Fig. 13.1
Schematic diagram explaining the food-entrainable oscillator (FEO) and food-entrainable peripheral oscillator (FEPO). Light signals can entrain the light entrainable oscillator (LEO) by activating the suprachiasmatic nucleus (SCN). On the other hand, scheduled feeding can entrain the FEO or FEPO. The FEO produces food anticipatory activity (FAA), which appears 2–3 h before food timing. The FEPO produces phase entrainment of clock gene expression rhythms and clock-regulated metabolic gene expression rhythms in peripheral tissues
Drugs also have the power to entrain circadian systems. Some drugs such as benzodiazepines and melatonin receptor agonist can entrain circadian rhythms. Many chemical compounds such as CK1 inhibitors and GSK3B inhibitors have been reported to affect the period of circadian rhythms. CK1 inhibitors prolong the circadian period, and GSK3b inhibitors such as lithium shorten it. On the other hand, the circadian clock influences the main effects and side effects of some drugs. Because many proteins or receptors have transcriptional or translational circadian rhythms, drug administration times should be considered in order to maximize the desired effects of the drug and reduce side effects. For example, antiasthmatic drugs are preferably taken early in the morning because airway obstruction is high during this time. As another example, antihyperlipidemia drugs such as statins are ideally taken early in the evening because these drugs inhibit HMG CoA reductase, the rate-limiting cholesterol synthase enzyme, whose activity is high during this time. Thus, in the past decade, scientific study of the connection between the circadian clock and pharmacology, chrono-pharmacology, has become a common medical research field.
Similarly, there are interactions between nutrients and the circadian system, which we call chrono-nutrition (Fig. 13.2). As an example of chrono-nutrition, SF can act as a signal to entrain the circadian system in rodents. The effect of food entrainment in humans is still unknown because of the lack of reports to date. However, eating times may function as an entrainment factor in humans. Because there are circadian variations in metabolic gene expression throughout the day, the food content and meal timing may affect health. Late night dinners increase fat synthesis and phase-shifted clock gene expression rhythms in peripheral clocks. Just as bright light exposure therapy in the morning has become a common treatment for circadian sleep disorders or seasonal affective depression, food-timing therapy may become a common therapy for many circadian disorders.
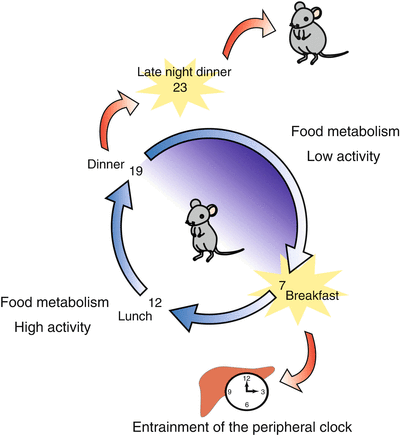
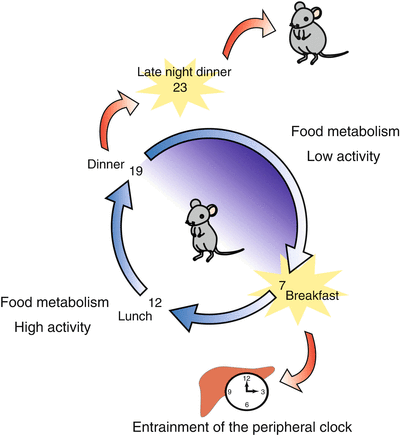
Fig. 13.2
Schematic diagram explaining chrono-nutrition. Chrono-nutrition is a new field of study, like chrono-pharmacology. There are two aspects. (1) Time-restricted food access can be an entrainable factor for the circadian system. (2) Peripheral clocks regulate day–night differences of clock-regulated metabolic genes. Therefore, food timing is an important factor for maintaining health
13.2 Mechanism of the Food-Entrainable Oscillator in the Brain
13.2.1 Role of the Dorsomedial Hypothalamus in the FEO
Neural centers in the hypothalamus are putative sites of the FEO in the brain. These centers are central to the regulation of energy homeostasis and arousal; they include the arcuate nucleus, paraventricular nucleus, dorsomedial hypothalamus (DMH), and ventromedial hypothalamus (Cone et al. 2001; Rodgers et al. 2002; Saper et al. 2005a, b; Ribeiro et al. 2007; Gooley et al. 2006). Daily SF can entrain the peripheral circadian and cerebral cortex clocks. In contrast, the SCN clock uses the light–dark (LD) cycle as the entraining stimulus (Castillo et al. 2004); it cannot be entrained by SF conditions. SF-induced entrainment of the peripheral clock was observed under constant lighting with ablation of the SCN (Damiola et al. 2000; Hara et al. 2001; Stokkan et al. 2001; Wakamatsu et al. 2001). After starvation, re-feeding increased Per1 and Per2 gene expression in the DMH of mice (Moriya et al. 2009; Mieda et al. 2006). These studies suggest that the DMH may be the brain region responsible for FAA formation. In other experiments, behavioral FAA rhythm persisted in rats (Landry et al. 2006, 2007) and mice (Moriya et al. 2009; Tahara et al. 2010) with lesions in the DMH. However, Gooley et al. (2006) reported that a cell-specific lesion in the DMH blocked SF-induced FAA and body temperature rhythms in rats. Therefore, the role of the DMH in FAA maintenance remains controversial. In a recent study, we demonstrated that a relatively large lesion in the medial basal hypothalamus (MBH) attenuated FAA, suggesting that MBH regions, including the DMH, arcuate nucleus, and ventromedial hypothalamus, may be involved in the formation of FAA (Tahara et al. 2010).
13.2.2 Role of Limbic Brain Areas in the FEO
SF shifts the phase of clock gene mRNA expression in the cerebral cortex and hippocampus as well as PER2 protein levels in the bed nucleus, stria terminalis, amygdale, and dentate gyrus of mice (Wakamatsu et al. 2001; Verwey et al. 2007; Shibata et al. 2009). Limited daily access to sucrose or saccharine in freely fed rats or scheduled access to saline in sodium-deprived rats had no effect on PER2 rhythms (Waddington Lamont et al. 2007). These data suggest that PER2 rhythms in the limbic forebrain are sensitive to signals that arise from the alleviation of a negative metabolic state associated with SF. Therefore, access to rewarding substances in the absence of food deprivation or metabolic challenge is not sufficient to alter the rhythm of PER2 protein in the limbic forebrain (Amir and Stewart 2009). Gestation appears to play some role in the protein level of PER2 because rats and hamsters entrain to the presence of palatable foods and demonstrate FAA even when regular rodent chow is available ad libitum (Mendoza et al. 2005).
13.2.3 Role of Other Brain Areas in the FEO
Ablation of sensory inputs that carry gustatory and satiety sensation appears to influence circadian FAA (Mistlberger 1994) because FAA is attenuated in the ghrelin receptor-deficient mouse (Lesauter et al. 2009). Destruction of the hindbrain parabrachial region, which processes visceral and gustatory information, also attenuates FAA (Davidson et al. 2000). These data suggest that the biological clock within the SCN that regulates circadian patterns of appetite and feeding is itself sensitive to the presence or absence of food. To investigate the brain regions that are involved in FAA, Nakahara et al. (2004) examined c-Fos expression before and after feeding in rats subjected to restricted feeding (RF) for 2 h. The thalamic paraventricular nucleus (PVT) was the only region in which c-Fos expression was higher before feeding than after feeding. After FAA was established, lesions created in the PVT attenuated this rhythm but did not affect the LD-entrained rhythm. The anticipatory increase of blood corticosterone levels was not established in long-term PVT-lesioned rats. These results suggest that the PVT is involved in the expression of FAA under a SF regimen.
Recently, Mendoza et al. (2010) reported that FAA was markedly reduced in mice injected intracerebroventricularly with an immunotoxin that depletes Purkinje cells in the cerebellum (OX7-saporin). In addition, Grid2 (ho/ho) mice did not show any FAA or SF-induced changes in cerebellar clock gene expression. However, in hypothalamic arcuate and DMH, shifts in PER1 expression in response to SF were similar in the cerebellar mutant and wild-type mice. The study concluded that the cerebellar oscillator is required for FAA formation.
13.2.4 Role of Clock Genes in the FEO
FAA rhythm formation was attenuated by mutations in the Per2 Brdm1 gene but not the Per1 Brdm1 gene (Feillet et al. 2006). In contrast to the phenotype in Per2 Brdm1 mutant mice, no obvious alteration of behavioral FAA has been reported in mice carrying Per2 1dc , which is considered a null-mutant allele (Storch and Weitz 2009 ). This difference may be due to the redundant function of paralog genes and/or compensatory developmental mechanisms present in the mice bearing the loss-of-function mutations, which are absent in the mice bearing point mutations or a short deletion in the gene (Takahashi et al. 2008, for review). Per2 1dc mutation, but not Per2 Brdm1 , may compensate for Per1 and/or Per3, although the mechanism for this is still unknown. Fuller et al. (2008) also recently reported that cell-specific downregulation of Bmal1 gene expression in the DMH reduced FAA formation. Our data, showing that mice with a Cry1/Cry2 double mutation exhibit FAA with several unstable changes, support these findings (Iijima et al. 2005). However, Bmal1 knockout mice exhibit normal FAA rhythm formation (Mistlberger et al. 2008). Multiple food-entrainable circadian clocks have been discovered in the brain and periphery, raising strong expectations that one or more of them underlie FAA. Mutant mice lacking a known circadian clock function (Bmal1, Per1, Per2, Clock, and Per1/Per2 double mutants) in all tissues exhibit normal FAA in an LD cycle and in constant darkness (Storch and Weitz 2009). Interestingly, a T-cycle experiment, exposing mice to non-24-h cycles, showed that FAA was entrained by T = 20 h to T = 28 h in Bmal1 mutant mice but not in wild-type control mice (Takasu et al. 2012). Therefore, normal clock gene expression is necessary to entrain to SF stimulation with a circadian cycle range, suggesting that the FEO is dependent on the known circadian clock. These studies indicate that FAA is the output of an oscillator or the output of a known circadian clock oscillator. As mentioned above, only a few clock genes have been considered within the framework of FEO. Specifically, the involvement of important components of the current model for molecular clockwork, such as Dec1, Dec2, Rev-erbα/β, and Rorα/β, remains to be investigated. The latter two genes, members of the orphan nuclear receptor superfamily, encode negative and positive regulators of transcription, respectively. These transcription factors are intracellular sensors for circulating lipids; they participate in diverse pathways of lipid and carbohydrate metabolism in the liver, skeletal muscle, and adipose tissues (Duez and Staels 2008). Rev-erbs and Rors genes are, therefore, involved in the circadian control of cellular metabolism (Yang et al. 2006; Teboul et al. 2008). It would be interesting to investigate whether these genes are necessary for the formation of FAA.
13.2.5 Role of NMDA Receptors in the FEO
Glutamate receptors are divided into three types depending on the secondary signal transduction pathway used: AMPA, NMDA, and mGluR. NMDA receptor antagonists impair learning/memory in passive avoidance, water maze, and radial maze learning performance. In circadian rhythm experiments, the NMDA receptor antagonist MK-801 attenuated the light-induced phase shift of behavioral rhythm and light-induced Per1 gene expression in the SCN (Moriya et al. 2000). Several days are required to establish the FAA, suggesting that the process of FAA formation is involved in the learning/memory process. In order to examine the role of NMDA receptor activation in the acquisition of FAA, we administered MK-801 to rats every day after 4 h of restricted feeding. MK-801 strongly inhibited FAA formation (Ono et al. 1996). Injection of MK-801 immediately before re-feeding attenuated feeding-induced Per1 gene expression in the mouse DMH (Moriya et al. 2009). Taken together, these data suggest that feeding-induced NMDA receptor activation is involved in FAA formation and increased Per1 gene expression.
13.2.6 Role of the Arousal Process in the FEO
Orexin knockout or destruction of orexin-positive cells impairs the maintenance of arousal and food-seeking behavior in mice (Akiyama et al. 2004; Mieda et al. 2004). These data suggest that activation of orexin neurons may be involved in FAA rhythm formation because it was attenuated by the destruction of orexin-positive cells (Akiyama et al. 2004) (Fig. 13.3). When orexin knockout mice were placed on an SF schedule, core temperature and activity entrained to the feeding schedule. Therefore, orexin is not required for the entrainment of activity and temperature to an SF schedule, but is required for the robust expression of gross locomotor activity seen in anticipation of the scheduled feeding (Kaur et al. 2008).
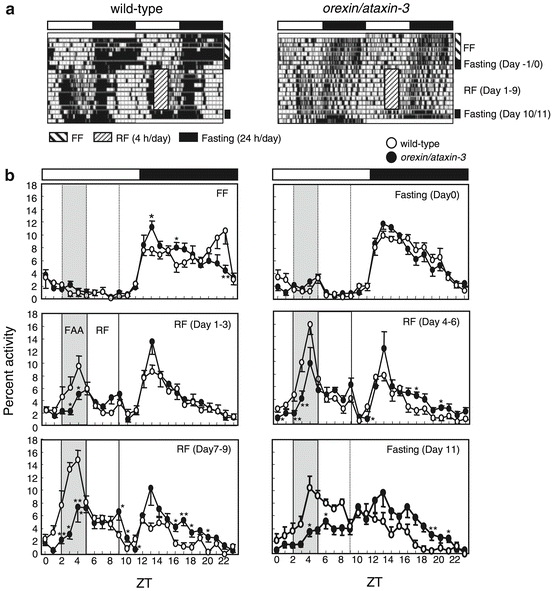
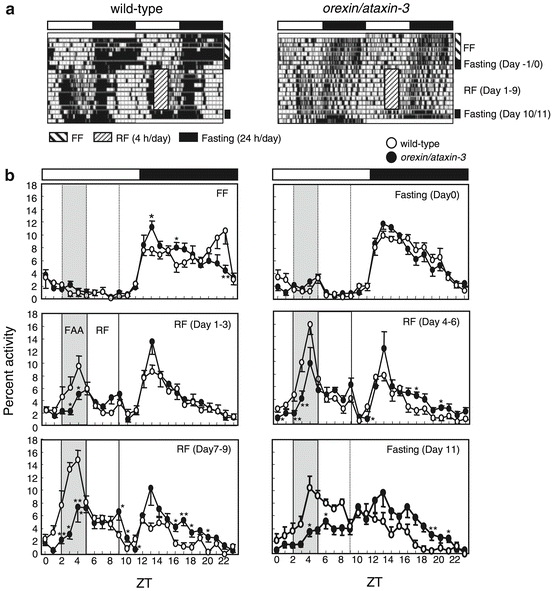
Fig. 13.3
Reduced FAA formation in orexin neuron-ablated mice (data from Akiyama et al. 2004). (a) Representative double-plotted actograms of the locomotor activity of restricted feeding (RF)-treated, wild-type (left), and orexin⁄ataxin-3 (right) mice (C57BL/6 background and 12 weeks old). The hatched box indicates RF treatment for 9 days (Zeitgeber time (ZT) 5–9 of days 1–9), during which food was available from ZT 5–9. Mice were fasted for 2 days before (days −1 and 0) and after (days 10 and 11) RF (fasting). (b) Averaged activity counts per hour as a fraction of the activity count in one whole day were plotted for wild-type (s, n = 5) and orexin⁄ataxin-3 (days, n = 5) mice. FF, averaged count under free-feeding (FF) conditions; fasting (day 0) and fasting (day 11), averaged count of fasted mice on day 0 and day 11, respectively; RF (days 1–3, days 4–9, and days 7–9), averaged activity count for each 3 consecutive days during RF treatment; *P < 0.05; **P < 0.01 vs. wild-type mice (Student’s t-test)
13.2.7 Role of Aging in the FEO
Aging affects learning/memory formation, and, in general, aging animals exhibit impaired learning/memory. Aging rats (≥1.5 years old) showed impaired FAA formation (Shibata et al. 1994). In other experiments, the effects of aging on the development and persistence of feeding-associated circadian rhythms were examined with respect to the pre-feeding plasma corticosterone peak levels under an RF schedule. Aging (12- to 20-month-old rats) impaired the development and persistence of feeding-associated circadian rhythm in rats (Walcott and Tate 1996 ). We examined the attenuation effect of bifemelane hydrochloride (a drug used in the treatment of senile dementia) on the impairment of FAA using aged and MK-801-treated rats (Shibata et al. 1995). FAA was impaired in 24-month-old rats and MK-801-treated rats. Daily injections of bifemelane or aniracetam (an experimental drug with potential cognition-enhancing effects) for six successive days significantly attenuated the impairment of FAA in a dose-dependent manner (Shibata et al. 1995; Tanaka et al. 2000). These results suggest that bifemelane and aniracetam enhance learning and memory performance, such as spatial and temporal perception.
13.2.8 Role of Nutrients in the FEO
Several circulating metabolic and hormonal factors driven by the SCN are also driven by food intake, including glucose, free fatty acids, glucocorticoid, thyroid hormones, and other factors (Escobar et al. 2009). Le Minh et al. (2001) demonstrated that adrenalectomized rats entrained faster to SF, suggesting that an induced corticosterone rhythm alone is not sufficient to drive FAA. At the behavioral level, glucose was the strongest entraining factor related to feeding schedules. In addition, a caloric-rich diet had a stronger influence on FAA induction than a fiber-rich or fat-rich diet (Stephan 1997). Because of the direct link to glucose, insulin secretion has also been examined for a potential role in FAA. However, because insulin did not induce or influence FAA, it has been disregarded as a main entraining signal for peripheral oscillators (Davidson and Stephan 1999 ; Kudo et al. 2004). It is worth mentioning here that mice fed a ketogenic diet show a phase-advanced rhythm of locomotor activity (Shirai et al. 2007; Oishi et al. 2009). Other food components, including sodium chloride, cholesterol, and proteins, do not have the capacity to phase-shift clock gene oscillation (Mohri et al. 2003; Iwanaga et al. 2005). Under ketogenic diet conditions, shortage of energy from carbon hydrate may happen in the SCN, and AMPK activation may affect CRY1 degradation (Lamia et al. 2009), resulting in free-running period changes. This may be one of the mechanisms by which ketogenic diet causes phase advance.
13.2.9 Role of Ghrelin in the FEO
Ghrelin is an orexigenic peptide predominantly secreted from the stomach. It acts on hypothalamic targets such as the ventromedial hypothalamus or arcuate nuclei to trigger food intake (Zigman et al. 2006). Plasma ghrelin is increased in calorie-restricted laboratory rats (Johansson et al. 2008) and gray mouse lemurs (Giroud et al. 2009). Stimulation of the ghrelinergic system can affect SCN activity in rodents (Yannielli et al. 2007). Interestingly, studies in mice with a targeted mutation in the ghrelin receptor gene show a reduction in FAA formation (Blum et al. 2009; LeSauter et al. 2009). Furthermore, ghrelin has phase-advancing effects in fasted but not fed mice (Yannielli et al. 2007). Therefore, ghrelin is considered a chronomodulating hormone possibly involved in the changes of activity timing during calorie restriction.
13.2.10 Role of Multiple Meal Times in the FEO
SF once per day has been a useful tool for investigating the FEO, but SF may not accurately represent the food availability patterns of nocturnal rodents in the wild. An alternative approach to assess the effects of multiple feeding times like in the wild is to schedule smaller meals throughout the day. Previously, meals were delivered 2–6 times per day to understand whether multiple FEO pacemakers are used to track each mealtime. Rats exhibit FAA when fed two meals per day separated by 5 h or more; both activity bouts exhibited properties of circadian timing rather than interval timing (Mistlberger et al. 2009; Stephan 1989a, b; Davidson et al. 2003). Some rats displayed partial FAA when they received two or three meals per day, but none anticipated all three meals simultaneously (Stephan 1989a). In addition, delivery of six meals per day did not lead to the same development of coordinated FAA (Mendoza et al. 2008). Interestingly, rats exhibited FAA twice with two meals per day (daytime and nighttime) and showed entrainment in the liver and gastrointestinal organs that was consistent with only the nighttime meal (Davidson et al. 2003). However, this study did not explain why only the nighttime meal entrained the peripheral clock or why the peripheral organ clock displayed a unimodal peak, but not a bimodal peak, rhythm when the rats were fed two meals per day. Because wild animals and humans have more complex feeding styles, it is necessary to examine the effect of multiple feeding times and volumes on SF-induced entrainment. In the future, we will examine the effect of two or three meals per day on FAA formation by using different food volumes at each meal as well as different starvation intervals between each mealtime.
13.3 Mechanism of the Food-Entrainable Peripheral Oscillator
13.3.1 Role of the Hypothalamus in the FEPO
SCN lesions do not affect FAA formation. However, it is unclear whether SCN lesions affect the SF-induced entrainment of the liver circadian clock. We discovered that SF causes a phase advance in the expression rhythm of the liver clock genes in mice with or without an SCN (Hara et al. 2001). Our recent experiments demonstrated that DMH lesions do not affect the SF-induced phase shift of Per2 gene expression in the cerebral cortex and arcuate nucleus (Moriya et al. 2009). Therefore, the DMH may not be involved in restricted feeding-induced entrainment of the brain clock. When we monitored Per2 gene expression rhythm using PER2::LUCIFERASE knock-in mice, restricted feeding-induced phase advance of Per2 gene expression in the liver was unaffected by MBH lesions (Tahara et al. 2010).
13.3.2 Effect of Food Volume, Starvation Period, and Food Frequency on the FEPO
Restricted feeding for 4 h during daytime caused phase advance of the mouse liver clock (Feillet et al. 2006); hence, we measured food intake for 4 h after a 24-h fasting regimen in PER2::LUCIFERASE mice (Hirao et al. 2009). Consumption of food caused phase advancement of the liver clock in a food volume-dependent manner. Under our experimental conditions, fasting itself caused a marked increase in phase advancement of the liver clock. The liver clock phase was advanced in a feeding day-dependent manner. Previous studies showed that the volume of food produced large phase shifts in the FAA rhythm in rats; these shifts occurred in a food volume-dependent manner, whereas substitution of the diet with non-nutritive bulk food failed to produce phase shifts (Stephan and Davidson 1998). When rats were entrained to 20 g of food per day, approximately 6 g was necessary to produce phase shifts (Stephan and Davidson 1998). In our present study, we found that phase advance of the liver clock was dependent on the volume of food (0.7, 1.4, or 2.0 g/day). Mice consume 4–6 g of food per day under free-feeding conditions, and 1.4 g of food per day is required to cause phase shifts. Therefore, we estimated that at least 30 % of the food intake under free-feeding conditions is required to cause phase shifts in the liver clock in mice and rats. After the mice were fed 1.4 g of food per day, a marked increase in the phase advance of the bioluminescence rhythm was observed on the second day but not the first day. A previous study also reported clear phase shifts induced by restricted feeding schedules on the second day of daytime feeding (Le Minh et al. 2001). Therefore, entrainable stimulation for at least 2 days may be necessary to produce a phase advance, and entrainable stimulation for 4 days is needed to produce a maximum phase advance of the liver clock.
When mice are fed two or three meals per day, food administered after longer intervals of fasting has more power to entrain peripheral clock phase than food administered after other food timings. In fact, mice fed at Zeitgeber time (ZT) 12, 18, and 1 were entrained by food at ZT 12, because food at ZT12 came 11 h after the last meal at ZT1, but the other meals came 6 or 7 h after the last meal (Kuroda et al. 2012). However, mice fed at ZT 12, 18, and 4 were entrained by food at ZT 4, because food at ZT 4 came 10 h after the last meal at ZT 18, but food at ZT 12 came 8 h after the last meal. In this paper, food timing mimicked human eating patterns: food at ZT 12 as breakfast, food at ZT 18 as lunch, and food at ZT 1 or ZT 4 as dinner, with ZT 4 as late dinner, in particular. This suggests that dinner has the power to the change clock phase if dinner occurs at late nighttime. Such a phase change may cause late dinner-induced obesity.
13.3.3 Effect of Nutrients on the FEPO
Glucose induces phase shifts in peripheral tissue clocks. In diabetic rats lacking insulin, the phase of cardiac circadian gene expression is advanced by approximately 3 h (Young et al. 2002), suggesting that high blood glucose levels can cause phase shifts in the peripheral tissue clocks. Clock resetting by downregulation of Per1 and Per2 has been observed in Rat-1 fibroblasts treated with glucose (Hirota et al. 2002). Glucose caused phase shifts in locomotor activity, such as FAA, when the rats were treated with SF (Stephan and Davidson 1998). The phase shift induced by glucose is probably not due to the higher energy content because the consumption of vegetable oil did not produce phase shift in rats on SF schedules (Stephan and Davidson 1998). Taken together, these data suggest that glucose may be at least one factor for peripheral tissue clock gene entrainment, as well as FAA, during SF conditions. We recently examined the effect of carbohydrates, including sugar and starch, on the entrainment of the circadian liver clock by using PRE2::LUCIFERASE knock-in mice (Hirao et al. 2009). Humans generally consume an appropriately balanced diet containing starch, protein, and oil, but not simple nutrients, including glucose. Therefore, to apply our study results to humans, appropriate dietary guidelines were developed. In order to elucidate the role of nutrition in inducing phase shifts, components of the AIN-93M diet were partially or completely substituted. In particular, we focused on cornstarch and sugar because glucose is a good candidate for inducing entrainment signals (Hirao et al. 2009).
It has been reported that fasting upregulates Per1 and downregulates Per2, Dec1, and Bmal1 in the mouse liver and that re-feeding prevents this fasting-induced change in gene expression (Kawamoto et al. 2006; Kobayashi et al. 2004). In our experiments, liver Per2 gene expression markedly increased when mice were re-fed after 24 h of starvation (Tahara et al. 2011). The increase of Per2 by re-feeding was dependent on the release of insulin from the pancreas. In addition to Per2, we also detected downregulation of Rev-erbα in the liver (Fig. 13.4). Therefore, the acute changes in clock gene expression that occur after re-feeding may cause a phase resetting of the peripheral clock.
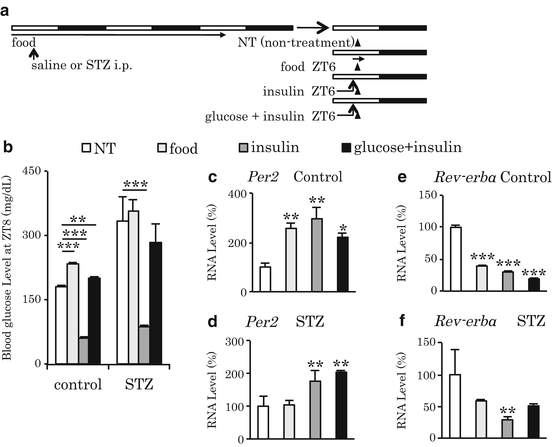
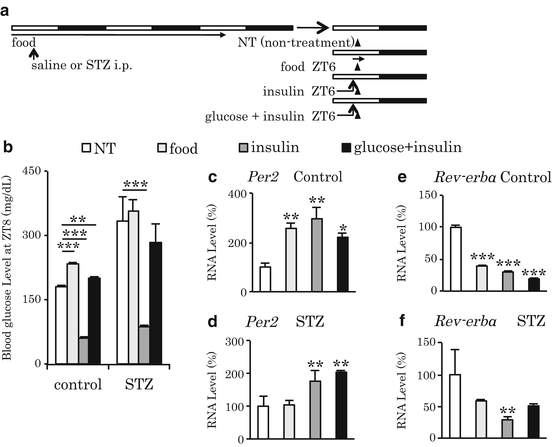
Fig. 13.4
Insulin-dependent, re-feeding-induced upregulation of Per2 and downregulation of Rev-erbα mRNA in the liver (data from Tahara et al. 2011). (a) Experimental schedule. Mice were treated with saline as control or streptozotocin (STZ; 200 mg/kg, i.p.) on day 0. On day 4, we prepared four groups. After 24-h fasting, mice were not treated (NT), fed (1 g), injected with insulin (5 U/kg, i.p.), or injected with insulin and glucose (1 g/ml/mouse, p.o.) at ZT 6 on day 4. Each arrowhead indicates the sampling point at ZT 8. Next, liver samples were analyzed for Per2 and Rev-erbα gene expression by real-time polymerase chain reaction. Three mice were assessed in the NT group, and four were assessed in the other groups. (b) Blood glucose levels were measured at the time of tissue sampling at ZT 8 on day 4. **P < 0.01; ***P < 0.001 vs. the NT group or the STZ condition, as determined by Fisher’s protected least significant difference (PLSD) test. (c, e) Per2 and Rev-erbα mRNA levels in the liver of control mice, respectively. (d, f) Per2 and Rev-erbα mRNA levels in the liver of STZ-treated mice, respectively. (c, f) The values of the NT group at ZT 8 were set to 100 %. *P < 0.05; **P < 0.01; ***P < 0.001 vs. NT group by Fisher’s PLSD test. Data are presented as the mean ± SEM
13.3.4 Effect of Nutrient Substitution on the FEPO
Each component of the control AIN-93M diet was completely substituted as a single nutrient (Hirao et al. 2009). An insignificant, weak phase advance of the liver clock was observed with 100 % cornstarch. Similarly, an insignificant, weak phase advance of the liver clock was observed in mice administered 0.3 ml of soybean oil on the first day and 0.43 ml of soybean oil on the second day, compared to the mice from the 2-day fasting group. Because neither a 100 % glucose diet nor a 100 % sucrose diet caused phase advances, other components in AIN-93M may cooperatively play a role in the action of sugar on the phase advance of the liver clock (Fig. 13.5). The combination of 86 % glucose with 14 % casein significantly (P < 0.05 vs. 100 % glucose) increased the phase advance of the liver bioluminescence rhythm. This study suggests that a balanced diet such as the control AIN-93M diet is important for the entrainment of the liver circadian clock. The present data demonstrated that simple diets such as 100 % sugar, 100 % protein, and 100 % oil are inadequate for inducing entrainment signals. Therefore, we suggest that a balanced diet such as the control AIN-93M diet not only maintains the health and metabolism of mice but also induces entrainment signals in the peripheral circadian clock. Although 100 % glucose, 100 % casein, 100 % starch, or 100 % soybean oil failed to cause a significant phase advance in the present experiment, 86 % glucose + 14 % casein caused a significant phase advance, compared to 100 % sucrose alone. Ingestion of meals rich in protein and fat leads to the secretion of gut hormones such as cholecystokinin, secretin, and peptide YY from the upper small intestine (Dockray 2009; Arora 2006; Green et al. 1989). At present, we do not know the role of such gut hormones on the phase shift of the liver clock; however, some papers have suggested a role of cholecystokinin in circadian systems (Lundberg et al. 2007; Shimazoe et al. 2008).
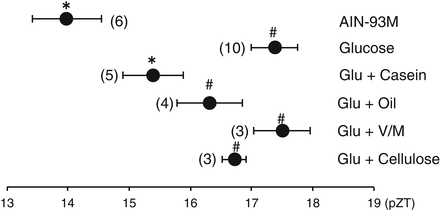
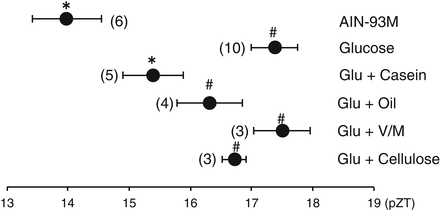
Fig. 13.5
The effects of complete substitution of a single nutrient component in the AIN-93M diet on the phase of the liver circadian clock in PER2::LUCIFERASE knock-in mice (data from Hirao et al. 2009). Mice were administered a diet tablet containing 0.6 g/10 g body weight (BW) of each nutrient in AIN-93M on the first day and 0.85 g/10 g BW of each nutrient on the second day after 24-h food deprivation. Some mice were administered 0.3 ml of soybean oil on the first day and 0.43 ml of soybean oil on the second day after 24-h food deprivation. The values indicate the mean ± SEM. The horizontal axis indicates the ZT at the peak of the bioluminescence rhythm. Fisher’s PLSD test. *P < 0.05 (vs. fasting); fasting, 2 days fast #P < 0.05, (vs. AIN-93M). The numbers in parentheses indicate the number of mice tested
In one behavioral study, glucose shifted the phase of FAA when the rats were subjected to a restricted feeding schedule (Stephan and Davidson 1998). We have found that in addition to the AIN-93M diet, 100 % glucose and 86 % glucose with 14 % casein caused FAA under a 2-day SF schedule, suggesting that 100 % glucose has the ability to produce FAA without affecting the liver clock. Dissociation of behavioral FAA rhythms and peripheral clock gene expression has been reported (Feillet et al. 2006; Hirao et al. 2009). Although the mechanism underlying the dissociation by glucose was not elucidated in the study, this is the first successful report of dissociation by diet. A combination of glucose and amino acids increased Per1 and Per2 gene expression and changed the phase of clock gene expression rhythms (Oike et al. 2011). However, we still do not know whether a specific amino acid or several amino acids in combination facilitate the glucose-induced phase shift.
13.3.5 Interaction of Entrainment Signals with Light, Food, and Corticosterone
Although light, food, and corticosterone can entrain peripheral clocks, there are some interactions among these entraining signals. SF-induced FEPO is observed under light–dark conditions and SCN-lesioned conditions, suggesting food-entraining signals are more powerful than light signals in the peripheral clock. Re-entrainment by a shift of the light–dark cycle was potentiated by adrenal organ depletion (Le Minh et al. 2001), suggesting that corticosterone released by the adrenal organ may negatively affect light–dark cycle entrainment. Chronic administration of prednisolone (a synthetic steroid) abolished the circadian rhythm of the peripheral clock. Recently, peripheral organ-specific entrainment was observed. Daily injection of corticosterone entrained the circadian clock of the kidney and lung, but not that of the liver. On the other hand, the liver clock was entrained by daily SF, even when daily corticosterone injections were given at antiphase. Thus, organ- and cellular-specific entrainments may exist.
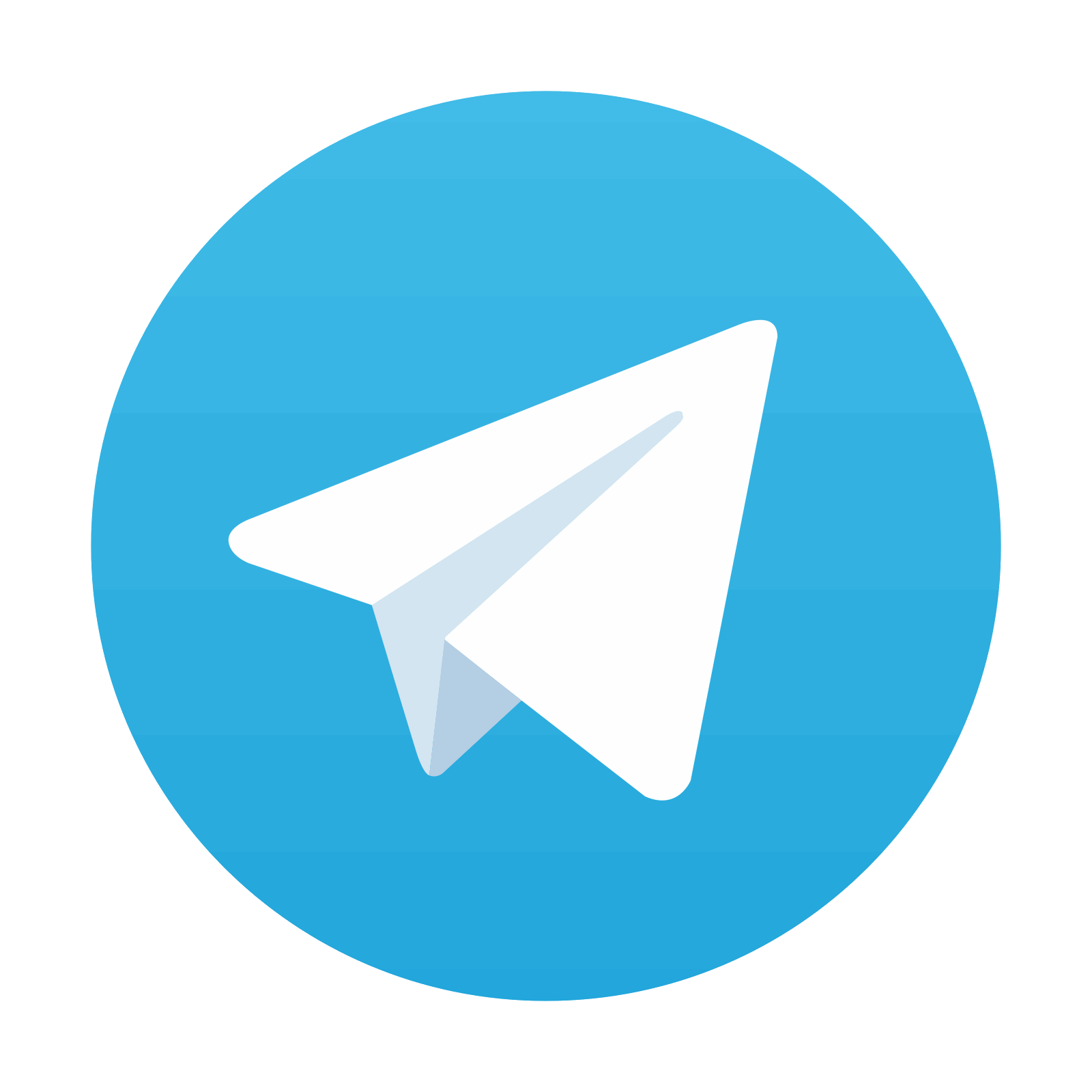
Stay updated, free articles. Join our Telegram channel

Full access? Get Clinical Tree
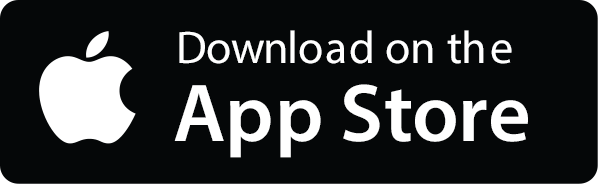
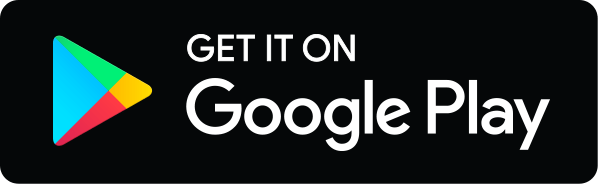