26 Caroline Le Maréchal, Cédric Woudstra, and Patrick Fach Botulism is a severe, flaccid neuroparalytic disease of humans and animals. Animal botulism was first described at the beginning of the 20th century, although it is thought to have occurred long before that. It affects most mammals, birds, and fish. Animal botulism is a major environmental and economic concern, mainly because of the high mortality rate during outbreaks. It is considered to be a zoonosis, as it may be a source of food-borne botulism for humans. It is also considered a potential bioterrorism threat. Botulism can be caused by one of seven different neuroparalytic toxin subtypes (A to G). These botulinum neurotoxins (BoNTs) are produced by anaerobic, Gram-positive clostridia, mainly Clostridium botulinum. All BoNT subtypes act at the neuromuscular junction, blocking the release of acetylcholine and leading to flaccid paralysis. BoNT types A, B, E, and, more rarely, type F, are mainly responsible for human botulism, whereas toxin types C and D and their mosaic variants are mostly involved in animal botulism worldwide. Cases of animal botulism caused by BoNT types A, B, and E have also been reported. BoNTs are proteins of approximately 150 kDa, composed of two subunits linked by a disulfide bond: a light chain (50 kDa) and a heavy chain (100 kDa). The light chain is a zinc protease that cleaves the SNARE protein, interfering with the exocytotic cell mechanism and preventing release of acetylcholine in the synaptic cleft. The heavy chain is involved in binding to the receptor on the neuronal membrane and the translocation of the light chain into nerve cells. BoNTs are absorbed from the intestine by binding to receptors on the apical surface of gut epithelial cells. Absorption may also occur in the stomach. Then, BoNTs reach the nerve endings via the bloodstream. Binding of type C BoNT to the receptor is ganglioside-dependent, but binding of type D toxin is not. C. botulinum strains can be divided into four groups based on their genotypic and phenotypic characteristics. Group I and II strains are mainly involved in human disease, while group III strains are associated with animal disease. Sequences of several C. botulinum group III strains have been published recently and revealed that C. botulinum group III strains, C. novyi, and C. haemolyticum are closely related. Given these genetic results, it has been suggested that a new genotypic name be created, C. novyi sensu lato for C. botulinum group III, C. novyi, and C. haemolyticum. Group IV strains produce the type G toxin and have been reclassified as the new species Clostridium argentinense. Group III strains produce type C, D, and mosaic toxin types C/D and D/C. These toxins are encoded by genes carried by bacteriophages in plasmids. These pseudolysogen circular plasmid prophages do not integrate into the genome. Mosaic toxins were first described in 1996. The mosaic-type gene comprises two-thirds of one gene and one-third of the other gene. Type C/D BoNTs are composed of the light chain of type C toxin and the heavy chain of the type D toxin; type D/C toxin consists of the light chain of type D toxin and the heavy chain of the type C toxin. BoNTs are produced during vegetative growth. Suitable growth conditions include anaerobiosis, the presence of a protein source, sufficient temperature (25–42 °C), and moisture. C. botulinum is not able to synthesize some essential amino acids, so high protein substrates are essential. Decaying matter, such as decomposing cadavers or other rotting organic matter, offers ideal conditions for growth of C. botulinum. This microorganism can survive extreme environmental conditions; spores remain viable for years. Two main routes of exposure to BoNTs have been identified: Ingestion of preformed toxins has been considered the main exposure route in cases of animal botulism. However, it seems that in vivo production of BoNTs has also to be considered as a route of exposure. Cattle, horses, and poultry are more likely to be affected by this form of botulism. In Japan, it has recently been suggested that bovine botulism results from ingestion of spores that germinate, multiply, and produce BoNT in the bovine gastrointestinal tract. It has been suggested that avian botulism (especially in broiler chickens) is mainly due to BoNT production in the cecum, as a high level of type C toxin is necessary to induce botulism (106.3 mouse lethal doses [MLD/kg] for 8-week-old broilers) and such levels of toxins are not found in the environment, supporting the theory of in vivo toxin production. Animal botulism is not a notifiable disease in most countries. Lack of accurate knowledge of outbreaks likely results in underestimation of the incidence of animal botulism. In addition, difficulties in evaluating cases of the disease include limited knowledge of the disease by some veterinary practitioners and lack of laboratories performing diagnostic analyses. Botulism is classified as an emerging disease in Europe at present, and sporadic, sometimes massive outbreaks have been reported around the world in recent years. This increase in the number of cases in the last decade may be linked to the decrease in antibiotic use in animal feed. Mosaic forms are the most common BoNTs causing animal botulism in Europe and in Japan. It is not known whether mosaic types are emerging or if their recent identification is due to the availability of more specific detection methods. Table 26.1 presents the occurrence of BoNT types involved in affected species during botulism outbreaks. Table 26.1 Occurrence of BoNT types in animal botulism BoNT C/D seems to be the prevalent type involved in avian botulism in Europe and the Americas at the moment. It has also been reported in Japan. Tools allowing the identification of mosaic BoNTs have been developed recently. Mosaic BoNTs may be involved in avian botulism in other parts of the world, but this has not been confirmed. Birds are considered to be fairly resistant to type D BoNT (approximately 320,000 MLD/kg are required to produce disease in poultry), but some D or D/C outbreaks have been reported in poultry. Type E avian botulism outbreaks are also reported, mainly around the North American Great Lakes, where it causes large-scale mortality among birds and fish. Some type E botulism outbreaks were also described in broiler flocks in France in the 1990s, but no type E outbreak in poultry flocks has been reported in the literature since then. Gallinaceous birds are more susceptible to type C/D BoNT than to type C. The LD50 for type C BoNT in chickens via intravenous inoculation is 16,000 MLD/kg, in peafowl 2700 MLD/kg, in turkeys 320 MLD/kg, and in pheasants 60 MLD/kg. Type C botulism has been diagnosed in water birds in at least 28 countries around the world, although it is rare in the tropics. Botulism has already been reported in 264 bird species representing 39 families. It often affects waterfowl but sometimes also fish-eating birds, and it is the most significant disease of migratory birds, notably waterfowl and shorebirds. Millions of birds have been killed by botulism type C during outbreaks in Canada or the USA, and outbreaks with losses exceeding 50,000 birds have been commonly reported. The effect of botulism on local populations can be significant and may cause important declines in some endangered species, impeding conservation efforts. For example, nearly 15% of the western population of American white pelicans died during a botulism outbreak in 1996. Botulism outbreaks are also reported in poultry production units. An increase in the number of outbreaks in poultry farms, notably in broilers, was reported in Sweden, Denmark, Norway, and France in 2007 and 2008. For example, according to the French epidemiological surveillance network for poultry, 121 botulism outbreaks were reported in 2007 in France, whereas only 28 cases were reported in 2006. In broilers and turkeys, 87 cases were reported between 2000 and 2006, while 393 cases were reported between 2007 and 2011. Production types mostly affected by botulism are broiler chickens and turkeys, but outbreaks in pheasants, layer hens, ducks, geese, and guinea fowl have also been reported. Not all avian species are susceptible to BoNTs; many of the carrion-eating species are resistant. Botulism in cattle was first described in South Africa in 1920. Bovine botulism outbreaks have been reported throughout the world (Europe, North and South America, Africa, Oceania, and Asia). Types D and D/C are the most common BoNT types involved in bovine botulism. Mosaic type D/C exhibits higher toxicity for cattle than BoNTs C or D. Botulism outbreaks due to type B have also been reported in the Netherlands and the USA, but they are less frequent. BoNT types C and D are often related to contamination of feed by carcasses of poultry, wild birds, or other animals, whereas type B is more associated with improperly stored silage previously contaminated by soils containing C. botulinum type B spores. Oral administration of 22.5 MLD/kg of type D BoNT can induce the disease in cattle. This species is 12.88 times more sensitive to BoNT type C toxin than mice, on a per kilogram basis. An estimated 1 g of BoNT can potentially kill 400,000 adult cows. The cattle intravenous median lethal dose is 0.388 ng per kg. Large numbers of mortalities occur during outbreaks, with rates varying from 8% to 80%. The highest mortalities are seen during the first week of the outbreaks but losses can continue for more than three weeks thereafter. Twelve outbreaks of botulism were reported in dairy and beef cattle between 2008 and 2012 in Italy. In the Netherlands, botulism is estimated to affect about 20 dairy herds each year. Clinical botulism outbreaks were confirmed in 1108 German cattle herds between 1995 and 2010. In the United Kingdom, between 20 and 30 cases per year are reported in cattle. In Sweden, seven cases were diagnosed during the last ten years. At least eight outbreaks were reported between 2004 and 2007 in Japan. Visceral or chronic botulism has been reported in Germany and Denmark. It is allegedly associated with toxico-infection, in which BoNT are produced in situ within the gastrointestinal tract. The existence of this specific clinical form of botulism is controversial. Botulism in horses is mainly attributed to C. botulinum toxin types A, B, and C. In the USA, C. botulinum type B toxin causes more than 85% of equine botulism cases. Type B is endemic in the mid-Atlantic region and Kentucky, whereas type A is endemic in states west of the Mississippi River. C. botulinum type C is uncommon in horses in the USA. On the contrary, types C and D are most common in Europe, except in the Netherlands where type B is encountered more frequently than either type C or type D. Type D is more common in South America and South Africa. The most common form of equine botulism is forage poisoning, an emerging problem in Europe, which is linked to the use of grass silage as feed. Horses are highly sensitive to BoNTs; often, horses are susceptible to less than a single mouse MLD. This leads to a very low rate of laboratory confirmation of diagnosis with the mouse bioassay. Equine grass sickness has been suggested as being associated with C. botulinum type C intoxication, but this association has not been confirmed and the etiology of this disease remains unclear. Fur animal botulism outbreaks are mainly caused by type C toxins, but rare outbreaks caused by types A and E have also been reported. Botulism is considered a major hazard in fur animal production. Mink, foxes, and ferrets are most often affected, and large outbreaks have been reported. For example, in 2002, 52,000 foxes from 83 breeding farms in Finland were affected. Fish are susceptible to C. botulinum type E toxin. The lethal dose for coho salmon is 90 MLD and mortality is observed at 50 MLD for round goby. BoNT E is responsible for visceral toxicosis of catfish (VTC). VTC causes large losses to the catfish industry, up to 37.8% of total loss in some years. Few data are available about the impact of botulism on fish populations or the prevalence of this disease on fish farms. However, type E spores are routinely ingested by healthy fish without any germination. Few reports exist on botulism in dogs, although they are known to be sensitive to the disease. Cats are reported to seem resistant. Only one paper reports type C botulism in a group of cats after being fed pelican carrion. Botulism in pigs is rare and they are also relatively resistant. Botulism has been reported in sheep and goats, with clinical signs similar to those in cattle, mostly in England, Wales, Australia, the USA, and South Africa. Such outbreaks are uncommon in the UK (14 were reported between 1999 and 2007). The source for initial proliferation of group III C. botulinum is typically unknown. However, several routes of contamination have been suggested, including: Many hypotheses about the origin of animal botulism outbreaks are based on the idea that C. botulinum is a normal inhabitant of the intestinal tract of healthy animals. Several studies have been conducted to determine the characteristics of such asymptomatic carriage by animals. C. botulinum has been detected in pig feces in 24% of the examined samples in a German study, 76% in a Japanese study, 62% in a Swedish study, and 3% in a Finnish study. In these studies, BoNT types B, E, and C were identified. Type C was found in the kidney and liver of healthy swine. C. botulinum has also been detected in porcine, equine, bovine, and caprine tonsils. Botulism is rare in pigs, but the data mentioned above indicate that pigs can be reservoirs. Particular attention should be paid to preventing cross-contamination between pigs and poultry and pigs and cattle. Healthy carriage in cattle has also been reported. Prevalence varies substantially from one study to another, including 73% of type B and less than 5% of types E and F in bovine fecal samples in a Swedish study, 13% in cattle feces in a Dutch study, and 0–4% of fecal samples in two German studies, respectively. Most of these studies have focused on the presence of BoNT associated with human botulism outbreaks, and further investigations are needed to evaluate the healthy carriage of group III C. botulinum. The possibility of C. botulinum being part of the normal cattle microflora is a controversial topic in the veterinary literature. Some authors consider that C. botulinum is part of the enteric microflora, while others suggest that the presence of these bacteria in feces of healthy animals is rare. C. botulinum is apparently not part of the normal gastrointestinal flora of the horse. Healthy carriage in birds is also a controversial topic. C. botulinum is reported to be a normal member of the avian gut flora but very few studies have clearly demonstrated its presence in the gastrointestinal tracts of birds. For example, C. botulinum spores have been detected in the gastrointestinal tracts of more than 50% of healthy mallards in a marsh with a history of botulism outbreaks. The organism has also been found in the proventriculus, gizzard, duodenum, and small intestine of unaffected birds during a botulism outbreak in a broiler chicken flock. Two studies have recently been conducted to evaluate such carriage in broilers using real-time PCR. Both studies showed that none of the investigated flocks was positive for C. botulinum types C or C/D. The difficulty in detection of C. botulinum in the avian gut may be because of the low level of bacteria, at least under the detection limit of available methods. In summary, it seems that birds can be healthy carriers of C. botulinum spores (notably after a botulism outbreak) but that normal carriage in broilers is non-existent or extremely low, under the detection limit of available methods. It is likely that an outbreak is preceded by a recent introduction of spores to the flock. The spores may cause disease directly, or may multiply in birds that die of an unrelated cause. Fish can also be healthy carriers of C. botulinum spores. Prevalence of C. botulinum in trout varies from 5–100% in winter and from 80–100% in summer. Tilapia have been implicated as the source of type C BoNT in pelican botulism outbreaks in California. These fish actually harbor C. botulinum capable of producing toxin in their gastrointestinal tracts without producing disease in the fish. They consume large amounts of sediment, which may explain the presence of toxin-producing C. botulinum in their intestines. Spores of C. botulinum type C are widely distributed in marsh sediments. Data about asymptomatic carriage of group III C. botulinum are scarce, especially in cattle and poultry production. New information on this topic will be very helpful in understanding the epidemiology and pathogenesis of animal botulism. Whatever the animal species, botulism is often reported to be seasonal, with an increase in the number of outbreaks during summer and fall, when ambient conditions are near the optimal temperature for bacterial growth. For example, this is typically reported in wild bird outbreaks, for which warm temperatures are also often associated with initiation of botulism. Incidence of botulism outbreaks increases during very hot summers. Occasional winter and spring outbreaks have also been reported in some countries. Water and feed pH are also reported as being risk factors for the germination of C. botulinum spores and BoNT production. Many of the large epizootics of wild bird botulism have occurred in areas of alkaline waters in western North America. The risk of botulism outbreaks has been reported to be increased when water pH is between 7.5 and 9.0, redox potential is negative, and water temperature is above 20 °C. In cattle, forage botulism occurs when pH (> 4.5), moisture, and anaerobic conditions within the forage allow the proliferation of C. botulinum. Animal feed without acidification is amenable to production of C. botulinum spores and toxin production. Silage with a pH above 4.5 is typically considered risky. In poultry, poor biosafety, no acidification or chlorination of water, and a lack of pest control have been reported as risk factors for botulism. The following conditions may also be considered risk factors: The increase in the number of botulism outbreaks in poultry in Europe in 2007 and 2008 was potentially linked to feed. The presence of C. botulinum spores was indeed demonstrated in feed samples collected from four outbreaks on Swedish farms. During a recent European workshop about animal botulism in Europe, feed, rice hulls used as litter, decomposing carcasses, rodents, soil, and hay packaged in plastic were mentioned as potential sources of C. botulinum spores. Foraging behavior seems to be one of the most important risk factors for development of wild bird botulism. Filter feeding and dabbling waterfowl, shorebirds that feed near the surface of wetland soils, and sediment- and fish-eating birds are at greater risk than other waterfowl. Some outbreaks have been associated with landfills. The presence of ideal conditions in landfills for C. botulinum growth (notably rotting organic matter and a rise in temperature due to the composting effect) may then induce spore germination followed by BoNT production. It is also thought that C. botulinum spores are passively transferred from landfills contaminated by spores to other locations. Environmental factors that contribute to initiation of avian botulism outbreaks include low water levels, water-level fluctuations, water quality, and the presence of vertebrate and invertebrate carcasses and rotting vegetation in ponds and other water reservoirs. Type C is highly prevalent in some wetland sediments (60% of samples from a marsh with a prior history of botulism were culture positive, as compared to 6% in lakes with no history of the disease). Type C BoNT and group III C. botulinum strains may be found in invertebrate organisms. The carcass-and-maggot cycle is a well-known scenario through which botulism outbreaks occur in waterfowl when maggots and other invertebrates are consumed by wild aquatic birds. Maggots collected from carcasses of animals dead from botulism carried BoNTs. These highly toxic maggots perform cascades of intoxications when birds consuming maggots develop botulism and die. The carcasses attract flies for egg laying, resulting in an increase of toxic maggots that leads to further botulism outbreaks. Although it has been suggested that BoNT production may occur in dead invertebrate organisms, there is no direct evidence that this occurs. Invertebrates can also carry C. botulinum spores and transfer them from place to place. This has been shown experimentally for necrophagous flies that can transfer C. botulinum spores between two points and excrete them up to 24 hours after exposure, playing a role in botulism outbreaks. Slugs can also excrete spores in feces for up to four days, while the spores persist in slug carcasses for longer periods of time. Slugs may be involved in silage contamination by C. botulinum spores. Recently, chironomids (a type of midget) collected in the Great Lakes region of the US, where avian botulism occurs regularly, were found to be carrying type E strains of C. botulinum. Their contamination may be due to the presence of C. botulinum spores and vegetative cells in cladophora (a type of green alga), which is a food source for larvae of chironomids. Larvae of chironomids are important food sources for fish and for birds, which might be exposed to botulism by eating these larvae. After a botulism outbreak, darkling beetles collected in affected poultry houses were often culture positive for C. botulinum. This suggests that darkling beetles could be reservoirs and carriers of C. botulinum. These beetles feed on bird droppings, bird carcasses, and litter, which could explain why they harbor C. botulinum. Poultry eat darkling beetles and can thus become infected. In conclusion, insects can be carriers of C. botulinum spores and, therefore, insect control is crucial in the management of avian botulism. Botulism outbreaks in fur-producing animals are typically associated with food poisoning. Fur animal feed is mainly composed of poultry, swine, and bovine slaughter by-products, fishery by-products, fur animal cadavers, animal fat, and cereals. The fur animal food chain is highly contaminated by C. botulinum, notably by type C toxin-producing strains, and these animals are widely exposed to these strains. In cattle, type B botulism is typically associated with grass-fed animals, while types C and D cases are more often related to carcass-contaminated feed. The latter are also grown on decomposing plant material. Contamination of feed and water by rotting organic matter containing BoNTs or BoNT-producing clostridia is often incriminated in cattle botulism outbreaks. Type C is usually associated with decomposing carcasses, such as cats or waterfowl. Grain, hay, or silage can be contaminated by rooting organic matter during harvesting or storage. Silage that is not properly stored (allowed to rot, for example) provides ideal conditions for C. botulinum growth (moisture, high temperature, anaerobiosis). The pH of silage has to be less than 4.5 to avoid growth of C. botulinum spores. Use of mixing and distributing feeders increases the risk of dissemination of contaminated feed to all animals, even if only a small quantity of feed is contaminated. Types D and C bovine botulism have also often been associated with poultry litter used as feed or bedding. The practice is no longer allowed in the USA, but the recent emergence of bovine botulism in England, Wales, and Northern Ireland has been associated with broiler litter. In Northern Ireland, for instance, most cases have been linked to the spreading of broiler litter on pastures, and 85% of outbreaks have occurred when cattle were at pasture. Equipment such as trailers or tractor buckets used for removing litter from broiler houses could play a role in the transfer of spores from poultry production to cattle production. Botulism can also occur as a result of pica in cattle. This behavior originates when phosphorus and/or calcium-deficient cattle develop a depraved appetite, gnawing on bone to compensate for those deficiencies. This has been notably described in Australia, South Africa, Brazil, and Argentina. Molecular epidemiological investigations of C. botulinum strains allow better characterization of the organism at the genetic level. There is little information in this regard about group III strains. This could be explained by the difficulty of isolating group III C. botulinum strains. There is actually no selective medium, and group III C. botulinum strains are known to grow poorly in mixed culture and on agar plates. The instability of the phage carrying the BoNT gene in laboratory conditions is also problematic, and this phage is easily lost during isolation procedures. One complete genome and twelve draft genomes from avian strains are available. Analysis of these genomes showed that the chromosome is highly conserved and that genetic diversity is observed in the plasmidosome of the isolates. This minor genetic diversity among the chromosomes has also been described by comparison of the group III isolates via classical molecular techniques. New typing methods should be designed to compare group III strains and perform epidemiological investigations when needed. A PCR-based method should be developed to compare strains while avoiding the need for isolation. Pulsed-field gel electrophoresis (PFGE), randomly amplified polymorphic DNA (RAPD), and amplified fragment length polymorphism (AFLP) have been used to compare group III C. botulinum strains. Type D-C strains isolated from the bovine gastrointestinal tract in Japan were compared by PFGE after digestion with SacII. This revealed that at least two strains of different origin were involved in bovine botulism outbreaks in Japan. On the other hand, studies revealed an apparently common pulsotype involved in avian botulism in Sweden and in Spain. RAPD analysis is a fast and simple PCR-based DNA fingerprinting method, but with the major drawback of poor reproducibility due to non-specific primer binding. This technique was used to compare avian isolates, and results match those obtained by PFGE analysis. AFLP is a useful technique in epidemiological investigations. This has been tested in a study comparing all C. botulinum groups and strains isolated from a type C bovine botulism outbreak. Using this method, C. botulinum strains clustered according to toxin type, source, and, for some profiles, to geographic location. Molecular epidemiological studies regarding group III C. botulinum are, thus, scarce, and further investigations are needed to provide efficient tools that will allow a better understanding of animal botulism epidemiology.
Botulism
Introduction
Etiology
Pathogenesis
Occurrence and prevalence of animal botulism
Animal species
BoNT type
Occurence
Fur animals (mink, fox)
C, C/D
A, E
++++
+
Cattle
D, D/C
C/D, C
A, B
++++
++
+
Birds
C, C/D
E, D
A
++++
++
+
Equine
C, D
B
A
+++
+
+
Fish
E
?
Birds
Cattle
Horses
Fur animals
Fish
Other animal species
Epidemiology
Source of contamination
Healthy carriage
Environmental conditions favorable for botulism outbreaks
Temperature
Water and feed pH
Risk factors associated with avian botulism
Risk factors in poultry production
Risk factors in wild birds
Role of invertebrates in avian botulism
Risk factors associated with fur botulism
Risk factors associated with bovine botulism
Molecular epidemiology
Clinical signs
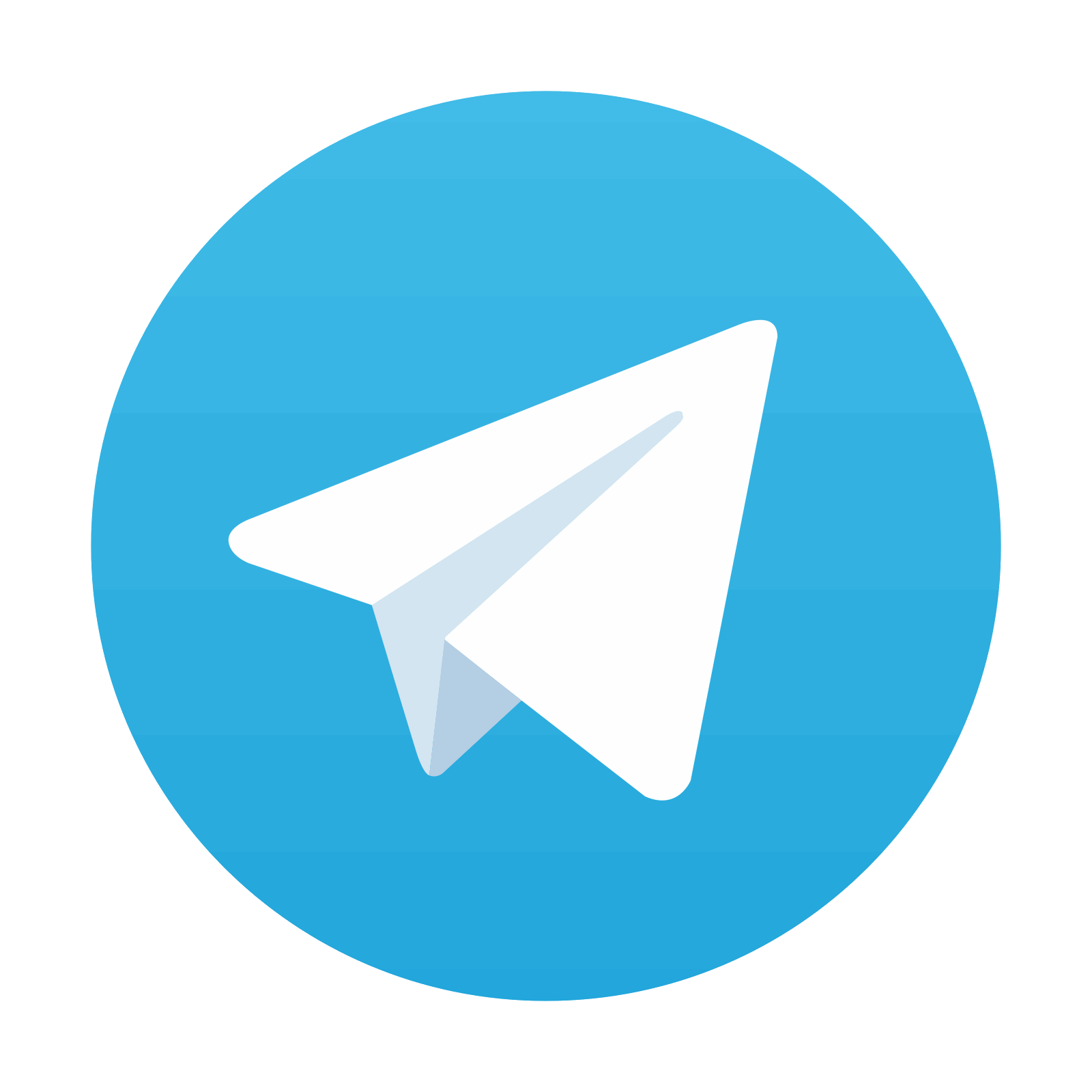
Stay updated, free articles. Join our Telegram channel

Full access? Get Clinical Tree
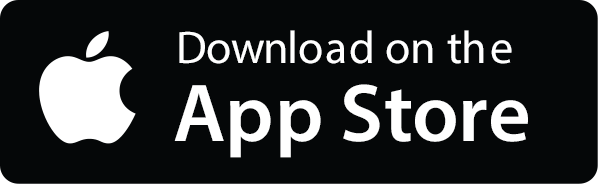
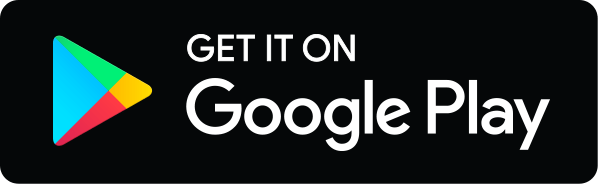