucleus transformed into 2 pronuclear-like structure (arrows) (Reproduced with permission from Kato et al. 2009)
This is the present state-of-the-art of mammoth cloning, impressive but not really sound. The Japanese group dealing with the mammoth cloning project is not an off-the-cuff team. This group has a robust reputation in the field of embryo manipulation and is one of the leading Japanese laboratories working on SCNT; hence the project must have some chances for success, as will be discussed in the following pages.
2.1 Cloning the Mammoth
There are two possible strategies to resurrect a mammoth:
1.
“Synthetic” genome assembly AND nuclear transfer.
2.
“Canonical” Interspecies Somatic Cell Nuclear Transfer (ISCNT).
2.1.1 “Synthetic” Genome Assembly AND Nuclear Transfer
The “Synthetic” approach relies on the recent annotation of the mammoth genomic DNA (Miller et al. 2008). Since the application of next generation DNA sequencing technologies (Metzker 2010) to ancient DNA (aDNA), the entire sequences of the mammoth genomic (Miller et al. 2008) and mitochondrial DNA (Gilbert et al. 2008) DNA have become available. Hence, we have the DNA recipe on which a new mammoth genome can be ex novo synthesized. The entire procedure has been brilliantly described by Henry Nicholls in a special Nature issue celebrating Darwin’s centenary (Nicholls 2008).
The first step is to synthesize the mammoth genome; secondly, the genome should be allocated into individual chromosomes, using the elephant genome and karyotype as a guide. This in itself is a formidable task given that elephants have 56 chromosomes. Of course the chromosomes must be canonically organized, with telomeres, centrosomes, and all vital sequences required for DNA replication and accurate segregation in mitosis; by all means an overwhelming task.
Let’s imagine we have accomplished the task of arranging the DNA into the respective chromosomes. There is a crucial organelle that is still missing: the centrosome. The centrosome is essential in cell division, therefore a centrosome, presumably obtained from elephant tissues, has to be somehow associated with the chromosome set. Obtaining purified centrosomes with subcellular fractioning through gradient centrifugation is an established procedure (Moritz and Alberts 1998), but the trouble would be to package and hold together the chromosomes and the centrosome in the mammoth-unique structure.
Given that the structure should also allow the transfer of the chromosomes/centriole into an egg, a synthetic lipid monolayer appears to be the most appropriated solution. Artificial membranes have been a reality for many years, and the state-of-the-art is particularly advanced thanks to recent development in the production of artificial cells (Zagnoni 2012). The electro-mediated fusion of the artificial chromosomes along with the centriole into an enucleated elephant egg will finalize the procedure. If all goes well, we will have mammoth/elephant hybrid embryos, with elephant mtDNA, which offers a realistic possibility for development.
In theory, this is an interesting approach. Technical problems such copying errors during DNA synthesis, which could jeopardize the reading of the artificial genome by the oocyte’s transcriptional machinery can occur and will need to be dealt with. An additional problem could be lack of communication between mammoth DNA and elephant mtDNA. The only remaining strategy left would be the generation of synthetic mammoth mtDNA, the sequence of which is available (Gilbert et al. 2008), and packaging it into artificial mitochondria. In the era of synthetic biology and artificial cells (Hammer and Kamat 2012), this might be technically feasible, but will certainly complicate the task. From a nuclear reprogramming point of view, paradoxically this might be an extraordinary possibility to improve the mammoth genome. We can actually confer upon the new DNA a structure compatibility using the reprogramming machinery of the oocyte, thus enabling complete reprogramming and hence normal development.
2.1.2 “Canonical” Interspecific Somatic Cells Nuclear Transfer (ISCNT)
In Interspecific Somatic Cell Nuclear Transfer (ISCNT) a nucleus taken from a target species is transplanted by electro-fusion or direct injection into an enucleated oocyte from a different species. ISCNT is a general term, often misused because in some reports nuclear transfer is accomplished between cell and oocyte donors belonging to different families, orders, or even classes (Loi et al. 2011).
SCNT and its variant ISCNT are potentially powerful tools for the production of unlimited numbers of offspring from a dead animal, in practice a real “asexual” reproduction, which has a tremendous appeal for multiplying endangered animals. The trouble though is that the outcome of the procedure in terms of offspring born is unpredictable and commonly low, about 1–5 %. The reason for the limited efficiency is an incomplete “nuclear reprogramming” of the differentiated nucleus. Put simply, the oocyte is unable in most cases to erase completely the differentiation memory accumulated in the form of epigenetic changes on the genome during development. Many excellent reviews are available on the topics, to which the reader is recommended (Gurdon and Wilmut 2011; Maruotti et al. 2010; Loi et al. 2008); in this chapter we would like to address exclusively the technical and biological aspects concerned with the cloning of a mammoth through a “canonical” ISCNT.
Kato’s paper (Kato et al. 2009) has demonstrated that nuclei can be isolated from mammoth tissue. The first step in our opinion would be to verify the state of preservation of mammoth DNA. It is likely that DNA will be mostly degraded after 15,000 years of permanence in the permafrost, probably worsened by cycles of thawing and freezing. The dynamics of DNA degradation over time is a constitutive branch of ancient DNA (aDNA), a relatively new scientific discipline (Hofreiter et al. 2001) launched by Svante Pääbo through his pioneer study on DNA extracted from Egyptian mummies (Pääbo 1985). The temperature and general conditions in permafrost are tolerated relatively well by the DNA, although its structural decay cannot be avoided. DNA degradation starts with deamination and depurination, followed by single and double strand breaks and deletion of large portion of the genome (Briggs et al. 2007). It is plausible that mammoth DNA, although stored in permafrost, has undergone the same kind of damage. Not all tissues however are equally exposed to damaging condition.
The best laboratory practice in aDNA for obtaining good quality DNA for sequencing studies consists of grinding compact bones and extracting DNA from the powder (Briggs et al. 2007). Bone mechanically protects cells and the DNA within from adverse conditions. Kato’s paper (Kato et al. 2009) shows that nuclei might be identified and dissected from mammoth’s tissues; hence we have a starting point for our cloning project. It is possible in fact that some, but not all, cells have a well-preserved nucleus so the first decision to be taken is how to assess genome preservation in isolated nuclei. In our opinion there are no better choices than an empirical approach using a biological assay: the transfer of the mammoth nuclei into enucleated oocytes.
The second decision is the source of oocytes. The long gone mammoth has living relatives, the elephants, particularly the Asian species; hence, our choice should be the use of Asian elephant oocytes as recipients of mammoth nuclei. This is indeed the best option, though not a problem-free route to success. To date there are no reports of oocyte collection in elephants. Attempts at superstimulation and oocyte recovery in other megaherbivores such as the rhinoceros have been reported (Hermes et al. 2009) so there is good reason to believe that the procedure, at least the oocyte retrieval part, in elephants is not insurmountable. The size of the elephant and the location of the ovaries make such a procedure technically challenging but not impossible. In elephants only one, and rarely two oocytes mature and ovulate at the end of each estrus cycle. With only three to four cycles per year (in the absence of pregnancy), the number of oocytes that each female elephant can “donate” for the mammoth cloning project is very limited (Hildebrandt et al. 2011). If thousands of oocytes will be needed, hundreds of elephants will have to be subjected to repeated oocyte collection procedures. Finding such a large number of female elephants for the procedures is probably going to be an impossible task. The entire world captive elephant population (Asian and African combined) in zoos and circuses is in the range of 1,500–2,000 animals of both sexes and of all age groups (Saragusty 2012). The alternative would be to seek captive elephants in range countries. Although the number of these throughout Southeast Asia is in the range of 15,000 animals, many are inaccessible or unsuitable (including males, immature females, and elephants in temples). Furthermore, by collecting oocytes from an elephant, we will prevent it from the possibility of becoming pregnant, thus putting the female at high risk of developing reproductive pathologies in the future (Hermes et al. 2004) and, by preventing animals from reproducing, putting the entire captive Asian elephant population at higher risk of extinction (Saragusty 2012). Once a procedure for ovarian superstimulation in elephants has been developed, this could be used in an attempt to somewhat reduce the number of elephants needed. The alternative approach would be to search for a different source of elephant oocytes. We think there are two leading options available for consideration.
In some regions of Africa, elephant populations have grown beyond the carrying capacity of the habitat in which they live. One of the measures employed by population managers is culling, often of whole family groups. When culling takes place, the ovaries can be retrieved and oocytes can be harvested. The drawbacks of this option are that, at any point in time, the vast majority of wild female elephants are not cycling either because they are pregnant or because of lactational anoestrus. The ovarian follicular reserve in African elephants is constituted almost entirely of early- and late-primary follicles (Stansfield et al. 2010) so in vitro culture and maturation protocols will need to be developed to bring the oocytes to a sufficiently mature stage to be used for the SCNT procedure. These in vitro techniques are not yet available. The other drawback of this option is that culling takes place only in Africa, so only African elephant oocytes may become available this way.
The alternative is to collect ovarian tissue slices from deceased Asian elephant cows and either maintain them in vitro or transplant them into host animals so that their circulation and hormones will support follicular growth in vivo. This has been attempted once when cryopreserved African elephant ovarian tissue slices were transplanted into nude mice (Gunasena et al. 1998). These mice supported the development of antral follicles but oocytes were generally of poor quality. As good quality oocytes will be needed for the SCNT procedures, improvements of the technique, or finding an alternative host that will give better support to follicular development, will be needed. For the number of oocytes required, a large number of immune-deficient animals will have to be maintained at very high costs. Once harvested, the good quality oocytes will still need to be matured so an in vitro maturation protocol will need to be developed. Despite all foreseen and unforeseen difficulties, we think that this approach stands a better chance of success.
Regardless of the approach taken, we think that it would be best to restrict the number of elephant oocytes needed to the very minimum, given the technical difficulties and the high costs involved in collecting and maintaining them. The probability of finding nuclei bearing intact DNA in a mammoth tissue is not very high, so a large number of oocytes, most probably in the order of thousands, will have to be injected with isolated nuclei to find the very few that might be reactivated and start development. As an alternative approach, we propose the use of easily available oocytes for the first round of cloning. A potential candidate for oocyte donation might be the bovine. The state-of-the-art of in vitro maturation and culture in cattle is the most advanced amongst all farm animals (Lonergan 2007), and a large number of oocytes can be conveniently collected from ovaries taken from slaughtered cows. Objections, however, can be raised against this option as it clashes with the established concept of ISCNT, namely genomic/mtDNA compatibility and the high probability of Zygotic Genome Activation (ZGA) failure in the “extreme” mammoth/bovine hybrid embryos (Loi et al. 2011).
3 Genomic/mtDNA Incompatibility in ISCNT
Mitochondrial DNA codes for proteins responsible for the production of cellular energy. Given the low fidelity of the mtDNA replication machinery, some of the mitochondrial crucial genes are secured in the nucleus where they are safely duplicated and expressed (Amarnath et al. 2011). Therefore, a coordinated mt/genomic DNA cross talk is necessary for normal embryo development. Mammoth/bovine hybrid cloned embryos have no chance of developing normally, but early cleavages might occur since energy production and mtDNA replication do not occur before the blastocyst stage (Thundathil et al. 2005).
Following our suggested approach, successfully cleaving cloned embryos will be used at the morula or even 4–8-cell stage, before the unavoidable ZGA failure, for a second round of cloning, but this time using elephant oocytes. The role of the first round of nuclear transfer is to probe a large number of mammoth nuclei, and select those with a genome capable of directing embryonic development. The second round of nuclear transfer will transfer the mammoth nuclei into elephant cytoplasm, where an appropriated mt/genomic DNA cross talk, as well as a successful activation of the mammoth “embryonic genome” will probably take place. Technically it is very easy. The blastomeres of the cloned embryo will be disaggregated and electro-fused into enucleated elephant oocytes, essentially, the standard nuclear transfer procedure for embryonic cells.
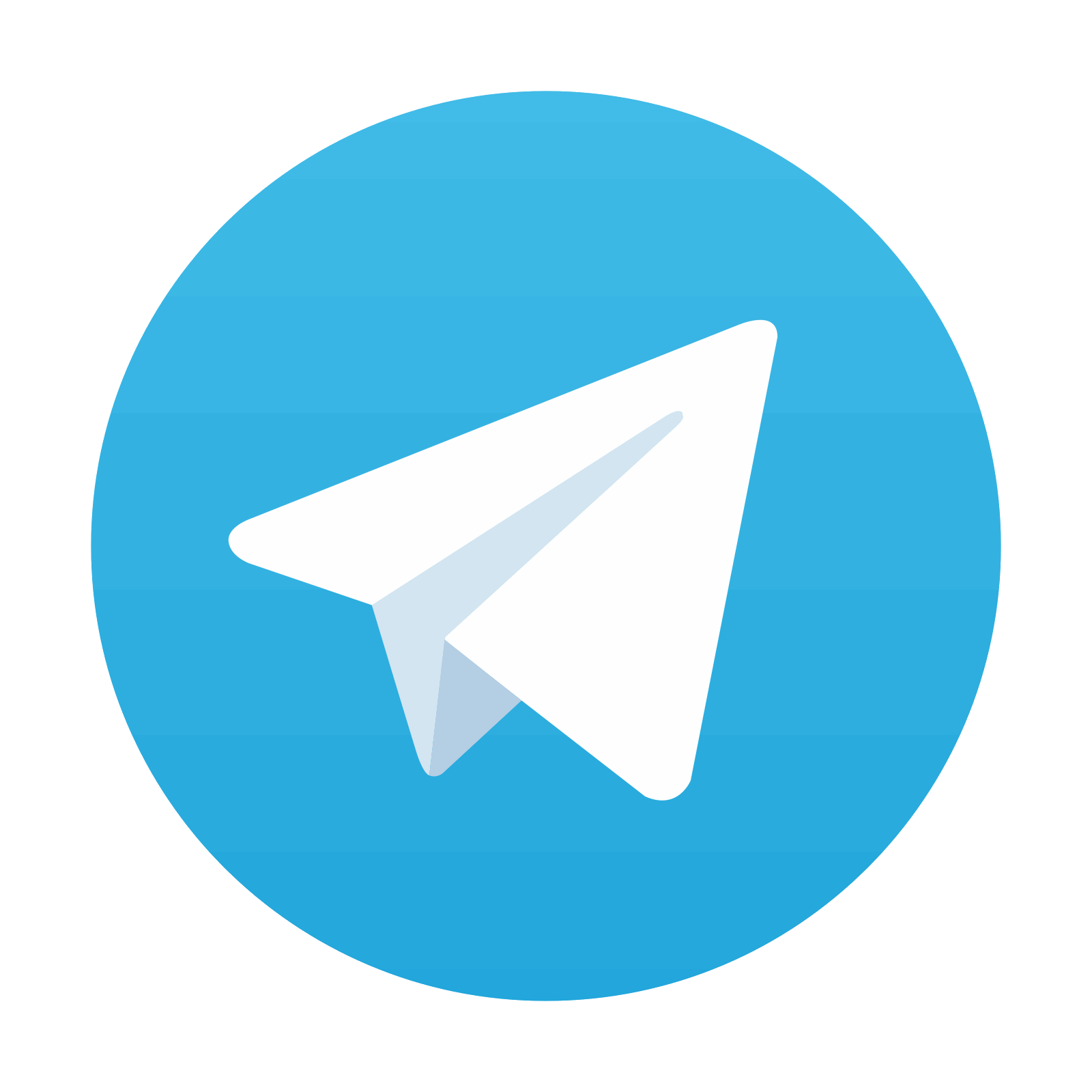
Stay updated, free articles. Join our Telegram channel

Full access? Get Clinical Tree
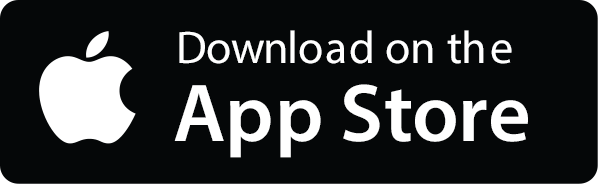
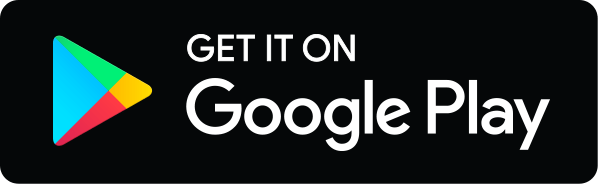