Chapter 10. Carbohydrate Metabolism
DIETARY REQUIREMENT FOR CARBOHYDRATE
The dog is capable of meeting its metabolic requirement for glucose from gluconeogenic pathways throughout growth and adult maintenance, provided that sufficient fat and protein are included in the diet. 1 However, the need for an exogenous source of carbohydrate during the metabolically stressful periods of gestation and lactation has been debated. During gestation the female dog’s needs increase because glucose provides a major energy source for fetal development. Similarly, during lactation, additional glucose is needed for the synthesis of lactose, the disaccharide present in milk. It is assumed that the female cat’s glucose requirement also increases during these physiological periods.
An early study with dogs examined the degree of reproductive success in females that were fed diets with varying levels of carbohydrates. 2 The data indicated that pregnant dogs did require a source of carbohydrate to whelp and rear healthy puppies. Dogs that had been fed a carbohydrate-free diet throughout gestation became hypoglycemic and ketotic near the end of their pregnancies, and also showed reduced blood concentrations of the amino acid alanine. Only 63% of their puppies were alive at birth, and puppy mortality was high shortly after birth. However, these results were subsequently refuted by data from a second study that also examined the effects of feeding a carbohydrate-free diet to female dogs throughout gestation and lactation. 3 These data indicated that a carbohydrate-free diet did not affect duration of gestation, litter size, litter weight, or puppy viability. Ultimately, the difference in the results of the two experiments was attributed to differences in the protein levels of the diets that were fed. The diet in the first study contained only 26% protein, compared with 51% and 45% protein diets in the second set of experiments. Feeding a higher protein diet provides sufficient amounts of gluconeogenic amino acids to allow the maintenance of plasma glucose levels despite the heavy demands of gestation and lactation. Alanine, glycine, and serine appear to be the principal gluconeogenic amino acids in dogs. 4 The reduced blood levels of alanine exhibited by the dogs in the first study suggest that insufficient alanine was available to allow adequate gluconeogenesis. The hypoglycemia observed in these dogs was probably a result of the lack of gluconeogenic precursors rather than an innate inability to synthesize sufficient glucose during gestation and lactation.
These results were further supported by a study that examined the ability of varying levels of dietary protein to ameliorate the effects of carbohydrate-free diets on gestation and lactation. 5 These data confirmed that feeding carbohydrate-free diets to pregnant and lactating dogs can cause adverse effects. However, performance was not impaired when the protein level in the diet was sufficiently high. The investigators estimated that if carbohydrate is provided in the diet, female dogs require about 7 grams (g) of digestible crude protein per unit of metabolic body weight. However, if no carbohydrate is supplied in the diet, this protein requirement must be increased to approximately 12 g of protein. Lactating females appear to require between 13 and 18 g of protein per unit of metabolic weight when fed a diet containing carbohydrate and 30 g when fed a carbohydrate-free diet. This information indicates that although glucose is a metabolically essential nutrient for the dog, carbohydrates are not an indispensable component of the diet, even during the metabolically demanding stages of gestation and lactation. Although specific studies have not been conducted during pregnancy and lactation in the cat, this species’ unique pattern of gluconeogenesis, coupled with its carnivorous nature, suggests that it too can survive all stages of life while consuming a carbohydrate-free diet.
Although glucose is a metabolically essential nutrient for the dog and the cat, digestible carbohydrates are not an indispensable component of the diet. Dogs, and probably cats, can synthesize adequate glucose from gluconeogenic pathways to meet their metabolic needs throughout life, provided that sufficient protein is included in the diet to supply gluconeogenic amino acids.
CARBOHYDRATE METABOLISM IN CATS
Compared with the dog and other omnivorous species, the cat has several unique mechanisms for metabolizing dietary carbohydrate. The cat’s ability to maintain normal blood glucose levels and health when fed a carbohydrate-free diet is partly related to its unique pattern of gluconeogenesis. In most animals, maximal gluconeogenesis for the maintenance of blood glucose levels occurs during the postabsorptive state, when dietary soluble carbohydrate is no longer available. However, carnivorous species are similar to ruminant species in that they maintain a constant state of gluconeogenesis with a slightly increased rate immediately after feeding. 6 Because the body is limited in its ability to conserve amino acids, and a carnivorous diet typically contains little soluble carbohydrate, the immediate use of gluconeogenic amino acids for the maintenance of blood glucose levels is an adaptive advantage.
The enzyme activity values in the cat’s liver indicate that gluconeogenic amino acids in the diet are deaminated and converted to glucose, rather than being directly oxidized for energy. 7 Liver phosphoenolpyruvate carboxykinase (PEPCK), a major gluconeogenic enzyme, does not change in activity level when cats that were previously fed high-protein diets are subjected to fasting. 8 In addition, no significant changes in hepatic PEPCK activity occur when cats are switched from a low-protein diet (17.5%) to a high-protein diet (70%). 9 These data support the supposition that the hepatic gluconeogenic enzymes in cats always have a high rate of activity, necessitating the rapid conversion of excess dietary amino acids to glucose. This lack of enzymatic adaptation to change in dietary protein concentration is also seen in other carnivorous species, including trout, vultures, and barn owls. 10.11. and 12.
There are also differences between cats and omnivores in the relative importance of various gluconeogenic and carbohydrate-metabolizing pathways. Compared with omnivorous species, the cat has a high hepatic activity of the enzyme serine-pyruvate aminotransferase and low activity of the enzyme serine dehydratase. 9. and 13. It appears that the cat is able to convert the amino acid serine to glucose by a route that does not involve either pyruvate or serine dehydratase. An alternative pathway has been proposed for the conversion of serine to glucose. 14 It has been observed that a high activity of the first enzyme in this alternative pathway, serine-pyruvate aminotransferase, appears to be associated with carnivorous dietary habits in mammals.
After glucose is absorbed into the body, it must be phosphorylated to glucose-6-phosphate before it can be metabolized. The liver of most omnivorous animals, including the domestic dog, has two primary enzymes that catalyze this reaction: glucokinase and hexokinase. Hexokinase is active when low levels of glucose are delivered to the liver, and glucokinase operates whenever the liver receives a large load of glucose from the portal vein. The cat’s liver has active hexokinase but has minimally active glucokinase. 15 The hepatic glucokinase that is present functions at a very low rate, and its activity cannot be up-regulated in response to a large intake of carbohydrate. Interestingly, recent studies show that, compared with dogs, the activity of feline hepatic hexokinase and two other glycolytic enzymes, phosphofructokinase and pyruvate kinase, are relatively high. 16 It is possible that the high activity rates of these enzymes compensate for the low activity of glucokinase in the cat’s liver. Cats also show minimal activity of hepatic glycogen synthetase, an enzyme essential for converting glucose to glycogen for storage in the liver. 17 Together these metabolic patterns may limit the cat’s ability to rapidly minimize the hyperglycemia that occurs after a large dietary glucose load. Conversely, the cat’s carbohydrate-metabolizing enzyme patterns support an ability to maintain normal blood glucose levels through the consistent delivery of glucose via gluconeogenic catabolism of amino acids.
< div class='tao-gold-member'>
Only gold members can continue reading. Log In or Register a > to continue
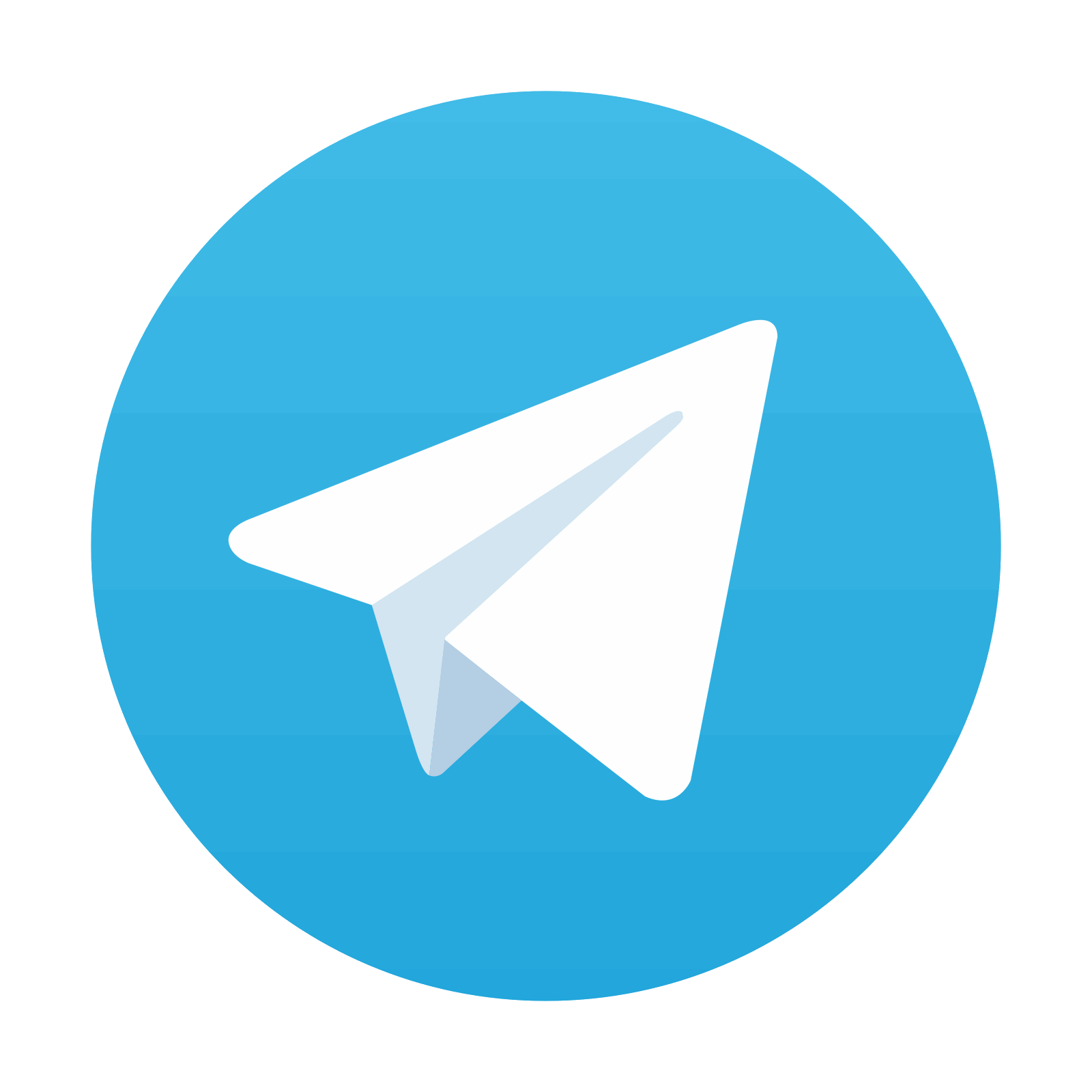
Stay updated, free articles. Join our Telegram channel

Full access? Get Clinical Tree
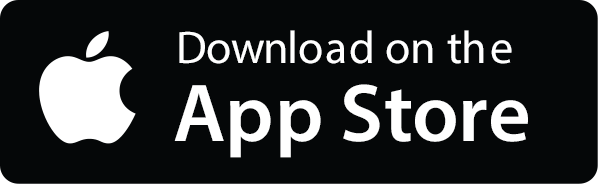
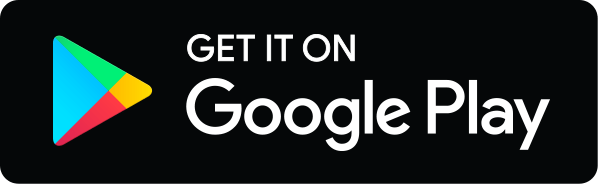