Fig. 12.1
Regulatory negative feedback system and graphs from its outcome versus time and as a phase portrait. (a) Diagram shows the main components of a regulatory negative feedback system: the effector, which generates the regulated parameter or output signal; the sensor, which detects the value of the regulated parameter and generates a signal that feeds back into the controller; the controller, which encompasses information regarding the optimal value or set point to which the parameter is being regulated; and a comparator, which determines the difference between the set point and the feedback signal. Such difference is translated into an error signal that modifies the output of the effector to compensate any deviation of the regulated parameter from its optimal value or range. The information flowing through the system is indicated in italic letters; the elements of the system are indicated with non-italic font. The set point is in bold italic letters to indicate that it could be either a constitutive element or information flowing through the system. (b) Schematic graph of the variation of a regulated parameter in time shows that any deviation from the set point (dotted horizontal line) is corrected within a short interval. (c) Schematic graph of the phase portrait from the data graph in b; in this graph the value of the parameter at time n (tn) is plotted against the value of the parameter at the next time n + 1 (tn + 1), as indicated by the vertical dotted lines in b; tn + 1 is then reset to tn and the process continues. In this type of graph the output of a negative feedback-regulated system is plotted within a small circular or elliptical area; the better the system is regulated the smaller is the area of the circle or ellipse
The heuristic value of the concept of homeostasis is reflected in the changes in ideas about the causes and treatment of disease, which have led to an increase in life expectancy from the last quarter of the nineteenth century to the first half of the twentieth century. So strong was the concept of homeostasis, and the misconception that during healthy conditions only minor deviations from the set points could take place, that the first reports on daily fluctuations in the value of physiological parameters, associated with biological rhythms, were received with indifference and skepticism by most members of the medical community. The concept of homeostasis remained unchanged and basically unchallenged until 1977, when Nicolaïdis provided evidence that under certain circumstances the values of the set point of homeostatic systems could be altered.
12.2 Rheostasis (Allostasis)
Even although Cannon never suggested that the set points of the homeostatic system were fixed throughout life, it was a common and unsubstantiated assumption; nevertheless, evidence that indicated that the set point could change from one value to another opened new perspectives to the understanding of physiology. Hammel (1968) proposed that the set point was adjustable to explain acute thermoregulatory corrections. Later on, Nicolaïdis (1977) proposed the term homeorheusis to refer to long-term changes in the set point of body weight occurring through life. Mrosovsky (1990) renamed this phenomenon rheostasis, and further developed this concept by providing an extensive review of the different conditions in which changes of the set point occur (Fig. 12.2).
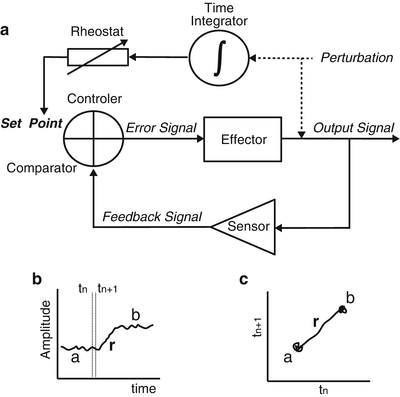
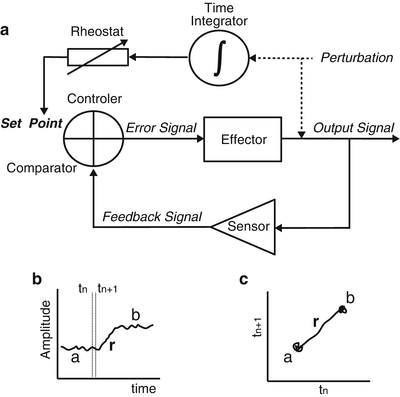
Fig. 12.2
Rheostatic regulatory system and graphs from its outcome versus time and as a phase portrait. (a) Diagram indicates a negative feedback system regulated by rheostatic processes. The set point of the feedback system varies according to sustained changes in environmental conditions or to the needs of the organism. Both environmental and internal changes are here indicated as perturbations. In this model we postulate that only two essential elements form the rheostatic regulatory system: a time integrator to evaluate whether there is a sustained perturbation which requires a change in set point values, and a rheostat element to generate a signal to modify the set point of the system. The rest of the elements of the system are as in Fig. 12.1. (b) Schematic graph of the rheostatic modification (r) of the set point of a regulated variable from a to b; at all other times any small deviation of the regulated parameter from the set point is corrected within a short interval. c Schematic graph of the phase portrait from the data graph in b. In this graph we can appreciate the trajectory of the regulated parameter from point a to b and the trajectory of the regulated change r
During their lifespan organisms frequently face changes in their physiological or environmental conditions; in some of these events, maintaining a physiological parameter within the narrow range dictated by an immovable set point would challenge the survival of the individual by preventing adequate physiological responses. To prevent the failure of homeostatic regulation, in such situations the organism would require processes or mechanisms that allow switching set point values under regulated conditions. Rheostasis refers to such processes and mechanisms that allow adjustment of homeostatic processes when facing changes in physiological or environmental conditions. Two types of rheostasis are recognized: when the change in set point is triggered by influences external to the organism it is considered as reactive rheostasis; in contrast, when these changes are triggered by genetic programs, such as those occurring at specific times during development, it is considered as programmed rheostasis. It is worth noting that Mrosovsky considered circadian rhythmicity as programed rheostasis; however, for reasons that are presented next (see Sect. 12.3), we consider circadian and other biological rhythms as regulatory processes different from rheostasis.
Sterling and Eyer, while studying the physiological changes induced by stressful life situations, developed a concept similar to that of rheostasis but independent from Nicolaïdis and Mrosovsky. They named the stability attained through change allostasis, that is, “to maintain stability an organism must vary all the parameters of its internal mileu and match them appropriately to environmental demands” (Sterling and Eyer 1988; quoted in Boulos and Rosenwasser 2005, p. 228). This definition is essentially similar to stating that the set point in homeostatic systems should be modified to adjust the physiological and behavioral responses of an individual to changes in its environment or physiological needs. Thus, although for all practical purposes we may consider the two terms, rheostasis and allostasis, as equivalent concepts, nevertheless we prefer to use the term rheostasis.
12.3 Chronostasis
Periodic variations in human functions that repeat every 24 h have been known for almost 60 years (Aschoff 1955, cited in Aschoff 1965), but its impact on human physiology and medicine was not noticeable until about 30 years later (Moore-Ede et al. 1982). It was not until the clock genes were characterized in rodents and their human homologues identified that widespread attention was attracted to the role of circadian rhythmicity as a probable cause of disease (Lowrey and Takahashi 2004; Turek et al. 2005). In spite of increased awareness of the relevance of circadian rhythmicity for health and disease, integration of circadian timing within a coherent conceptual framework on physiological regulation is still lacking. Such a conceptual framework will allow us to insert circadian rhythms in the perspective of integrative physiology, as well as to integrate recent findings related to clock genes and their interaction with genes related to regulation of the cell cycle or metabolism originally studied in the context of cell biology and genetic regulation.
As previously mentioned, Mrosovsky (1990) considered circadian and other biological rhythms as programed rheostasis, and other authors have accepted this perspective without much further elaboration (Boulos and Rosenwasser 2005), whereas some others considered circadian rhythms as part of the homeostatic process (Moore-Ede et al. 1982; Perreau-Lenz et al. 2004; Woods and Ramsay 2007). The first conceptual model that attempted to join homeostatic regulation with circadian rhythmicity was developed by Alexander Borbély (1982) to account for the nonlinear sleep rebound that occurred after increasing duration of sleep deprivation. This model proposed that sleep regulation involves an interaction between circadian and homeostatic processes. The model, later formalized by Daan et al. (1984), also provided computer simulations that could reproduce a number of phenomena related to recovery from sleep deprivation and internal desynchronization induced by temporal isolation in humans. Internal desynchronization refers to the loss of the time relationship among physiological parameters such as temperature and sleep onset, which occurs when the subject is maintained isolated in an environment free of time cues and thus loses track of the time. More recently, Kalsbeek et al. (2006) have provided abundant evidence regarding glucose regulation involving both circadian and homeostatic processes. Nevertheless, Kalsbeek considers circadian rhythms as guarding homeostasis, thus missing the opportunity to recognize the wider spectrum of parameters (amplitude, period, and phase of rhythmicity; see following) regulated by circadian modulation of the homeostatic systems and the cooperative aspects among time regulation (chronostasis) and homeostasis.
The concept of an adjustable set point in a feedback system as proposed by Mrosovsky for rheostasis is a necessary step to incorporate time regulation into physiological systems. Nevertheless, the dependence of circadian rhythmicity on biological clocks or oscillators provides particular characteristics about this type of physiological regulation that justify its consideration as a process independent from rheostasis. Furthermore, in the past 15 years our knowledge of the cellular and molecular mechanisms of circadian clocks has advanced to allow us to set apart the physiological time regulation or chronostasis from the rest of the processes included in the concept of rheostasis and homeostasis.
The term chronostasis was coined to refer the cyclic timing (circadian or otherwise) of physiological systems (Fig. 12.3). We followed the reasoning of Mrosovsky for the term rheostasis, but here the prefix homeo or rheo was substituted by chrono to indicate that the steady state guarded by homeostatic and rheostatic processes is time modulated. Chronostasis is used to refer to the cyclic time regulation of physiological systems, which includes the period, amplitude, and phase of the oscillations in any physiological variable, as well as the phase relationship among different physiological systems and also with environmental cycles. The period of a rhythmic phenomenon refers to the elapsed time for a complete cycle to occur; in natural (entrained) conditions, the period of the circadian rhythmicity is 24 h. Amplitude refers to the change in the value of the variable from its highest value (peak) to its lowest (trough). In the context of the cosinor analysis (which is an statistical procedure used to fit cyclic phenomena to a cosine function), amplitude refers to the change in intensity of the variable from the mean value of the variable throughout the cycle (mesor) to the highest value of the best fitting cycle (acrophase). Finally, the phase of the rhythm refers to the time of the cycle at which any particular value of the cyclic variable occurs; for example, the time of waking or sleep initiation corresponds to a particular phase of the sleep–wake cycle. Also, the time at which the peak or acrophase occurs is also a clear-cut phase reference for cyclic or rhythmic phenomena. Finally, because each rhythmic variable has specific phase descriptors, the time relationship between two variables is best referred to as a phase relationship. Thus, it is clear that time regulation of physiological variables broadens the scope of parameters under study and analysis, beyond the changes in their mean values and their reset to new values, as seen from homeostatic and rheostatic perspectives, respectively. These considerations provide further support to the view of chronostasis as an independent regulatory process in physiology.
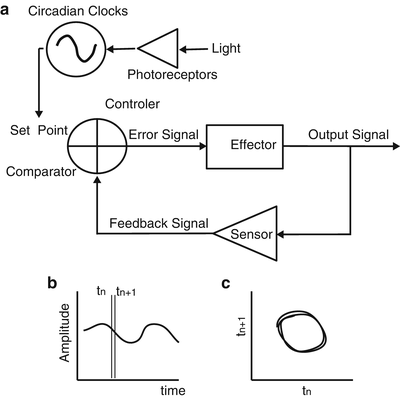
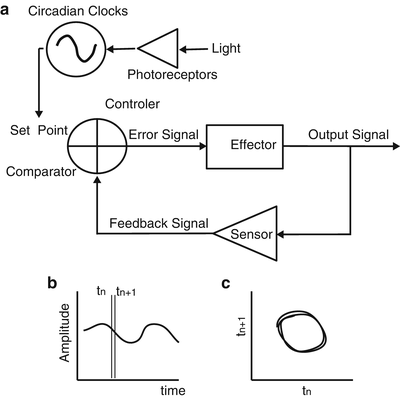
Fig. 12.3
Chronostatic regulatory system and graphs from its outcome versus time and as a phase portrait. (a) Diagram indicates a negative feedback system regulated by chronostatic processes. The set point of the feedback system varies according to the time signal generated by the circadian clock. In this scheme the clock is entrained to the light–dark cycle by the effects of light on specialized photoreceptors, which in mammals generate a glutamatergic signal that is received by the suprachiasmatic nuclei (SCN) to adjust the phase and period of the circadian molecular clocks. (b) Schematic graph of the regulated signal shows circadian rhythm in the regulated parameter. (c) Schematic phase portrait from the data graph in b shows the limit cycle of the regulated parameter; the dispersion from a perfect circular or elliptical trajectory reflects mainly the perturbations occurring throughout the cycle. At any given time the phase of the limit cycle is regulated within a narrow range by the negative feedback regulatory system
12.3.1 A Review of the Set Point Concept
Because the adjustable set point from a regulatory feedback loop is a key concept in rheostasis and chronostasis, a brief review of this concept is required before any further elaboration on chronostasis. According to Cabanac (2006) a set point is “an information input that may be determined by an external signal to which the regulated variable is compared, or it may be determined by the structural characteristics of the system itself.” Thus, the set point refers to the information used by a regulatory system to compare with the actual value of the regulated variable, to generate a correction signal to actively counteract any deviation presented in the regulated variable from a desired value, which is somehow encoded by (or in) the set point.
The notion of the set point as an “external signal” most not be misunderstood as external to the organism, but rather as external to the regulated system (or subsystem) although originating from the organism itself. On the other hand, nowadays the notion of the set point as an “structural characteristic” of the system extends beyond the architecture of the regulatory feedback loops, either from neural or endocrine networks (Russek and Cabanac 1983), but also includes the concentration of hormones or neuroactive substances, the density of channels and chemical receptors, or even the constitutive level of expression of some genes (Boulant 2006; Roth et al. 2006; Jéquier and Tappy 1999; Jéquiere 2002; Speakman et al. 2011; Viola et al. 2007; Wright et al. 2008; Goel et al. 2009).
The concept of set point has been questioned by some physiologists, who suggest it should be discarded because it is confusing and misleading to the point of oversimplification, whereas regulatory systems are quite complex and involve several subsystems and regulatory loops. A deeper review of such criticisms is beyond the scope of this chapter, but for those interested in this controversy we suggest referring to Harris (1990), Werner (2010), Romanovsky (2004, 2006), Cabanac (2006), Jéquier and Tappy (1999), and Speakman et al. (2011). Although we agree with Werner that in the end we must examine the actual experimental data to draw any conclusion on how a physiological system is regulated, the idea of set point allows us to examine the hypothesis of a possible integrative mechanism underlying time regulation of physiological processes, at different levels of biological complexity. Thus, we shall not discard the concept but embrace it in its complexity.
Here the concept of chronostasis is proposed as the set of mechanisms involved in the rhythmic timing of physiological regulation. This model incorporates circadian clock mechanisms, to the feedback regulatory loops used in homeostatic and rheostatic regulatory systems, which feedforward a time signal to modulate the set points of the system (Fig. 12.3). Thus, the feedback-regulated variables are compared to different optimal values that depend on the time of the day (Aguilar-Roblero and Díaz-Muñoz 2010).
12.3.2 Time Regulation of the Set Point
In mammals, the major circadian clock has been located in the suprachiasmatic nuclei (SCN), in the anterior hypothalamus, and interconnected to autonomic and behavioral regulatory systems located mainly in the hypothalamus (Klein et al. 1991). At the molecular level the circadian clock is formed by a set of genes, known as clock genes, functionally arranged in a transcription–translation feedback loop with a delay that oscillates with a period close to 24 h. The clock genes have been found in brain regions other than the SCN (Abe et al. 2002; Yamazaki et al. 2000) and also outside the brain in most organs of the body (Yamazaki et al. 2000, 2002), which indicates the presence of multiple circadian oscillators collectively known as peripheral circadian oscillators. It has been suggested that the circadian system is a hierarchical multioscillatory system, coordinated by neural and endocrine systems driven by the SCN. This hierarchical multioscillatory circadian system would enable different physiological systems to resonate at a circadian frequency (Aguilar-Roblero and Díaz-Muñoz 2010). Thus, the clock mechanisms involved in chronostatic time modulation can be recognized at a systemic level in the SCN and its neuronal network that modulates regulatory neural and neuroendocrine networks. At a cellular level chronostatic time modulation occurs throughout the body by clock genes from peripheral oscillators, coordinated in time by neural and endocrine signaling, interacting with regulatory systems of metabolic intracellular signaling, and gene expression.
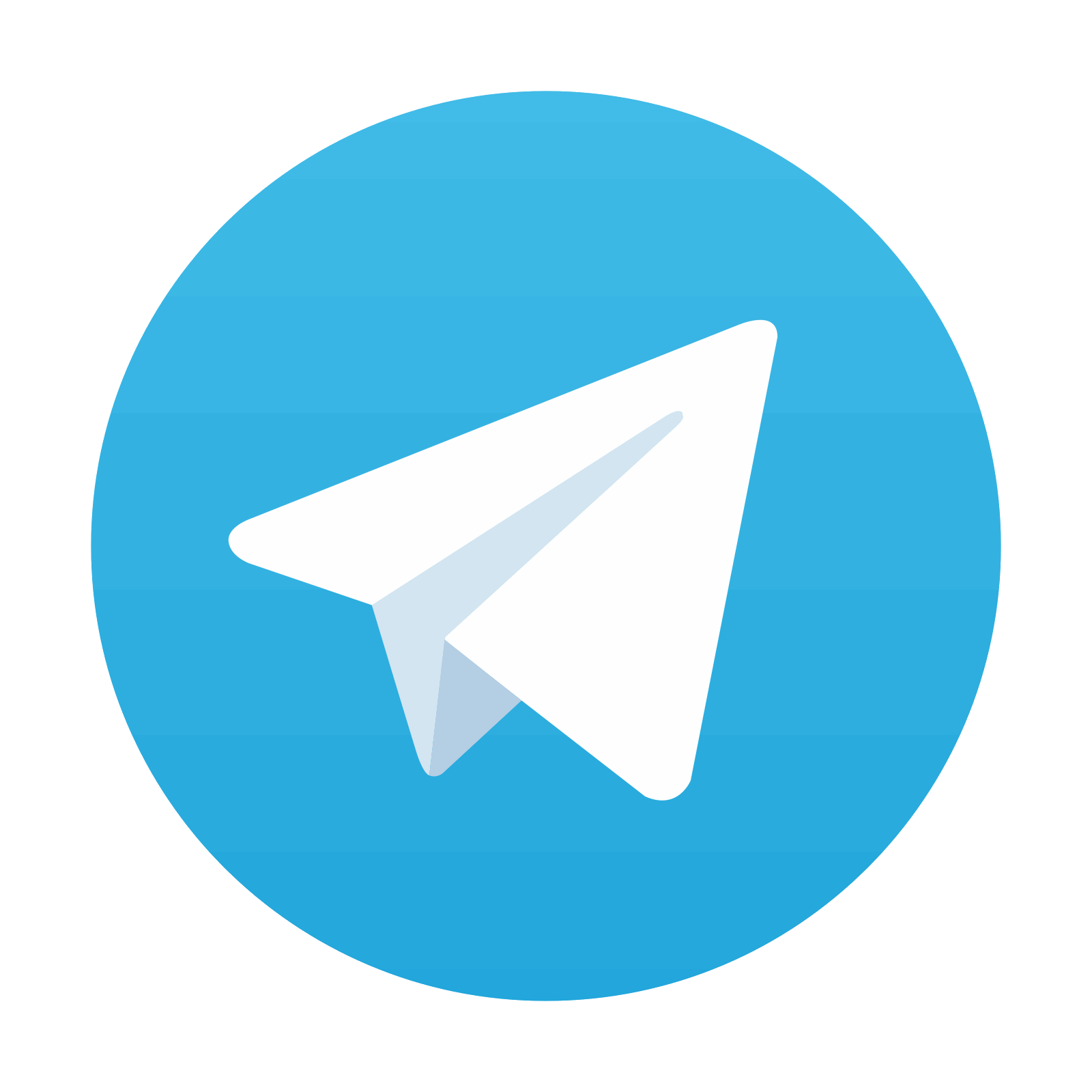
Stay updated, free articles. Join our Telegram channel

Full access? Get Clinical Tree
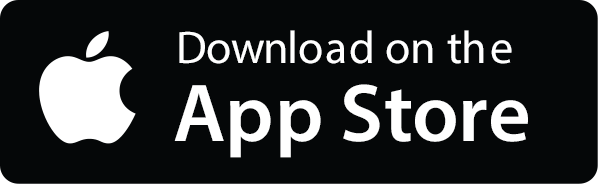
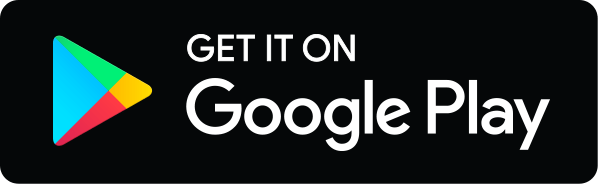