Chapter 1.4
Characterization of canine filaggrin: gene structure and protein expression in dog skin
Background – Filaggrin (FLG) is a key protein for skin barrier formation and hydration of the stratum corneum. In humans, a strong association between FLG gene mutations and atopic dermatitis has been reported. Although similar pathogenesis and clinical manifestation have been argued in canine atopic dermatitis, our understanding of canine FLG is limited.
Hypothesis/Objectives – The aim of this study was to determine the structure of the canine FLG gene and to raise anti-dog FLG antibodies, which will be useful to detect FLG protein in dog skin.
Methods – The structure of the canine FLG gene was determined by analysing the publicly available canine genome DNA sequence. Polyclonal anti-dog FLG antibodies were raised based on the canine FLG sequence analysis and used for defining the FLG expression pattern in dog skin by western blotting and immunohistochemistry.
Results – Genomic DNA sequence analysis revealed that canine FLG contained four units of repeated sequences corresponding to FLG monomer protein. Western blots probed with anti-dog FLG monomer detected two bands at 59 and 54 kDa, which were estimated sizes. The results of immunohistochemistry showed that canine FLG was expressed in the stratum granulosum of the epidermis as a granular staining pattern in the cytoplasmic region.
Conclusions and clinical importance – This study revealed the unique gene structure of canine FLG that results in production of FLG monomers larger than those of humans or mice. The anti-dog FLG antibodies raised in this study identified FLG in dog skin. These antibodies will enable us to screen FLG-deficient dogs with canine atopic dermatitis or ichthyosis.
Introduction
Filaggrin (FLG) is known to be a key protein for skin barrier formation. Filaggrin is translated as a large precursor protein, proFLG, which consists of tandem FLG monomer repeats. Each FLG monomer repeat is then processed to generate the FLG monomer in keratohyalin granules in the stratum granulosum of the epidermis. Filaggrin monomer proteins function in keratin filament aggregation in the stratum corneum (SC)1 and are degraded to natural moisturizing factors during SC differentiation, which play an important role in skin hydration.2
Recently, it was reported that FLG gene mutations cause ichthyosis vulgaris in humans, which is characterized as a ‘dry and scaly’ phenotypic keratinization disorder.3 A strong association between FLG gene mutations and atopic dermatitis (AD) has been reported in Irish and other human populations, indicating that skin barrier dysfunction caused by FLG mutations represents a strong predisposing factor for AD.4
Similarity in the pathogenesis and clinical manifestation between human and canine AD (cAD) has been reported.5,6 Furthermore, genetic inheritance of AD was also reported in both species.7 It is important to validate whether skin barrier impairment caused by a FLG gene mutation is associated with cAD, as shown in human AD.
The gene structure and protein architecture of FLG has been reported in humans and mice.8,9 These studies showed that N-terminal and C-terminal regions of proFLG were conserved but the number and length of the FLG monomers differed between humans and mice. 8,9 It has been suggested that amino acid sequences of FLG monomers are highly variable in mammals. The aim of this study was to determine the FLG repeat structure of the canine FLG gene and to evaluate whether the deduced proFLG sequence differs from the human and mouse FLG sequence. Moreover, we raised anti-dog FLG antibodies against a synthetic peptide design based on the canine FLG monomer sequence predicted in this study. These novel anti-dog FLG antibodies were used to examine the expression pattern of FLG proteins in dog skin.
Materials and methods
Canine FLG genomic DNA sequence
The DNA sequence of the canine FLG gene was obtained from the database (http://asia.ensembl.org/index.html) that is based on the whole genome shotgun assembly CanFam2.0.10 Dot-matrix analysis by the dotter program11 was used to find repeated FLG sequences in the canine FLG gene sequence. The multiple sequence alignment program Clustalx12 was used for FLG comparison analysis. Sequence data of human FLG gene (NM_002016) and mouse Flg gene13 were used for comparison with the canine FLG gene sequence.
Animals
Three healthy laboratory beagle dogs were used for skin biopsy, two for histopathology and one for protein extract, and a healthy laboratory beagle dog was used for tape stripping (TS) in this study. A neonatal mouse (C57BL/6J strain) was used for protein extraction from skin. All animal experiments performed were approved by Keio University Ethics Committee for Animal Experiments and the animal research committee at Tokyo University of Agriculture and Technology.
Antibodies
Rabbit anti-dog FLG sera were prepared by immunizing New Zealand white rabbits with a synthetic peptide with amino acid sequence SRHSRTGHGSGNSKHR, which is located in the FLG monomer and corresponds to amino acid residues 998–1013, 1547–1562, 2096–2111 and 2603–2618 in canine proFLG. For western blotting, the IgG fraction was affinity purified using HiTrap™ Protein G HP (GE Healthcare, Uppsala, Sweden). For immunohistochemistry (IHC), the anti-dog FLG antibodies were affinity purified using antigen peptide and HiTrap™ NHS-activated HP (GE Healthcare).
Skin biopsy for histopathology and protein extraction
Six-millimetre-diameter punch biopsy specimens were obtained from the footpad, dorsal neck and axillae of healthy beagle dogs for histopathological examination and protein extraction. Samples for histopathology were fixed in 10% neutral buffered formalin and embedded in paraffin.
The protein samples for western blotting were extracted from the skin of a healthy beagle dog and a neonatal mouse (C57BL/6J strain). Skin extracts from the dog and the mouse were chopped and sonicated in sodium dodecyl sulfate (SDS) sample buffer [2% SDS, 0.125 mol/L Tris-HCl (pH 6.8), 10% glycerol, 0.005% bromophenol blue and 5% 2-mercaptoethanol], boiled at 95°C for 5–10 min, and then centrifuged at 20,400g for 10 min to collect the supernatant.
Western blotting
The extracted proteins were separated on 5–12% SDS–polyacrylamide gels and then transferred to Immobilon-P (Millipore, Billerica, MA, USA). Blots were probed with polyclonal rabbit anti-mouse FLG antibodies (Covance, Berkeley, CA, USA) diluted 1:6000 with phosphate-buffered saline (PBS; 137 mmol/L NaCl, 8.1 mmol/L Na2HPO4, 2.7 mmol/L KCl and 1.47 mmol/L KH2PO4) or polyclonal rabbit anti-dog FLG antibodies diluted 1:1000 with PBS and incubated overnight at 4°C. Immunoreactive proteins were detected in a second reaction using horseradish peroxidase-conjugated anti-rabbit immunoglobulin antibodies (Dako, Glostrup, Denmark) diluted 1:10,000 with PBS, incubated for 1 h at 25°C and visualized with ECL Plus western blotting detection reagents (GE Healthcare, Little Chalfont, UK).
Immunohistochemistry
Formalin-fixed and paraffin-embedded specimens were deparaffinized and then the antigen was retrieved by microwaving for 20 min in HistoVT One (Nacalai tesque, Kyoto, Japan). The slides were blocked with 10% goat serum and incubated with polyclonal anti-dog FLG rabbit antibodies diluted 1:200 with PBS overnight at 4°C. Incubation with secondary antibodies using Histofine SAB-PO (MULTI) (Nichirei Biosciences, Tokyo, Japan) was performed for 1 h at 25°C. The Histofine DAB (3,3′-Diaminobenzoide) substrate (Nichirei Biosciences) was used for the visualization of the immunolabelling, and slides were counterstained with haematoxylin.
Tape stripping and sample extraction
Skin biopsy samples were collected before and after 20 consecutive TS using PPS 625-25 (Nichiban, Tokyo, Japan) to collect the SC from a healthy beagle dog. The protein was extracted from each of 1–5, 6–10, 11–15 and 16–20 consecutive stripped tapes. Tapes were washed and boiled with SDS sample buffer, and then centrifuged as described above. Haematoxylin and eosin staining of skin biopsy specimens from samples collected before and after TS were performed to confirm the remaining amount of SC.
The extracted proteins from TS were separated on 10% SDS-polyacrylamide gels and transferred as described above. The blots were probed with polyclonal rabbit anti-dog FLG antibodies diluted 1:1000 with PBS or monoclonal mouse anti-pancytokeratin (clone AE1/AE3) antibodies (Thermo Fisher Scientific, Rockford, IL, USA) diluted 1:6000 with PBS. Immunoreactive proteins were detected using secondary antibodies and visualized as described above.
Results
Canine FLG sequence analysis
The canine FLG genomic DNA sequence in whole genome shotgun assembly (CamFam2.0)10 indicated that the epidermal differential complex (EDC) containing FLG was located on chromosome 17 in the canine genome. To distinguish the FLG gene from a FLG-like gene in EDC, we compared the order and direction of canine EDC genes with human and mouse genes. These analyses indicated that the order and direction of EDC genes are mostly conserved among humans, mice and dogs;14,15 therefore, we considered the canine FLG gene found in whole genome shotgun (CanFam2.0) to be a canine orthologue of the FLG gene (Figure 1a) and used this sequence for further analysis.
Figure 1. Features of canine filaggrin (FLG) gene and protein. (a) Deduced structure of canine FLG gene. The FLG gene consists of three exons in humans and mice. Unidentified exon 1 of canine FLG was assumed to be a noncoding exon, similar to the human and mouse genes. (b) The dot-matrix analysis between the canine proFLG coding sequence and the FLG repeat 1 sequence. Canine proFLG has four FLG repeats flanked by two truncated FLG repeats. (c) Structure of proFLG protein. Profilaggrin consists of an N-terminal region, four units of FLG monomers flanked by two truncated FLGs, and a C-terminal region. Abbreviation: ORF, open reading frame.

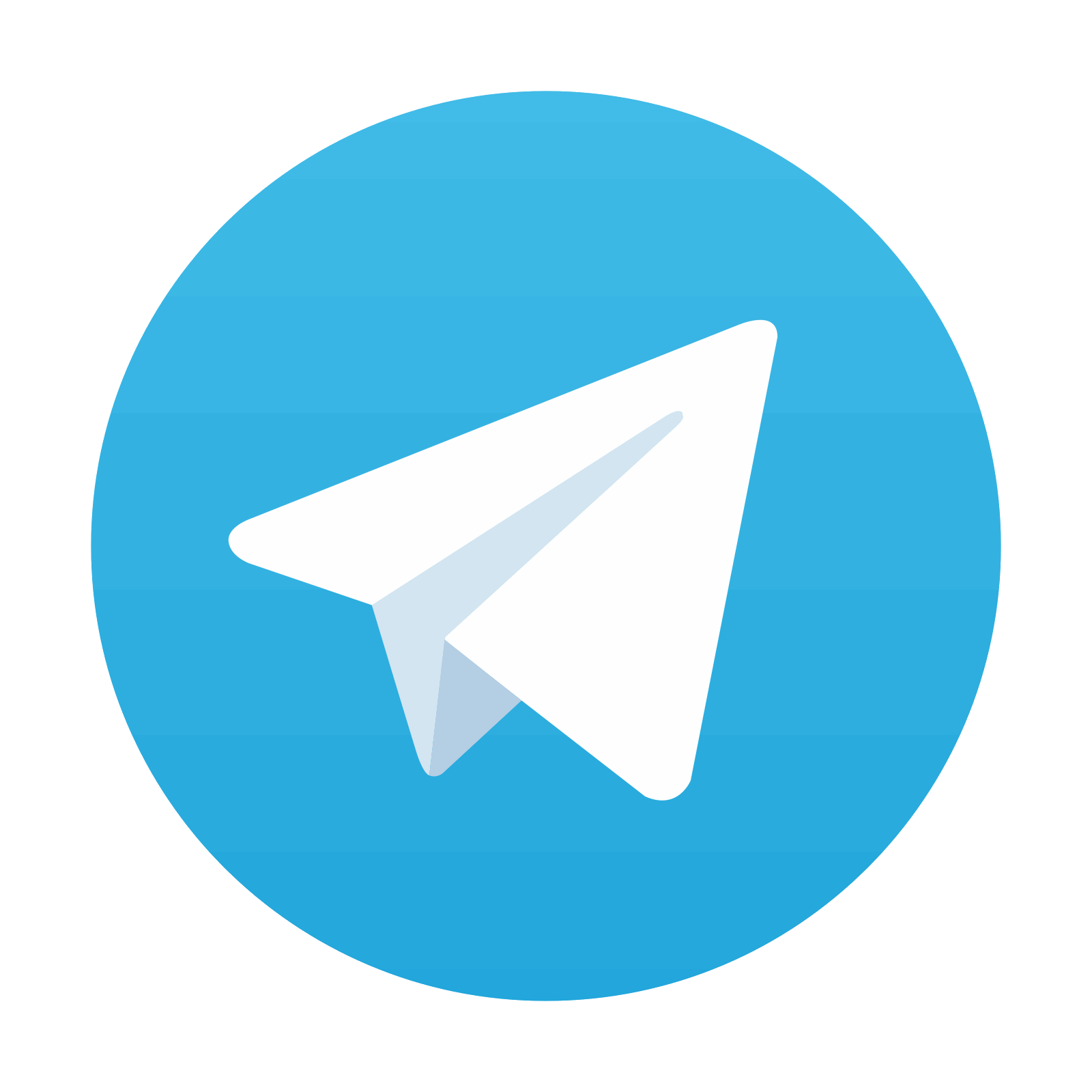
Stay updated, free articles. Join our Telegram channel

Full access? Get Clinical Tree
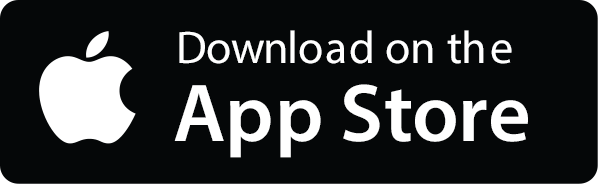
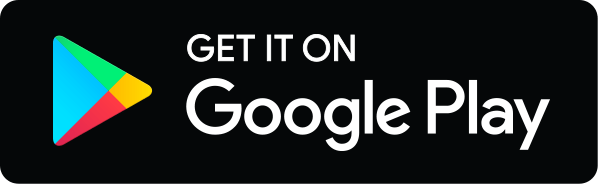