12 Bovine Ephemeral Fever Virus 1Institute of Molecular Biology, National Chung Hsing University, Taiwan; Bovine ephemeral fever virus (BEFV) is an arthropod-borne rhabdovirus, which has been classified as the type species of the genus Ephemerovirus (Wunner et al., 1995). This genus includes the type species BEFV and antigenically related species Adelaide River virus (ARV) and Berrimah virus (Tordo et al., 2008). Like other rhabdoviruses, BEFV virions are bullet- or cone-shaped (Murphy et al., 1972), consisting of a ribonucleoprotein complex and an envelope and containing a negative single-stranded RNA genome and five structural proteins (Della-Porta and Brown, 1979; Walker et al., 1991). It occurs in tropical and subtropical regions of Africa, Asia, Australia and the Middle East, causing a disabling febrile infection in cattle and water buffalo (St George, 1990). The other recognized ephemero-viruses are not known to cause disease. BEFV is serologically related to several other rhabdoviruses including ARV, Berrimah virus and Kimberley virus (Calisher et al., 1989) and these have also been grouped in the genus Ephemerovirus (Wunner et al., 1995). BEFV consists of a single-stranded, negative-sense RNA genome. The negative-strand rhadovirus RNA contains five to six genes in the order 3′-l-N-P-M-G-G(NS)-α1-α2-β-γ-L-t-5′ (McWilliam et al., 1997). The genes encode five structural proteins, including the large RNA-dependent RNA polymerase (L), polymerase-associated protein (P), envelope glycoprotein (G), nucleoprotein (N) and matrix protein (M) (Walker et al., 1991). Between the G and L genes, BEFV and ARV contain multiple open reading frames (ORFs) encoding a non-structural transmembrane glycoprotein (GNS), a putative viroporin (α1) and several other small proteins (α2 and β in ARV; α2, α3, β and γ in BEFV) of unknown function (Walker et al., 1991; McWilliam et al., 1997). Despite the complex genome organization, sequence comparisons of G, GNS, N and 3′-leader sequences have revealed that BEFV and ARV are most closely related to vesicular stomatitis virus (VSV) and other vesiculoviruses (Walker et al., 1992, 1994; Wang and Walker, 1993; Wang et al., 1995). Although there appear to be differences in phosphorylation, sequences of ephemerovirus P and M proteins are also most closely related to vesiculoviruses (Wang et al., 1995; Dhillon, 1996). To initiate a productive infection, all viruses must translocate their genome across their host-cell membrane (Smith and Helenius, 2004). Cell membrane is a barrier for outcoming sources, which include viruses. Virus penetration can take either by fusing with the plasma membrane directly to release viral capsids into cytosol or by endocytosis (Marsh and Helenius, 2006). Therefore, virus must bind to their host-cell surface, followed with signaling induction in order to penetrate into cells. The virus binding and entry of BEFV are similar to that of other membranes of the Rhabdoviridae, such as VSV. The glycoprotein G of VSV is involved in receptor recognition at the host-cell surface. It is suggested that G protein is distinct from both class I and II viral fusion proteins that had been already described (Gaudin, 2000; Roche et al., 2008). After endocytosis of the virion, fusion is triggered by the low pH of the endosomal compartment and is mediated by the viral glycoprotein. G is an atypical fusion protein, as there is a pH-dependent equilibrium between its pre- and post-fusion conformations. In fact, the reversibility of the fusogenic low-pH-induced conformational change is crucial to allow G to be transported through the acidic compartments of the Golgi apparatus and to recover its native, pre-fusion state at the viral surface (Gaudin et al., 1995). Virus entry often uses multiple pathways and is cell-type specific, due to the component diversity in either membrane or organelles that might limit virus entry ability. Studies by Cheng et al. (2012) suggested that BEFV entry follows a clathrin-mediated and dynamin 2-dependent endocytosis pathway. BEFV infection also depends on endosomal acidification and on dynamin GTPase activity, which is a hallmark of clathrin-mediated endocytosis (Hinshaw, 2000). After entry, BEFV requires Rab5 and Rab7 for transport to early and late endosomes for infection. Cheng et al. (2012) demonstrated that microtubules disruption impairs BEFV infectivity, because early endosomal transport requires microtubules and Rab5, suggesting that BEFV requires transport to early endosome for infection. Furthermore, data obtained from Cheng and colleagues also demonstrated that BEFV requires trafficking through early and late endosomes. In contrast to BEFV, VSV does not require late endosome function for infection (Sieczkarski and Whittaker, 2003). Bovine ephemeral fever (BEF) occurs frequently in Australia, Africa and Asia, including China, Taiwan and the Middle East (Wang et al., 2001; Walker, 2005). The disease became enzootic in many countries, including Australia, China and Taiwan. In Asia, the Middle East and Africa, BEF occurs as epizootics, which originate in enzootic tropical areas and sweep north or south to sub-tropical and temperate zones. They are many possible transmission routes for arboviruses, such as wind and animal transport. The main routes of transmission between countries and continents have been studied (Aziz-Boaron et al., 2012). Both winds and animal transport were found to be important factors in trans-boundary transmission of BEFV. For example, the Egyptian isolate grouped phylogenetically with the Taiwanese isolates, coinciding with results on importation of cattle from China to the Middle East in the year preceding the isolation of the Egyptian isolates (Aziz-Boaron et al., 2012). BEF is most prevalent during rainy seasons when flying insects are numerous, and the spread may be affected by wind movement. The causative virus is transmitted by haematophagous biting insects, probably mosquitoes or midges that can be carried on the wind allowing rapid spread of the disease. It was reported that BEFV can be isolated from various species of mosquitoes and culicoides (Davies and Walker, 1974; Blackburn et al., 1985). Studies on models for arbovirus dispersion by winds may help in quantifying the risk of BEFV transmission in Australia, Africa and Asia, as well as its invasion to other geographical locations, including Europe (Hendrickx et al., 2008; Ducheyne et al., 2011). BEF is an inflammatory disease and is characterized by some common pathological lesions, including serofibrinous polysinovitis, polyarthritis, polytendovaginitis, cellulitis and focal necrosis of skeletal muscles. The lungs may show fatty oedema and lymph nodes are oedematous. Lesions in the upper cervical region of the spinal cord were also seen (Hill and Schultz, 1977). Histopathology reveals neutrophilia, leukocytosis and high fibrinogen level. A reduction in erythrocyte number in the early part of the infection followed by a larger fall corresponds to haemosiderosis of lymph node and spleen. Lesions have also been found in venules and capillaries in tendon sheath, synovial membranes, muscle, fascia and endothelium, along with perivascular neutrophilic infiltration, focal or complete necrosis of vessel walls, thrombosis and perivascular fibrosis (Basson et al., 1970). BEF causes an acute febrile disease in cattle and water buffalo. Neutralizing antibodies have also been found in other species of African wildlife (Davies et al., 1975). BEF is widespread throughout Asia, Australia, Africa and the Middle East, and is commonly known as ‘ephemeral fever’ or ‘three-day stiffness sickness’ because of the immobilization of infected animals for 3–5 days following the height of viraemia and fever (Bevan, 1912; St George, 1994). Accumulating evidence indicates that clinical signs of BEF, which include bi-phasic fever, anorexia, muscle stiffness, ocular and nasal discharge, ruminal stasis and recumbency, are due primarily to a vascular inflammatory response (Nandi and Negi, 1999; Walker, 2005). Its economic effect is mainly due to mortality and severe reduction in milk production in dairy herds, loss of draught animals at the time of harvest and loss of condition in beef cattle. The reduction in milk production ranges from 34% to 95%, with an average of 46%. The milk yield did not return to pre-illness level on convalescence. There is some loss from abortion in females (Theodoridis et al., 1973) and males may suffer from temporary infertility (Parsonson and Snowdown, 1973). Although recovery may be complete, a permanent drop in milk production in cows and reduced fertility in bulls often occurs, resulting in heavy economic losses (St George, 1994). In addition, BEFV poses a severe threat to both national and international trade of animals. Many countries require imported cattle and buffalo to be free from BEF-neutralizing antibodies. It is expensive to keep bulls whose semen is to be exported in insect proof areas and to monitor the evidence of BEFV infection continuously (Walker and Cybinski, 1989). Endocytosis is required for a number of cellular functions, including pathogen entry, antigen presentation, nutrient uptake, drug delivery, neurotransmission, membrane receptor recycling, cell adhesion and migration, cell polarity, mitosis, cell growth and cell differentiation. Many viruses have evolved to utilize endocytosis to enter their host cells after initial binding of virions to receptor(s) on the cell membrane. After attachment to their host-cell surface receptor(s), internalization usually follows directly from the plasma membrane or via endocytosis. To date, a number of different routes of endocytosis used by viruses have been demonstrated. The best understood endocytic pathways include clathrin-dependent and lipid raft/caveolae-dependent entry pathways. Clathrin-mediated endocytosis is a major endocytic pathway from the plasma membrane to early endosomes. In clathrin-mediated endocytosis, clathrin is assembled on the inside face of the plasma membrane to form a characteristic coated pit (CCP) and in response to receptor-mediated internalization signals. Once assembled, CCPs pinch off from the cell membrane and mature into clathrin-coated vesicles (Keen, 1990), which then transport the cargo into endosomes. Dynamin is critical for clathrin- or caveolae-mediated endocytosis and phagocytosis, but it is not necessary for macropinocytosis (Henley et al., 1998; Huang et al., 2011). A number of viruses have been discovered to enter their host cells through the clathrin-dependent endocytosis pathway (Helenius et al., 1980; Sieczkarski and Whittaker, 2005; Sun et al., 2005). Many other viruses have also been reported to enter their host cells through the caveolae-dependent pathway (Bousarghin et al., 2003; Huang et al., 2011). The specific cell pathways involved in cell entry of BEFV have been determined (Cheng et al., 2012). By using a fluorescently lipophilic dye, 3,30-dilinoleyloxacarbocya-nine perchlorate (DiO) labelled-BEFV, Cheng et al. (2012) demonstrated that clathrin-mediated and dynamin 2-dependent endocytosis in a pH-dependent manner is an important avenue of BEFV entry. After endocytosis, Rab 5 and Rab 7 were activated and involved in early and late endosomes that are required for endocytosis and subsequent infection in cells. Treatment of BEFV-infected cells with nocodazole significantly decreased the viral protein synthesis and viral yield, suggesting that microtubules play a critical role in BEFV productive infection likely by mediating trafficking of BEFV-containing endosomes. Several viruses rely on activation of the PI3K-Akt pathway for efficient replication or long-term persistence. Similar to findings with other non-segmented, negative-sense RNA viruses (NNSVs) (Sun et al., 2008), inhibition of Akt through the use of Akt inhibitors had negative effects on BEFV replication, suggesting that activation of Akt is required for BEFV propagation (Ji et al., 2011). Interestingly, suppression of PI3K or mTOR complex 1 (mTORC1) through the use of inhibitors (wortmannin, LY294002 and rapamycin) increased BEFV replication (Ji et al., 2011). Some viruses, such as hepatitis B virus (HBV) and hepatitis C virus (HCV), which are persistent viruses, can activate PI3K-Akt-mTOR signaling to promote cell survival and long-term infection. Inhibition of PI3K, Akt or mTOR slightly up-regulates replication of these two viruses (Mannova and Beretta, 2005; Guo et al., 2007). Unlike HBV and HCV, BEFV possesses a different survival strategy, as seen from its reliance on Akt for efficient replication but not PI3K and mTORC. BEFV might maintain Akt activity to slow down cell death and prolong viral infection. To date, several forms of live-attenuated, inactivated and recombinant vaccines have been reported but with variable efficacy and durability of protection. The G protein of BEFV is a highly effective vaccine antigen, either as a purified subunit or expressed from recombinant viral vectors (Hertig et al., 1996; Walker, 2005; Johal et al., 2008). Natural BEFV infection induces a strong neutralizing antibody response and infection usually induces durable immunity. It is necessary to replace current live-attenuated virus vaccines that are sensitive to heat, especially in Africa where maintenance of a continuous cold-chain is quite difficult. During major outbreaks, involving entire herds, the diagnosis is primarily based on clinical outcomes and history of the outbreak. However, individual cases are difficult to diagnose (Uren et al., 1992). A differential leukocyte count on the blood smear provides the most rapid supporting evidence for field diagnosis, but a high percentage of neutrophils with many immature forms are not pathognomonic of ephemeral fever. Sporadic cases, or cases occurring early in a possible epidemic, can be confirmed by virus isolation and identification (Tzipori, 1975) or serology to detect specific antibodies in paired serum samples (Nandi and Negi, 1999). There are several immuno-logical methods established for detection of specific antibodies to BEFV, including a blocking ELISA (Zakrzewiski et al., 1992; Hsieh et al., 2005). BEF virus antigen can be detected in blood leukocyte films prepared from feverish animals by immunofluorescent test (Zaghawa et al., 2002). However, a sensitive, reliable and quantitative technique for serological diagnosis of BEFV is currently lacking. Currently, virus isolation seems to be the standard method for BEF diagnosis. In recent years, nucleic acid detection has become a standard technique for monitoring virus infection. Molecular diagnostic assays have been reported to detect the genome of BEFV (Table 12.1). A real-time RT-PCR assay, targeting the glycoprotein (G) gene of BEFV, has been developed by Stram et al. (2011). This assay appears to be sensitive and has a detection limit of 10 copies of BEFV genome in a clinical sample. Using RT-PCR, a primer designed to amplify 438 nucleotide fragments from the G gene, allowed specific amplification of BEFV-cell culture isolates collected from Egypt and Japan. Zaghloul et al. (2012) used same gene spot successfully in Dot-blot hybridization assay. A rapid, sensitive and specific assay has been developed to detect and quantify the G protein-encoding gene of BEFV known as ‘a reliable assay protocol for identification of diseases (RAPID)-bioactive amplification with probing (BAP) assay’ (Hsieh et al., 2005). This technique combines a nested PCR and magnetic bead-based DNA probing assay. By using this technique, a minimum of 1 copy/μl of the BEFV plasmid DNA could be detected. Furthermore, Hsieh et al. (2005) have compared the sensitivity of the RAPID-BAP assay, real-time RT-PCR, and conventional RT-PCR in the detection of clinical blood samples suspected to have BEFV infections, and the results revealed that the RAPID-BAP assay was more sensitive than the conventional RT-PCR and real-time RT-PCR assays for the detection of BEFV. The novel RAPID-BAP assay is an excellent diagnostic tool with high sensitivity and specificity. Another assay, reverse transcription loop-mediated isothermal amplification (RT-LAMP), again targeting the G gene of BEFV, has also been developed. The advantage of RT-LAMP is that no specialist equipment is required because there is no requirement for thermal cycling (Zheng et al., 2011). However, a range of specialist apparatus is now available specifically for application with RT-LAMP such as lateral flow devices and turbidometers. Given the above, current available assays could not quickly and completely detect BEFV. A high-speed assay with high specificity and sensitivity to detect BEFV from available samples is urgently needed. Table 12.1. Features of the available PCR formats for the detection of BEFV.
2The Pirbright Institute, Compton Laboratory, Compton, UK
12.1 Introduction
12.2 Virus Biology
12.2.1 Genome organization and coding assignment
12.2.2 Virus life cycle
12.3 Pathogenesis
12.3.1 Virus transmission
12.3.2 Pathology
12.3.3 Clinical outcome
12.4 Molecular Pathogenesis
12.4.1 Virus entry
12.4.2 Virus–host interactions and virus-induced apoptosis
12.4.3 Immune response
12.5 Diagnosis
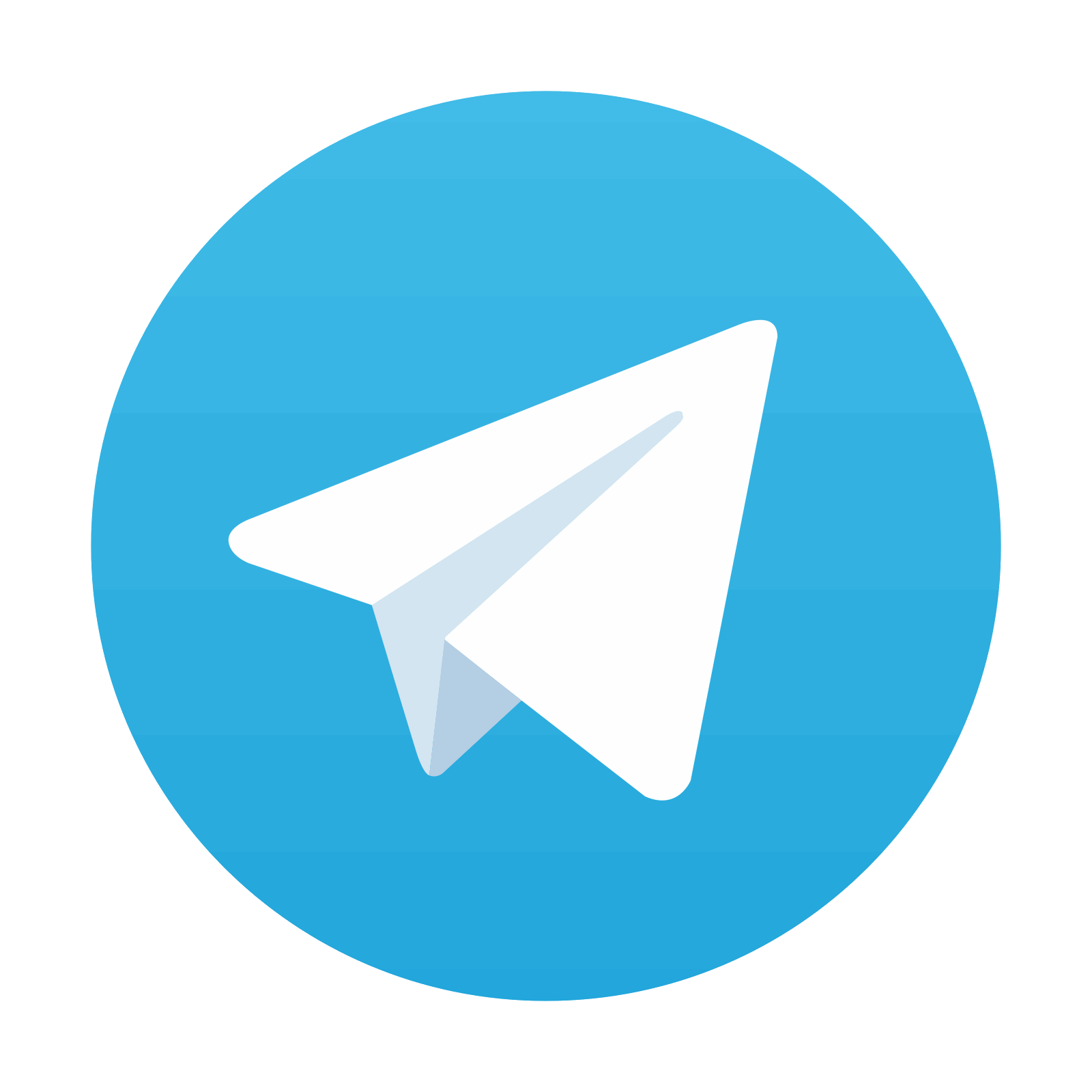
Stay updated, free articles. Join our Telegram channel

Full access? Get Clinical Tree
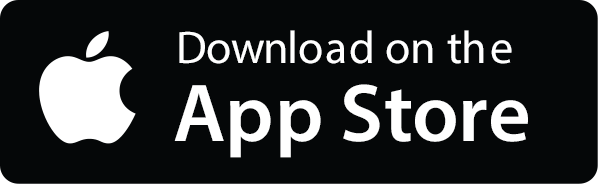
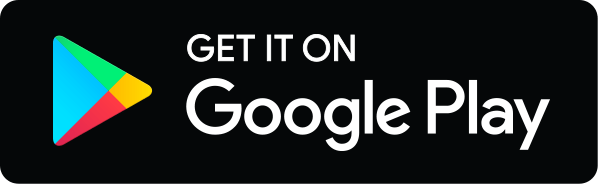