BLOOD VESSELS
Structure–Function Relationships of Blood Vessels
The blood vessels of the macrovasculature are visible with the naked eye and include elastic and muscular arteries and accompanying veins. Arteries of the macrovasculature carry the blood from the heart to the microvasculature, which includes arterioles, metarterioles, capillaries, venules, and arteriovenous anastomoses. Functioning as return vessels of the macrovasculature, veins then carry the blood from the microvasculature back to the heart.
Blood vessels are commonly defined by their position in the vascular circuit. They are further characterized histologically by their individual structure, which reflects the particular forces withstood during blood flow and the mechanisms of control over vascular function. Arteries control flow to the microvasculature where blood flows slowly and can stop intermittently because blood pressure is only slightly above or below the counteracting pressure of surrounding tissues. In veins, the velocity of the blood flow returns to at least half that of the corresponding arteries, but the blood pressure is reduced.
Contractions of the heart ventricles are the greatest impelling force to blood circulation. The thick conducting elastic arteries, such as the aorta, receive the first surge of blood from each contraction, during which both velocity of flow and pressure reach their peaks. The high pressure of the cardiac blood pumped out during contraction of the cardiac ventricles (systole) is largely absorbed by the stretch of the highly elastic arterial walls. At the time of ventricular dilation (diastole), arterial wall tension lowers while maintaining blood pressure, and the volume of blood is distributed into more numerous muscular arteries. These vessels lead to specific organs or body parts and eventually into the smallest branches of the arterial tree, the arterioles. The velocity of flow is gradually reduced as additional distributive branches greatly expand the total blood vessel volume, but pressure in the muscular arteries remains high. Peripheral outflow is regulated by the sympathetic division of the autonomic nervous system, which determines contraction or relaxation of the smooth muscle cells in the walls of these arteries.
Frequently, the elastic, muscular, and arteriolar arteries are referred to as large, medium, or small arteries, respectively. On a comparative anatomic basis, the latter nomenclature is confusing because an elastic artery of a cat may be of smaller caliber than a muscular artery of a large ruminant. But within one species, the assumption of a relationship between structure and relative size is valid.
From the arterial tree, the vessels open into voluminous networks of small, uniformly thin-walled tubules called capillaries. In the liver, bone marrow, and certain endocrine glands, the capillaries are called sinusoids. The total blood volume in capillaries and sinusoids is much greater than in arterioles, such that the velocity of blood flow decreases from meters per second in the arterial tree to less than 1 mm per second in capillaries. The shearing forces generated by viscous blood flowing within the narrow arterioles decrease the pressure of the blood until it exceeds the pressure of the surrounding tissue fluid by 10 mm Hg or less. Thus, capillaries only oppose small-scale forces and therefore can have thin walls structured from single cells. Blood-tissue exchanges take place within the capillary network, as well as across the wall of postcapillary venules, which have a comparable structure.
Blood from the capillaries and sinusoids returns to the heart via the veins. Veins of increasing size, usually classified simply as small, medium, or large veins, form inverse vascular trees that are analogous to, and in most instances parallel with, the arterial trees. Consequently, arteries and their accompanying veins are seen adjacent to each other in most tissue sections. Usually, a nerve and sometimes a lymphatic vessel are seen along with the paired blood vessels. There are, however, specific exceptions, such as in the lung where arteries and veins group independently.
Because veins receive blood from low pressure capillaries, they oppose little residual pressure from the pumping action of the heart. They do, however, withstand pressures caused by gravity and surrounding tissues such as muscle. Flow of blood through the veins is caused by the minimal pressure of blood flowing from the capillaries into the veins and by pressure differences with surrounding tissues. This flow is augmented by a series of valves in the long veins of the extremities that facilitate movement of blood toward the heart.
The small pressure gradients within the veins provide relatively low velocities of flow as compared to flow velocity in arteries. However, the venous channels are large in comparison to those of companion arteries; consequently, the rate of flow through the two different vessel types is equal.
Because veins are larger than arteries, they hold nearly half of the total blood volume in the body, and the contractile state of the walls of the larger veins is an important determinant of total vascular volume.
General Structural Organization of Blood Vessels
The walls of all blood vessels larger than capillaries are composed of three concentric layers or tunics that include an inner tunica interna (intima), a middle tunica media, and an external tunica externa (adventitia) (Fig. 7-1). Arteries and veins are distinguished from each other on the basis of the composition of the various tunics, particularly the tunica media (Fig. 7-2).
The tunica interna (tunica intima) is lined with a simple squamous epithelium known as endothelium and its underlying basal lamina. A subendothelial layer includes collagen and elastic fibers, fibrocytes, and in some vessels, smooth muscle cells. The outermost layer of the tunica interna is the internal elastic membrane. This membrane is a sheet of elastin that has gaps that permit diffusion of nutrients into the tunica media; it is usually absent in the smaller veins and thin or inconspicuous in the larger ones. The internal elastic membrane is often indistinct under the light microscope but can be identified readily by electron microscopy (Fig. 7-3). The tunica interna is avascular and is nourished through transendothelial transport of substances from the circulating blood.
The tunica media consists of several layers of smooth muscle in helical arrangement, interspersed with varying numbers of elastic laminae, elastic fibers, and collagen fibers. Most of the inner half of the tunica media receives nutrients from the tunica interna; the remainder is supplied by vasa vasorum (small blood vessels that supply the vessel wall). An external elastic membrane, similar in structure to the internal elastic membrane, is clearly distinguishable in only the largest muscular arteries (Fig. 7-1).
In the outermost tunica externa (adventitia), collagen and elastic fibers predominate, and smooth muscle cells may be present. This tunic also contains vasa vasorum that extend into the outer layers of the tunica media (Fig. 7-4). Vasomotor nerves (nervi vasorum) form plexuses in the externa of most large blood vessels. A few axons penetrate the tunica media and terminate near smooth muscle cells (Fig. 7-5).
FIGURE 7-1 Cross section through part of the wall of a canine muscular artery. The most prominent layer of the tunica interna is the internal elastic membrane (A); the tunica media consists of several alternating layers of smooth muscle cells and elastic lamellae or fibers (B). In the tunica externa, elastic fibers predominate (C). Verhoeff’s elastic stain (×130).

FIGURE 7-2 Cross section through part of the canine femoral artery (a) and vein (b). Arteries and veins are distinguished from each other on the basis of the thickness of the vascular wall and differences in the composition of the various tunics, particularly the tunica media (M). Tunica interna (I); tunica externa (E). Resorcin-fuchsin (×400).

FIGURE 7-3 Electron micrograph of part of the wall of a muscular artery (rat). The endothelium rests on a thick internal elastic membrane (A). The smooth muscle cells of the tunica media are surrounded by a basal lamina and collagen fibrils are present in the intercellular spaces (×7800).

Vascular Endothelium
The vascular endothelium is interposed between the blood in circulation and the surrounding tissues or organs. Endothelial cells have been shown to be functionally interactive with other cells in many complex ways. The endothelial cytoskeleton, a complex network of actin microfilaments, microtubules, and intermediate filaments, combines to change and transduce signals between cells and regulate cell shape (Fig. 7-6).
Endothelial cells manifest extensive heterogeneity. Morphologic heterogeneity of these cells is found in arteries versus veins and can be demonstrated by corrosion casts that exhibit details of the endothelium. In arteries, endothelial cells are usually very elongated and lie parallel to the long axis of the vessel (Fig. 7-7). In contrast, endothelial cells of veins and capillaries are more rounded and less highly oriented.
FIGURE 7-4 Scanning electron micrograph of microcorrosion cast showing bovine pododermal vein (A) with accompanying vasa vasorum (B) (×220). (Courtesy of Dr. R. Hirschberg, Berlin.)

Immuno- and glycohistochemical studies have led to the recognition of species-, organ-, and age-specific endothelial cell surface receptors. Selectivity in the seeding of metastatic cells from tumors and site-specificity of lymphocyte homing are examples of preferential adhesion to the endothelial cell surface. Structural heterogeneity such as the presence or absence of certain cellular organelles like Weibel-Palade bodies (rod-shaped bundles of microtubules in Fig. 7-8), differences in the synthesis of specific enzymes or cytokines, and variability in the production of vascular regulatory products such as prostaglandins are testimony to the complexity of the endothelium.
Arteries
Elastic Arteries
The tunica interna of elastic arteries is often thicker than the interna of other types of arteries (Fig. 7-9). The endothelial cells often appear to have a bricklike shape. The subendothelial layer contains smooth muscle cells, fibroblasts, primarily longitudinally oriented collagen fibers, and numerous fine elastic fibers. In the large domestic mammals, this layer is particularly thick. The internal elastic membrane is often split into lamellae that may merge with the elastic laminae of the tunica media.
The tunica media is the thickest of the three tunics of the vessel wall and consists primarily of concentrically arranged, fenestrated elastic laminae (Fig. 7-10). Smooth muscle cells lie between adjacent laminae to which they are attached by collagen fibrils. The amorphous ground substance is basophilic due to the high quantity of sulfated glycosaminoglycans. All intercellular fibers and ground substance of the media are synthesized by the smooth muscle cells. With increasing distance from the heart, the number of smooth muscle cells in the tunica media of elastic arteries increases and the amount of elastic tissue decreases. The external elastic membrane is either absent or indistinct.
In the tunica externa, longitudinally arranged bundles of collagen fibers predominate and are intermixed with a few elastic fibers and fibroblasts. The interlacing of the collagen fibers limits the elastic expansion of the vessel.
Transition from elastic to muscular arteries may be either gradual or abrupt. In the dog, typical muscular renal arteries arise immediately at right angles from the elastic abdominal aorta. Carotid, femoral, vertebral, and brachial arteries commonly begin as elastic arteries and gradually transform into the muscular type in peripheral regions. The site of the transitional zone for each vessel varies among species and individual animals.
Muscular Arteries
The tunica interna of muscular arteries consists of the endothelium with an underlying thin subendothelial layer composed of collagen and elastic fibers. In large muscular arteries, a few fibroblasts and smooth muscle cells are present. With decreasing vessel size, the subendothelial layer gradually becomes thinner. The prominent, thick internal elastic membrane (Figs. 7-1 and 7-3) has fenestrations through which cytoplasmic processes of endothelial cells contact the smooth muscle of the tunica media. Blood vessel tone is determined by both smooth muscle and endothelial function. The two cell types interact in a dynamic manner to regulate blood vessel diameter.
Muscular arteries are characterized by a thick tunica media, composed mainly of smooth muscle cells in the form of circular or helical wrappings from three to more than 40 cell layers in thickness. Interspersed between these smooth muscle cells are elastic fibers or lamellae, as well as collagen fibers (Figs. 7-1, 7-3, and 7-10). The external elastic membrane is often discontinuous and not always clearly defined. It consists of a dense feltwork of elastic fibers adjacent to the tunica externa (Fig. 7-1).
FIGURE 7-5 Longitudinal section through a bovine pododermal arteriole (A). Nervi vasorum (B) form a plexus around the vessel and few axons penetrate the tunica media. Acetylcholinesterase counterstained with nuclear fast red (×100). (Courtesy of Dr. S. Buda, Berlin.)

FIGURE 7-6 Cytoskeleton of endothelial cells, cultured in vitro and labeled by immunocytochemistry (i.e., a fluorescein-coupled monoclonal antibody to actin) (×600).

The tunica externa is composed of collagen fibers, fibroblasts, and elastic fibers that decrease in number with decreasing size of the vessel.
When living tissues are prepared for fixation or when an animal dies, the muscular arteries contract considerably and blood is forced out of the lumina into the more expandable venous circulation. The tunica interna, including the underlying internal elastic membrane, and the external elastic membrane are thrown into longitudinal folds. Consequently, the cross-sectional profiles of muscular arteries in most histologic preparations have relatively small lumina containing little blood, the elastic membranes appear scalloped, and endothelial cells have a bricklike shape (Fig. 7-1).
FIGURE 7-7 Scanning electron micrograph of microcorrosion cast showing bovine pododermal artery with elongated endothelial cell imprints (A) lying parallel with the long axis of the vessel (×320). (Courtesy of Dr. R. Hirschberg, Berlin.)

FIGURE 7-8 Electron micrograph of a porcine endothelial cell of the chorioid containing a so-called Weibel-Palade body (A), longitudinally cut. These endothelial-specific bundles of microtubules are located in the cytoplasm. Cell nucleus (B) (×150,000).

FIGURE 7-9 Cross section through part of the wall of the equine thoracic aorta. A particularly thick subendothelial layer (S) contains many longitudinally oriented elastic fibers. In the tunica media (M), elastic laminae predominate. Resorcin–fuchsin–van Gieson’s stain (×50). (Courtesy of A. Hansen.)

FIGURE 7-10 Electron micrograph of part of the tunica media of the aorta (rat). Smooth muscle cells (A) surrounded by collagen fibrils (arrowheads) alternate with elastic laminae (B) (×9000).

Microvasculature
In its simplest form, the microvasculature comprises afferent arterioles that lead to capillary networks drained by efferent venules. Shunts may exist between arterioles and venules in the form of either arteriovenous anastomoses or central channels that create preferential flow through capillary beds. In many organs, the microvasculature has unique architectural and structural characteristics. Examples of exceedingly intricate and extremely convoluted microcirculation are found in the lung, hoof, and claw (Fig. 7-11).
Arterioles
The tunica interna of arterioles consists of endothelium, a thin subendothelial layer of collagen and elastic fibers (which is absent in the smallest arterioles), and an internal elastic membrane. The internal elastic membrane is fenestrated and eventually disappears in the smallest arterioles, allowing basal processes of the endothelial cells to establish direct contact with the underlying smooth muscle cells of the tunica media. In addition to one to three layers of smooth muscle cells, the tunica media may also contain collagen fibers (Fig. 7-12). An external elastic membrane is absent. The tunica externa is loose connective tissue.
Capillaries
Capillaries are tubules of uniform diameter that are approximately 8 µm wide (ranging from 5 to 10 µm). The walls of capillaries are composed of endothelial cells, an associated basal lamina, pericytes, and a thin adventitial connective-tissue layer that is lacking around brain capillaries (Figs. 7-13 and 7-14). A tunica media is absent.
Pericytes of capillaries and postcapillary venules are basal lamina–enclosed cells with numerous processes. They are considered to be undifferentiated mesenchymal cells that can be readily stimulated to divide mitotically and to migrate around or away from the vessels. Pericytes may play a critical role in blood vessel development and maturation. These cells are believed to transform into other cell types, especially fibroblasts and smooth muscle cells. Apparently a capillary can transform by this means into other types of vascular tubes if the internal flow characteristics change. Indeed, this description is compatible with the method by which arteries and veins develop during embryogenesis. All blood vessels start as simple, endothelium-lined capillarylike tubes during vasculogenesis, which is the de novo differentiation of blood vessels from mesodermal precursor cells, or during angiogenesis, which is the formation of new blood vessels by migration and proliferation of endothelial cells from preexisting vessels. Recent studies have shown that, in the presence of various soluble factors, it is possible to activate or inhibit capillary growth in certain tissues.
FIGURE 7-11 Scanning electron micrograph of microcorrosion cast of bovine pododermal arteriole (A) with branching convoluted capillaries (B) (×70). (Courtesy of Dr. R. Hirschberg, Berlin.)

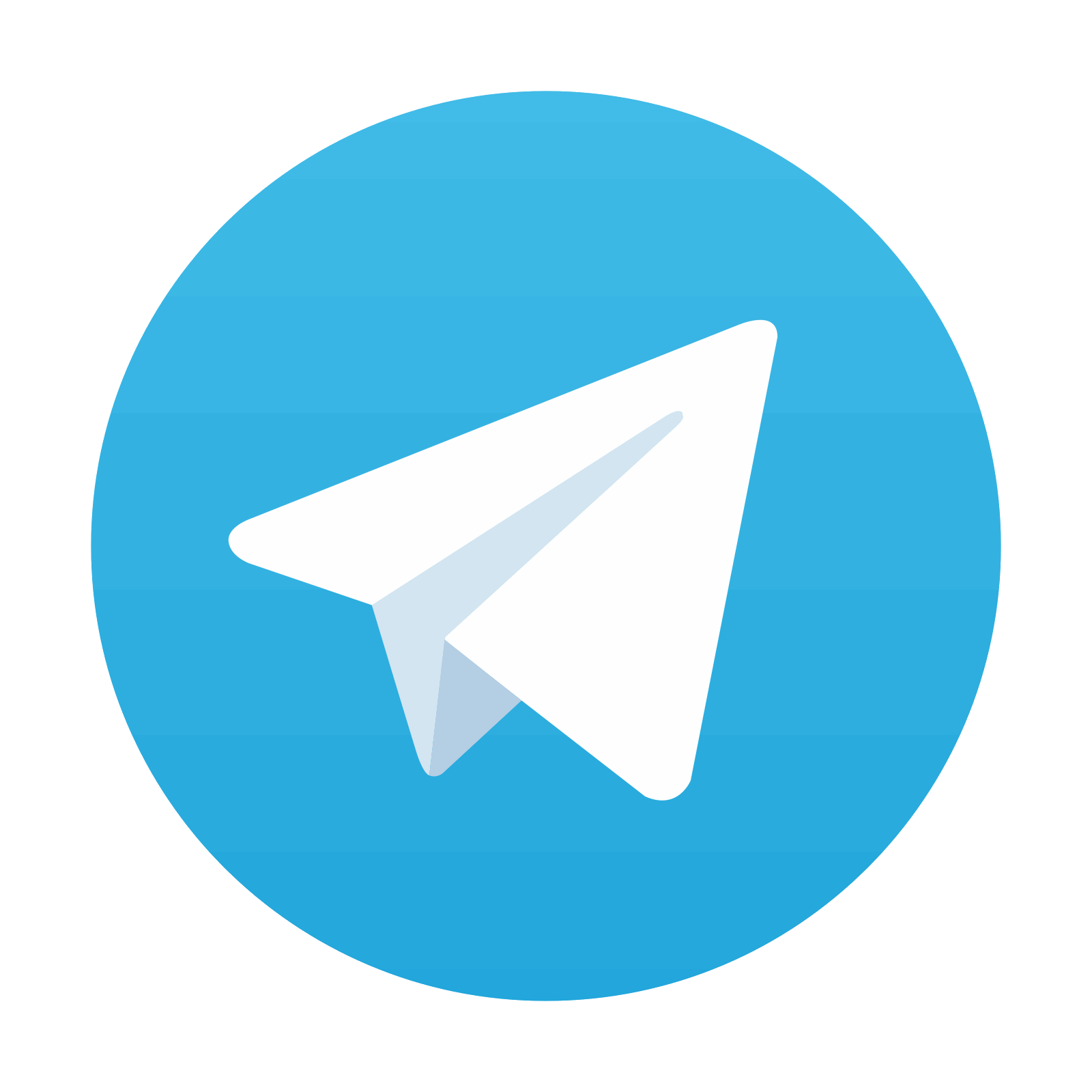
Stay updated, free articles. Join our Telegram channel

Full access? Get Clinical Tree
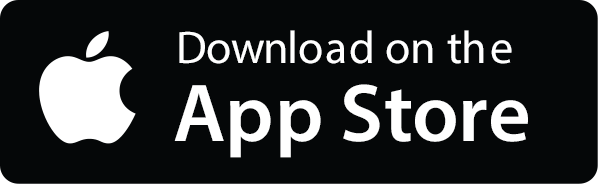
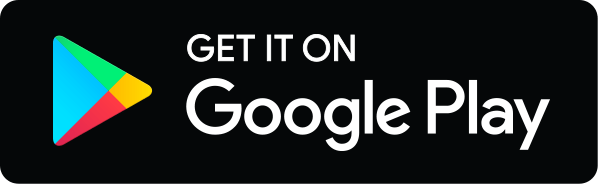