Chapter 6
Clinical Pathology
Barbara von Beust
Independent consultant, Winterthur, Switzerland
Clinical pathology is the study of diseases in animals by examination of blood, tissues and fluids; in the context of preclinical safety, clinical pathology focuses primarily on in-life variables, in contrast to anatomic pathology, which is the gold standard for end-point assessment. The most common tissue to use for the in-life assessment of test article-related effects is blood and its components, and this chapter will thus explain in detail the characteristics of this relatively easily accessible and dynamically changing, life-supporting fluid in traditional clinical pathology. Other body fluids, such as urine, cerebrospinal fluid and bronchoalveolar lavage specimens can also be subject to clinical pathologic investigation, which in general measures analytes and other variables (Tomlinson et al., 2013). A compilation of nontraditional clinical pathology applications can be found in Jordan et al. (2014).
Other in-life variables that are important for the assessment of test article-related effects are very basic, and include food consumption and body weight gain or loss. Organ weights can also provide valuable information about the effect of a compound on a tissue at the time of the study end point.
6.1 Clinical Pathology in Study Phases and Good Laboratory Practice
The collection of clinical pathology data represents an integral part of a study. It requires not only careful planning (including the scheduling of blood sampling time points and the provision of recommended volumes and variables), but also a professional and competent laboratory in which to perform the analysis, including quality control and assurance. The evaluation of the resulting data is best performed by experts trained in all aspects of clinical pathology, and in general, it is recommended that study personnel consult or appoint a specialist veterinary clinical pathologist for this purpose (Tomlinson et al., 2013).
6.1.1 Preanalytic Phase: Study Plan
The preanalytic phase includes the overall planning of a particular study, the determination of the animal species, sex, number of animals and schedule of blood samplings, as well as the list of variables to be measured and the equipment to be used (Hall and Everds, 2003; Jordan et al., 2014; Braun et al., 2015). All aspects of the preanalytic phase may influence the validity and scientific meaning of the collected data (Gunn-Christie et al., 2012; Vap et al., 2012). For instance, excessive blood sampling from small species such as mice, rats and even monkeys will result in a compensatory response from the bone marrow to replace the removed blood volume. This can affect the statistical relevance of data analyses (e.g. standard haematology variables such as red blood cell mass, including reticulocyte numbers and bone-marrow myeloid (granulocytes)-to-erythroid (red blood cells) (M : E) ratio (NC3Rs, 2016). Anaesthetising for blood sampling can also affect clinical pathology results (Bennett et al., 2009).
Nonstandardised blood-sampling methods and/or blood sampling by inadequately trained staff can result in artefacts such as haemolysis (the rupturing of red blood cells and the release of their contents into surrounding fluid), which can affect entire data sets, and introduce animal stress-related changes, which can result in variations in some variables (e.g. leukocyte counts), again affecting data interpretation (Gunn-Christie et al., 2012; Vap et al., 2012).
Some artefacts that can be introduced during the preanalytic phase (e.g. due to partial clotting of samples that were difficult to collect from very young or small animals, inadequate aspiration technique (leading to haemolysis), the use of inappropriate tubes) resulting in incorrect sampling ratios between anticoagulant and blood volume (Gunn-Christie et al., 2012). The most important thing is to correctly document any incident that could potentially cause skewed clinical pathology data later on.
It is recommended that study personnel involve the clinical pathologist in the review of the final study plan. This should include details such as the total allowable blood volume to be removed per animal by the end of the treatment phase, the types of tubes used for blood collection, the most scientifically meaningful profile of analytes for the purpose of the study, and acceptable storage and transportation of samples (ambient temperature versus refrigerator, time until separation of serum, etc.). In addition, standard procedures, such as randomisation of the blood-sampling order and fasting of the animals, should be discussed and defined before initiating the study (Tomlinson et al., 2013).
In general, whole blood is collected in plain serum tubes (serum is the colourless fluid produced after centrifugation of clotted blood) for use in creating biochemical profiles. In some environments, the preferred practice is to collect blood into tubes containing lithium heparin, and to determine the biochemical profile on heparin plasma (plasma is the colourless fluid produced after centrifugation of blood and anticoagulant). There are likely small differences between serum and heparin plasma (with the exception of fibrinogen in plasma), but the validation method and reference intervals should be established on the relevant matrix. Matrix includes all the properties of blood components in a particular species that can affect test performance, e.g. serum colour can affect photometric read-out. Usually, this is a nondefined effect, but it will result in biased results when comparing a standard with a particular species-specific specimen. For haematology profiles, the preferred anticoagulant is ethylenediaminetetraacetic acid (EDTA). For coagulation tests, it is citrate.
If the use of special kits designed for human use is planned (e.g. for biomarkers), the laboratory should be allowed sufficient time to properly validate such assays (Jensen and Kjelgaard-Hansen, 2006), and pretreatment blood samples should be scheduled to allow adequate interpretation of potential individual test article-related changes.
6.1.2 Analytic Phase: Data Generation
The analytic phase includes the actual generation of clinical pathology data (i.e. the measurement of all variables and analytes) (Tomlinson et al., 2013). This phase also includes proper laboratory management practices, including method validation and the regular (e.g. daily, monthly) quality control of all applied testing methods and instruments (Flatland et al., 2014; Camus et al., 2015). The personnel performing the analytic phase in preclinical studies should have adequate training on all the instruments they operate, as well as on the interpretation of instrument flags (error messages) and their follow-up (e.g. evaluation of blood smears in haematology, or trouble-shooting of coagulation tests via understanding of the coagulation cascade, including the intrinsic, the extrinsic and the common pathways) (Tomlinson et al., 2013).
Although some of the instruments developed for clinical pathology are advertised by their manufacturers as being very user-friendly, they still require adequate training of the personnel operating them.
6.1.3 Postanalytic Phase: Data Interpretation and Reporting
The postanalytic phase consists of the actual assessment and reporting of all measured variables (Tomlinson et al., 2013). The individual assessing clinical pathology data should be trained in the recognition of potential artefacts or laboratory errors, and should have a good understanding of the physiologic, pharmacologic and pathologic context of test item-related changes of toxicologic significance.
The storage of leftover samples is a very important component of postanalytic management; this includes freezer management and the keeping of detailed freezer logs. Leftover samples are important not only as back-up for confirmation of questionable results or the addition of new variables, but also for validation purposes (Flatland et al., 2014).
In summary, a high degree of standardisation, as reflected in carefully updated standard operating procedures (SOPs), helps minimise artefacts and variability, allowing for efficient and scientifically meaningful data interpretation. Clearly, the three phases of clinical pathology are very much interdependent, and they must be coordinated accordingly, ideally with the input of a certified clinical pathologist (Figure 6.1) (Tomlinson et al., 2013).

Figure 6.1 The three phases in clinical pathology.
6.1.4 Good Laboratory Practice
Good Laboratory Practice (GLP) standards apply to the clinical pathology laboratory. SOPs should be established for all routine procedures. Importantly, a large amount of raw data are generated in a clinical pathology facility. The handling of these data includes transfer, tabulation, statistical analysis and final archiving, all of which must follow validated procedures. This includes the validation of any laboratory informatics management systems (LIMS) and software. Details can be found in FDA (2015).
6.2 What is Measured in Clinical Pathology?
Blood is composed of a water base, which is termed ‘plasma’ or ‘serum’ depending on the separation of anticoagulated (plasma) or clotted (serum) blood from its cellular components (Figure 6.2). Serum is the main matrix for the measurement of the concentration of chemical variables (e.g. electrolytes, minerals and metabolites) and enzyme activities. Many of these analytes can provide information on the rapidly changing physiology or pathology of certain tissues and organs (e.g. heart, liver, kidney). In contrast, the cellular blood components (i.e. the red and white blood cells and platelets (thrombocytes)) are evaluated based on counts in whole anticoagulated blood and their morphologic appearance in a blood smear (Figure 6.2). Increased or decreased blood cell numbers can provide valuable information on potential test article-related effects such as blood loss, inhibition of haematopoiesis (blood cell formation in bone marrow) and inflammatory reactions. In addition, changes in morphology can point to disturbed maturation in the bone marrow or metabolic effects (e.g. phospholipidosis). Tables 6.1–6.4 provide a list of standard variables that should be evaluated in toxicologic studies (Tomlinson et al., 2013). Notably, there are some species-specific differences. For instance, in rats and dogs, increased alanine aminotransferase (ALT) and aspartate aminotransferase (AST) activities are indicative of potential hepatotoxicity, whereas in pigs, increased sorbitol dehydrogenase (SDH) activity is more specific for hepatotoxicity (Tomlinson et al., 2013).

Figure 6.2 The main materials for toxicologic clinical pathology testing are anticoagulated whole blood for haematology (e.g. in EDTA), serum collected after centrifugation of clotted whole blood for clinical chemistry and Wright–Giemsa-stained blood smears for microscopic evaluation and validation of quantitative and qualitative aspects of red and white blood cells and platelets. The arrow points to the feathered edge, where platelet clumps or atypical cells can preferentially be seen.
Table 6.1 Recommended haematology variables for routine toxicology studies
Variable | Unit |
Red blood cell mass | |
Haematocrit | % |
Haemoglobin concentration | g/l |
Red blood cell count | 1012/l |
Indices | |
Mean cell volume (MCV) | fl |
Mean cell haemoglobin (MCH) | pg |
Mean cell haemoglobin concentration (MCHC) | g/l |
Reticulocyte count | |
Red cell distribution width (RDW) | % |
Total white blood cell count | 109/l |
Differential white blood cell count (absolute counts only) | |
Neutrophils | 109/l |
Lymphocytes | 109/l |
Monocytes | 109/l |
Eosinophils | 109/l |
Basophils | 109/l |
Large unclassified cells (LUCs) | 109/l |
Table 6.2 Recommended coagulation variables for routine toxicology studies
Variable | Unit |
Prothrombin time (PT) | seconds |
Activated partial thromboplastin time (APTT) | seconds |
Platelet count | 109/l |
Fibrinogen | mg/l |
Table 6.3 Recommended serum chemistry analytes for routine toxicology studies
Analyte | Unit |
Enzymes | |
Aspartate aminotransferase (AST) | U/l |
Alanine aminotransferase (ALT) | U/l |
Sorbitol dehydrogenase (SDH) (minipigs) | U/l |
Glutamate dehydrogenase (GLDH) (minipigs) | U/l |
Alkaline phosphatase (ALP) | U/l |
γ-glutamyltransferase (GGT) (rodents) | U/l |
Creatine kinase (CK) | |
Metabolites | |
Total bilirubin | µmol/l |
Glucose | mmol/l |
Urea | mmol/l |
Creatinine | µmol/l |
Triglycerides | mmol/l |
Total cholesterol | mmol/l |
Total protein | g/l |
Albumin | g/l |
Albumin : globulin ratio | g/g |
Electrolytes | |
Sodium | mmol/l |
Potassium | mmol/l |
Chloride | mmol/l |
Total calcium | mmol/l |
Phosphorus | mmol/l |
Table 6.4 Recommended urinalysis variables for routine toxicology studies
Variable | Unit |
Volume | ml |
Colour | Macroscopic description |
Clarity | Macroscopic description |
Specific gravity | g/g |
pH | |
Glucose | −, +, ++, +++ |
Protein | −, +, ++, +++ |
Blood | −, +, ++, +++ |
Ketones | −, +, ++, +++ |
Bilirubin | −, +, ++, +++ |
6.2.1 Interference by Haemolysis, Lipaemia and Icterus
Haemolysis (ruptured red blood cells), lipaemia (fat molecules in the blood) and icterus (yellow discolouration of tissues due to high blood bilirubin levels) are relatively common changes that can be either incidental or test article-related. They are visible as colour changes in plasma or serum after centrifugation of coagulated or anticoagulated blood (Figure 6.3). Such colour changes should always be carefully documented, as they can provide important information on either preanalytic issues or analytic concerns. For instance, haemolysis due to suboptimal blood-sampling practices requires a change in blood-sampling protocols, whereas haemolysis due to a test article-related effect (e.g. combined with a reduced red blood cell mass) requires in vitro haemolysis testing. Lipaemia may result from food consumption shortly before blood sampling; this requires fasting procedures prior to blood sampling. Alternatively, test article-related lipaemia can be indicative of metabolic changes. Finally, icterus is indicative of increased bilirubin concentrations (e.g. as a consequence of marked haemolysis or potential hepatobiliary dysfunction). Haemolysis, lipaemia and icterus can all interfere with some analytic tests. Each laboratory should establish its own list of potential interferences based on the assays it is using.

Figure 6.3 Microhaematocrit tubes with haemolysis, lipaemia (small fat layer after centrifugation; arrow) and marked icterus in the plasma layer. Such discolourations can be accidental or treatment-related and can interfere with analytical measurements.
Source: Courtesy Dr Ilse Schwendenwein, University of Veterinary Medicine Vienna
.6.3 Haematology
Haematology primarily evaluates the cellular components of blood for their qualitative and quantitative properties. Qualitative or morphologic aspects include the recognition of maturation status (e.g. polychromasia: different-coloured red blood cells; left shift: release of immature neutrophils), signs of toxic change or activation (e.g. in neutrophils, lymphoblasts, large unclassified cells (LUCs)) and the presence of other morphologic changes (e.g. intracytoplasmic vacuoles in phospholipidosis) in the different cell populations. There are three main cell populations, which also represent the developmental lineages originating in the bone marrow: red blood cells or erythrocytes, white blood cells or leukocytes and platelets or thrombocytes.
For more detailed information concerning any morphologic or quantitative aspect of blood cells, it is recommended to consult a standard veterinary haematology reference, such as Weiss and Wardrop (2010).
6.3.1 Manual and Automated Techniques in Haematology
Over the last decade, automated haematology analysers equipped with species-specific software for most of the species used in preclinical studies have become standard in most laboratories. Although these instruments provide reliable and consistent results, errors due to preanalytic incidents or biologic aberrations require the operating personnel to be familiar with traditional manual haematology practices; they must be able to confirm atypical results indicated by instrument flags or error warnings by assessing red and white blood cell counts by alternative means, and to evaluate blood smears for morphologic changes, including common artefacts such as platelet clumps.
6.3.2 Haematocrit and Red Blood Cell Mass
The haematocrit, or packed cell volume (PCV), is a quantification of the cellular components relative to the liquid phase of whole or anticoagulated blood (Figure 6.3). The proper term for instrument-generated data is ‘haematocrit’, which is extrapolated from flow cytometrically determined, mean cell volume (MCV) and red blood cell counts. Manually, the PCV is determined in a microhaematocrit tube after centrifugation to separate the cells from plasma (Figure 6.3). As a more functional unit, the red blood cell mass may include all variables providing information on the total oxygen-carrying capacity of peripheral blood, including haematocrit/PCV, red blood cell count (including reticulocyte count), haemoglobin concentration and the indices (see Section 6.3.3.1). A reduction in red blood cell mass is usually accompanied by a decrease of haematocrit/PCV, red blood cell count and haemoglobin concentration, while reticulocyte count and indices can vary according to the origin and pathophysiology of the reduction.
6.3.3 Blood Cells
6.3.3.1 Red Blood Cells
Mammalian red blood cells are biconcave, discoid anuclear cells that transport haemoglobin – the protein responsible for binding oxygen in the lung and transporting it to the tissues. Oxygen binding is mediated by iron, a potentially highly oxidative and toxic element contained inside the porphyrine ring, which is held in position by four globin molecules. A basic understanding of haemoglobin synthesis and function can be of relevance when evaluating test article-related effects on red blood cell mass in many different settings. For more details, see Weiss and Wardrop (2010).
In mature animals, red blood cells originate in erythropoietic islands in bone marrow, which are present in the marrow cavity of trabecular bone. The earliest precursor cell, the erythroblast, is a large basophilic cell with a large amount of dark-blue cytoplasm and a large nucleus with a nucleolus. Transferrin receptors are expressed on maturing erythroid precursor cells, allowing the transfer of iron and the synthesis of haemoglobin, which results in an increasing orange-red colouration of the cytoplasm in routine stains of the subsequent stages of red blood cells, which also decrease in size with advanced maturation and haemoglobination. At the same time, the nucleus condenses continuously until only a small, compact, round, dark-blue or black apoptotic body remains inside the metarubricyte. Shortly before the reticulocyte or polychromatic immature red blood cell is released into the peripheral blood circulation, the apoptotic nucleus is expelled and phagocytised by macrophages. Immature red blood cells may still contain some remnants of RNA, giving them a slightly bluish tinge (polychromasia) in routine stains (Figure 6.4). The term ‘reticulocyte’ comes from the appearance of such young red blood cells after staining with new methylene blue, which demonstrates a network of ribosomes, appearing as a reticular or network pattern. Examination of new methylene blue-stained blood smears is the traditional method for assessing reticulocytes (Figure 6.4) (Weiss and Wardrop, 2010).

Figure 6.4 Increased numbers of reticulocytes indicate active haematopoiesis in the bone marrow, a physiologic response to blood loss due to moderate or excessive blood sampling, haemorrhage or haemolysis. (a) Traditionally, reticulocytes are visualised by vital stains, such as new methylene blue, which make the reticular pattern of remnant ribosomes and RNA visible. (b) In the haematology analyser, reticulocytes are identified by their remnant RNA (in the absence of a nucleus) (x-axis), with most immature reticulocytes on the far-right side. (c) In a Wright–Giemsa-stained blood smear, younger red blood cells are characterised by a larger diameter (anisocytosis) and the lack of a central pallor, as well as a slightly bluish tinge, termed ‘polychromasia’. (d) In the analyser, these cells typically have a higher volume (y-axis) and a lower haemoglobin concentration (x-axis). In a very pronounced response, there can actually be two populations of red blood cells, with normal and lower haemoglobin concentrations (x-axis).
Source: Courtesy Dr Ilse Schwendenwein, University of Veterinary Medicine Vienna.
In cases of acute need, such as following acute haemorrhage or intense blood-sampling regimens, the release of immature red blood cells can be accelerated such that increasing numbers of reticulocytes or polychromatic cells, or even nucleated red blood cells (nRBCs) or metarubricytes, will be visible in blood smears. In addition, the mean cellular volume (MCV) will be increased, and red cell distribution width (RDW) will be enlarged or even biphasic, because the immature precursors released in response to the increased demand will be larger than the mature erythrocyte (Figure 6.4).
Modern haematology analysers report reticulocytes based on the amount of stainable cytoplasmic RNA in absolute and relative numbers (as a percentage of total red blood cells). In addition, nRBCs are reported. Typically, regenerative haematology profiles are accompanied by an increased MCV, manifesting as anisocytosis (variation in cell size) in the blood smear (as immature red blood cells are slightly larger, and thus there are erythrocytes of different sizes present), which in a toxicologic clinical pathology context is usually considered a normal physiologic response to blood loss (Figure 6.4).
The size and haemoglobin content of individual red blood cells is assessed by the indices: the MCV, the mean cell haemoglobin (MCH) and the mean cell haemoglobin concentration (MCHC). More recently, a new variable, the RDW, is also being reported by modern haematology analysers. This represents a histogram of the frequency distribution of the differently sized, red blood cells. In regenerative red-blood-cell profiles, the MCV and RDW will typically be increased, due to the appearance of larger (macrocytic) immature red blood cells (Figure 6.4). Depending on the availability of iron for haemoglobin synthesis, MCH and MCHC can be slightly lower, especially in cases of chronic ongoing inflammatory processes (e.g. anaemia of chronic disease).
If the location of blood loss is not evident from either in-life observations or excessive blood sampling for analytic purposes, coagulation profiles should be checked for a potential explanation (e.g. coagulation disorder causing bleeding and blood loss).
6.3.3.2 White Blood Cells
White blood cells are responsible for antigen-specific (lymphocytes) and -nonspecific (granulocytes, monocytes) defences against infectious pathogens. While granulocytes contain cytoplasmic granules with cytotoxic and vasoactive compounds (peroxidase, histamine and many others), they are also capable of phagocytosis of bacteria – which is also the main function of activated monocytes or macrophages. The numbers, migration and actual physiology of white blood cells in blood and tissues are regulated by complex networks of messenger molecules (i.e. cytokines and immunoregulatory networks), which can be modulated by treatments (particularly biologicals), leading to test article-related changes in leukocyte numbers in the absence of infectious pathogens. Importantly, test article-related effects concerning the absolute and differential numbers of white blood cells may not be apparent in the histopathology analysis, and thus clinical pathology data may provide the only indication of a treatment effect.
There are three types of granulocytes: the neutrophil, eosinophil and basophil. All granulocytes are characterised by lobulated or convoluted to segmented nuclei with a moderate amount of cytoplasm, which contains different types of granules that either do not stain (neutrophil) or take on a pink (eosinophil) or purple to blue (basophil) colour after staining with standard Romanowsky stains – hence the descriptive terminology (Figure 6.5). The predominant granulocyte is the neutrophil, while the eosinophil can be relevant in particular immunologic settings, such as allergic reactions and parasitic infections (particularly in non-human primates). The number of eosinophils is typically lower in the presence of an adrenocortical stress reaction (see later), while the neutrophil count will be higher (Bennett et al., 2009; Everds et al., 2013). The basophil is in general of no relevance in toxicologic clinical pathology, but may parallel changes in eosinophil numbers. Like red blood cells, the granulocytes originate in the bone marrow, where they undergo a maturation process from the early myeloblast with an agranular cytoplasm and a large nucleus with a nucleolus to the mature segmented granulocyte with granular cytoplasm and a segmented condensed nucleus. In times of increased need, such as under the influence of certain cytokines, severe inflammation or other mediators, a left shift – characterised by the premature release of band neutrophils – can be seen (Figure 6.5). By definition, the nucleus of the band neutrophil displays parallel outlines and no constrictions, as seen in the mature neutrophil. Changes in neutrophil counts can happen relatively swiftly due to either release from the wall of blood vessels during stress, extravasation to tissues due to chemotaxis or rapid release from bone marrow.

Figure 6.5 (a–e) dog; (f) cat. Leukocytes and platelets in canine blood smear. Wright–Giemsa, x100 objective (a–e). (a) Segmented neutrophil with pale cytoplasm and an irregular convoluted and knobby condensed nucleus (left); monocyte with slightly basophilic/bluish cytoplasm and an irregular shaped nucleus with a reticulated chromatin pattern (right). (b) Eosinophil with large eosinophilic cytoplasmic granules and an irregular ribbon-shaped nucleus, (c) Basophil with numerous purple cytoplasmic granules and an irregular lobulated nucleus. (d) Lymphocyte with a small rim of deeply basophilic/blue cytoplasm and a dense bean-shaped nucleus. (e) Segmented (right) and band (left) neutrophil with pale cytoplasm and the typical band-shaped nucleus. (f) Platelet aggregates ×20 objective.
Source: Courtesy Dr Ilse Schwendenwein, University of Veterinary Medicine Vienna.
Lymphocytes are produced in the bone marrow, but also originate in the thymus in growing animals and in the lymph nodes and other secondary lymphoid tissue in the body (e.g. mucosal-associated lymphoid tissue (MALT), gut-associated lymphoid tissue (GALT)). Lymphocytes generally appear as round cells with a small rim of basophilic cytoplasm and usually round nuclei without visible nucleoli. They are slightly smaller than granulocytes, but larger than red blood cells (Figure 6.5). After stimulation of the immune system, either by cytokines or by immune-modulating compounds, activated lymphocytes can transform into lymphoblasts, which are characterised by increased cellular and nuclear size, and sometimes by visible nucleoli. Lymphocyte numbers can be decreased under the influence of an adrenocortical stress reaction, which is usually accompanied by a decrease in the size and weight of immunologic organs (Everds et al., 2013).
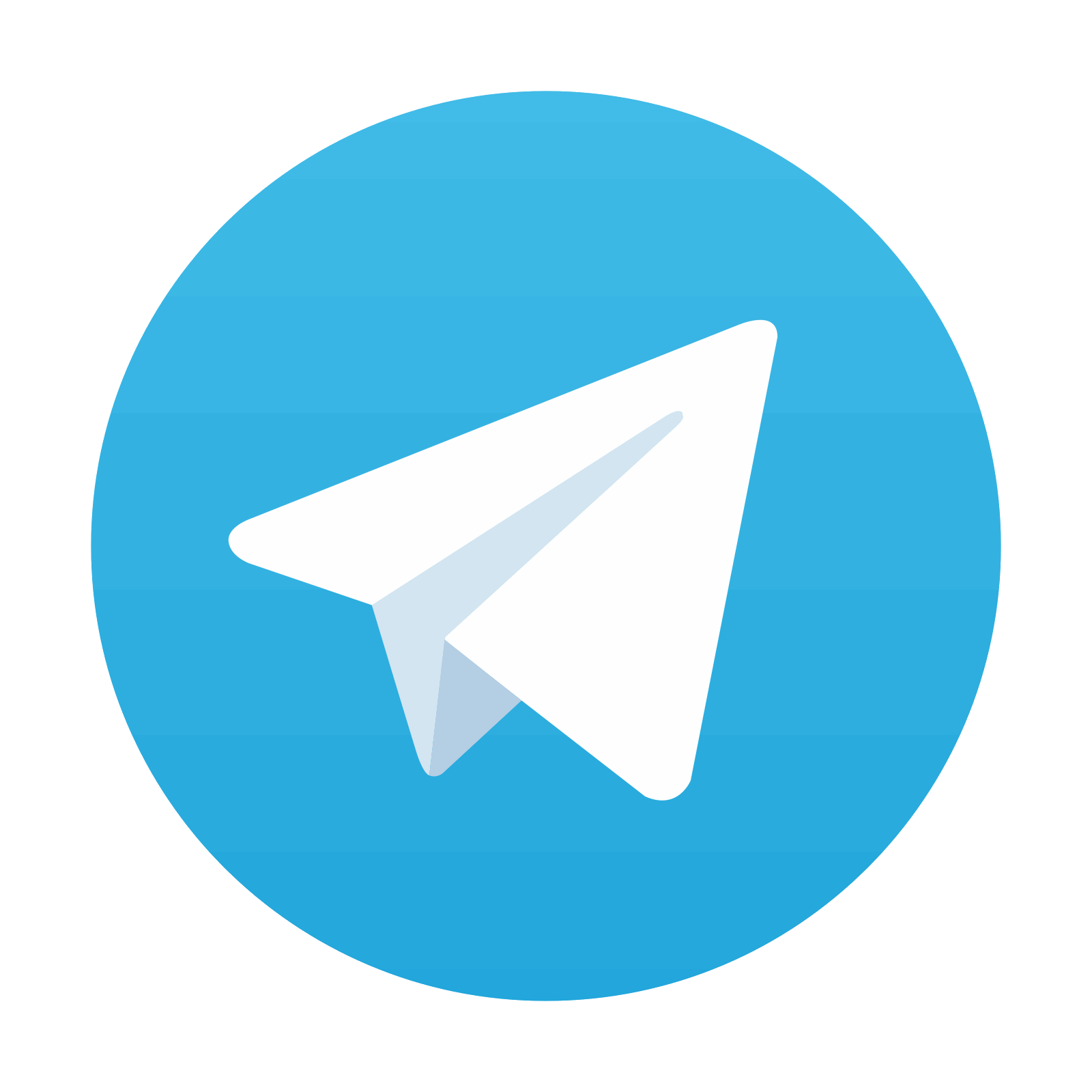
Stay updated, free articles. Join our Telegram channel

Full access? Get Clinical Tree
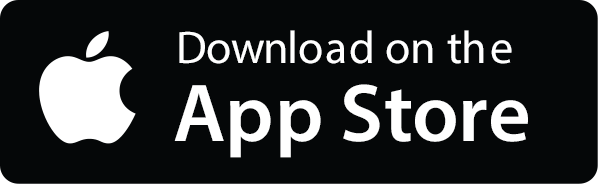
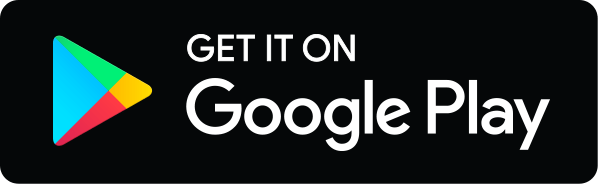