Disease or infectious agent
Presence in ovary, oviduct or uterus
Association with washed embryos
Transmission by ET
Transmission by semen
Investigated species (source/reference-as superscript)
Potential wildlife counterparts
Viral
SVSV3
−
+
?
+
a, bSus scrofa
Suidae
PRRS3
+
−
−
+
e, n, sSus scrofa
Suidae
PrV
+
+
±
±
a, bSus scrofa
Suidae
PCV4
+
+
−
+
tSus scrofa
Suidae
HCV2
+
+
−
±
a, bSus scrofa
Suidae
ASFV4
−
+
?
+
a, bSus scrofa
Suidae
PPV4
+
+
±
a, bSus scrofa
Suidae
FMDV3
−
+
+
a, bSus scrofa
Suidae
FMDV1
FMDV3
FMDV3
+
−
−
−
−
−
−
−
−
+
+
+
a, bBos Taurus
hOvis aries
hCapra hircus
Bovidae, Cervidae, Camelide
Maedi-Visna3
+
−
−
?
rOvis aries
Unknown
Maedi-Visna
−
+
−
?
hCapra hircus
Unknown
BVDV CP3
+
−
−
+
a, bBos Taurus
Some ruminants
BVDV NCP3
+
+
−
+
oBos Taurus
RPV3
−
+
−
±
a, bBos Taurus
Bovidae; Cervidae
PI-3V4
−
+
?
?
a, bBos Taurus
Various spp.
BIV3
−
_
−
+
iBos Taurus
Unknown
BHV1
+
+
−
+
a, bBos Taurus
Bovidae
AKV4
−
−
?
+
a, bBos Taurus
Some ruminants
BLV1
−
−
−
−
a, bBos Taurus
Unknown
VSV4
+
−
±
a, bBos Taurus
BTV1
BTV-84
BTV2
+
−
?
?
−
−
?
−
+
+
a, bBos Taurus
hCapra hircus
hOvis aries
Bovidae; Cervidae
FIV
?
?
?
+
cFelis catus
Felidae
CAEVC2
+
−
−
−
hCapra hircus
Small ruminants
EAV
+
±
±
+
pEquus ferus caballus
Equidae
TSEs
BSE1
−
−
−
−
mBos Taurus
Bovidae; Felidae
CVD
?
?
?
−
mOdocoileus sp.
Cervidae
Scrapie2 (typical)4
?
?
−
−
−
−
mOvis aries
mCapra hircus
Unknown species
Bacterial
M. bovis4
−
−
?
+
fBos Taurus
Bovidae
B. abortus1
B. ovis
−
−
−
−
−
?
+
±
a, bBos Taurus
a, bOvis aries
Bovidae; Cervidae;
Leptospirosis4
+
+
?
?
+
+
a, dBos Taurus
aSus scrofa
Bovidae; Suidae
Yohnes disease3
+
+
−
±
a, lBos Taurus
Bovidae; Cervidae
Mycoplasmas4
+
?
+
a, b, gBos Taurus
All mammals
Vibriosis
+
?
+
aBos Taurus
Bovidae
Parasitic
Trichomoniasis4
+
+
?
+
a, iBos Taurus
Unknown
Piroplasmosis
−
?
±
?
aBos Taurus
Bovidae
Neosporosis3
−
+
−
?
a, jBos Taurus
Bovidae; Cervidae
Felidae; Canidae
For background on basic cryobiology, germplasm and ovarian tissue cryopreservation, readers are referred to the publication by Karrow and Critser (1997) and the more recent articles on new methods of vitrification by Vajta and Nagy (2006), Tucker and Liebermann (2007), Yavin and Arav (2007), Kuwayama (2007), Mazur et al. (2008), Mazur and Seki (2011), Saragusty and Arav (2011), Seli and Agarwal (2011), Zhang et al. (2011), Arav and Natan (2012) and Liu et al. (2012). Comparative cryobiological requirements for germplasm and ovarian tissue of wildlife and threatened species were reviewed by Leibo and Songsasen (2002), Santos et al. (2010) and Comizzoli et al. (2012). Factors to be considered in developing a genome resources bank (GRB) for a taxon/species also were identified by Wild (1997), Woelders et al. (2012), Agca (2012) and Mara et al. (2013). Lastly, managing the potential for cross contamination of germplasm during cryobanking in the liquid (LN) and vapour phases of nitrogen (VPLN) was previously discussed by several authors (Rall 2003; Bielanski and Vajta 2009; Pomeroy et al. 2010; Criado 2012).
2 The Origin of Microbial Contamination in Germplasm and Somatic Cells Prior to Cryopreservation
2.1 Semen
It has been recognized that systemic and local infections of the reproductive tract, as well as the inadvertent introduction of microorganisms during processing, may potentially contribute to the contamination of semen. In general terms, microorganisms can already be present in the semen of an infected male when it is ejaculated or they can gain entry during collection, processing or storage. Spermatozoa can become infected by a microorganism in the testes or during their transit through the epididymis, ductus deferens and urethra. Other occasions when microorganisms can be present in semen are when they are associated with blood cells or there is inflammation or trauma of the accessory glands (prostate, seminal vesicle or bulbourethral gland). Furthermore, there are some microorganisms that can contaminate semen due to their high concentration in urine or in the preputial cavity.
In addition, some potential contaminants (e.g. mycoplasmas) may be introduced into semen with animal derived supplements used in diluents and extenders (egg yolk, milk). Environmental microbes may also contribute to semen contamination or a result of poor laboratory hygiene (Schiewe and Hasler 2010; Wrathall et al. 2007).
Frequently, ejaculated semen is not free from bacterial flora. The saprophytic bacteria of the prepuce in a healthy male comprise numerous species that may become associated with the semen. Some of these bacteria may behave as opportunistic pathogens (e.g. Pseudomonas aeruginosa) and may be a potential risk to the inseminated female. For example, the most common potentially pathogenic microorganisms isolated from bull semen are P. aeruginosa. Streptococcus spp., Staphylococcus spp., Proteus spp., and Bacillus spp. (Wierzbowski 1985). The notion that spermatozoa could function to transport surface-bound bacteria has been reported for Chlamydia trachomatis, Chlamydia psittaci, Escherichia coli, Neisseria gonorrhoea, Veillonella parvula, Peptostreptococcus spp., Ureaplasma urealyticum, Mycoplasma spp., and Candida albicans (Toth et al. 1982).
A number of viral pathogens have also been identified in association with the semen of infected animals and humans (Hare 1985; Dejucq-Rainsford and Jegou 2004; Bielanski 2006; Zea-Mazo et al. 2010). Some of the viruses can adhere to the surface of spermatozoa and others are associated with the seminal plasma or non-sperm cells present in the semen. Several reports, some of which are controversial, have suggested an ability of some viruses to penetrate the sperm head and integrate their nuclei acid into the sperm genome. These viruses include, Simian virus 40 (Brackett et al. 1971), bluetongue virus (Foster et al. 1980), bovine herpesvirus-1 (BHV-1) (Elazhary et al. 1980), human hepatitis B virus (Hadchouel et al. 1985), Rous sarcoma virus (Habrova et al. 1996), herpes simplex 1 and 2 (Kotronias and Kapranos 1998), murine cytomegalovirus (Magnano et al. 1998), human immunodeficiency virus-1 (HIV-1) (Piomboni and Baccetti 2000), and human papilloma virus (Rintala et al. 2005). For this reason, the complete elimination of these viral agents from semen and sperm cells may be difficult or even not possible to achieve.
2.2 Embryos
Prior to ovulation oocytes may become infected by contact with an infectious agent present in either the ovarian granulosa cells or the follicular fluid, probably during viremia at the acute stage of a disease (Van Soom et al. 2010a). At this stage viruses may be present in the blood and other body fluids and spread to various tissues and organs (Table 17.1). For example, in cattle, microorganisms have been found in follicular fluid a few days after natural and experimental exposure to bovine viral diarrhea virus (BVDV) and BHV-1 (Bielanski and Dubuc 1994; Bielanski et al. 1998a). This indicates that the collection of oocytes for in vitro fertilization (IVF) at this stage of the disease may result in embryos contaminated with the pathogenic agent. This hazard may be substantial when ovaries are harvested from asymptomatic persistently or latently infected donors (e.g. BVDV, BHV-1). Post-ovulation, oocytes may become infected by a spermatozoon during fertilization, or by contact with a pathogen that has been excreted into the oviduct or uterus (Booth et al. 1995). Other sources of contamination include agents introduced with culture supplements of biological origin such as serum, trypsin, supporting co-culture cells or cell lines for nuclear transfers (Van Os et al. 1991; Brock 1998; Shin et al. 2000; Drew et al. 2002; Schiewe and Hasler 2010; Nikfarjam and Farzaneh 2012). Environmental microbes associated with an operator, abattoir origin or the laboratory may pose risks during the production of embryos in vitro when pooled materials are used. In this regard, inadvertent inclusion of the follicular fluid from an infected animal into the pool may pose a risk of the cross-contamination of all clean oocytes and consequently lead to batches of contaminated embryos (Bielanski and al. 1993; Bielanski and Stewart 1996; Marquant-Leguienne et al. 2000). Also transvaginally collected oocytes are potential sources of microbial contamination of the in vitro fertilization (IVF) and embryo transfer (ET) culture systems. Cottell et al. (1996) reported that various microorganism (Mycoplasma hominis, Ureaplasma urealyticum, Staphylococcus epidermis, Lactobacilli sp., Difteroids) were isolated from approximately one-third of the needle flushes after oocyte recovery and from more than one-third of the follicular fluids aspirated from the first follicle punctures on each ovary.
In general, there are two periods prior to gamete retrieval that affect success of gamete preservation and their sanitary status. The first is the interval from animal’s death to necropsy and the second is from gonad retrieval to gamete recovery in laboratories (Chatdarong 2011). It can be expected that harvesting somatic tissues and germplasm from wildlife animals in their natural habitat may occur hours or days after their death. It is well known that gastro-intestinal bacteria are capable of migrating from the gut to any other region of the body by using the lymphatic system and blood vessels as early as 5 min after death (Melvin et al. 1984; Heimesaat et al. 2012). How rapidly microbial invasion reaches the reproductive tract, and particularly testes and ovaries, have been not established. It is predictable however, that in climatic regions with high environmental temperatures and humidity, the process of carcass decomposition will be accelerated. Nevertheless, it appeared that sperm cells harvested after death may remain viable longer than oocytes (Johnston et al. 1991; Stoops et al. 2011).
2.3 Tissues for Somatic Cells Nuclear Transfer (SCNT)
In recent years SCNT procedures have become an important tool for regeneration of valuable genetically species as part of the assisted reproductive technologies (ART) (Holt et al. 2004; Andrabi and Maxwell 2007; Galli and Lazzari 2008). There are three main potential routes for the introduction of pathogens during cloning embryos prior to cryopreservation. These include contaminated donor somatic cells, oocytes and culture system used for reconstructed embryos. Another important source of contamination can be laboratory environment and personnel, who handle cell cultures. Most frequently mycoplasma and other bacteria from human skin, airborne fungal spores and saprophytic microorganisms are isolated from cell cultures (Cobo and Concha 2007).
A variety of somatic cells such as mammary epithelium, adult and fetal fibroblast, granulosa, cumulus cells and other, have been used to produce animal clones (Rodriguez-Osorio et al. 2012; Men et al. 2012). More recently, induced pluripotent stem (iPS) cells derived from somatic cells opened a potential source of reprogrammed donor cells for nuclear transfer and gene banking of domestic and endangered species (Malaver-Ortega et al. 2012; Verma et al. 2012).
Cells for SCNT are usually obtained either from the existing established cell lines or from live animals with desirable phenotypes. When somatic cells are harvested, health status of donors should be taken into consideration, since microorganisms can be present in blood and many tissues in acutely and persistently infected animals (Fields 2006). For example, persistently BVDV-infected cattle represent a special hazard since they constitute reservoirs of infectious virus in serum, ovarian follicular fluid, gametes, and somatic cells as well as fetal tissues and serum (Brownlie 1990). Stringfellow et al. (2005) reported that 5 of 39 fetal fibroblast cell lines used for cloning research were positive for BVDV as determined by the reverse transcription-polymerase chain reaction (RT-PCR) assay. The risk and consequences of introducing BVDV by infected somatic cells in nuclear transfer was demonstrated by Shin et al. (2000). At days 40–55 all cloned fetuses produced from BVDV-infected fetal fibroblast cell lines were positive. In addition, pregnancy loss was significantly greater in fetuses derived from BVDV-infected cell lines as compared to non-infected cell lines.
It is relevant to mention that there is a theoretical risk of reactivation of endogenous retroviruses (ERV) (dormant viruses) via introduction of a foreign nucleus into an enucleated oocyte. However, to date there have not been any reports of such outcomes occurring during the cloning process (Thibier 2001; Kochhar and Rudenko 2010). Experimentation with bovine clones revealed that ERV sequences were not transcribed and no RNA was detected in the blood of clones, donor animals or controls (Heyman et al. 2007; Anon 2008a).
Health risks associated with the procedure of oocytes recovery from live animals using ultrasonography or from abattoir materials and their handling are of equal importance to those encountered during IVF (Bielanski 2010). Oocytes for SCNT recovered from follicular fluid pools of slaughtered animals with unknown health status may be a potential source of infectious agents for cloned embryos. Some of those agents [e.g. caprine arthritis encephalitis virus (CAEV), BVDV, BHV-1] may adhere to the ZP of oocytes and may also have ability to replicate in oocyte cumulus cells (OCC) (Vanroose 1999; Stringfellow et al. 2000; Ali Al Ahmad et al. 2005). Therefore, it is of paramount importance to remove OCC and wash oocytes prior to puncturing the ZP and transfer a somatic cell into an enucleated oocyte. Nevertheless, the risk of transmission of some viral agents such as lentiviruses [e.g. HIV, bovine immunodeficiency virus (BIV), feline immunodeficiency virus (FIV), and equine infectious anemia virus (EIAV)] by SCNT seems to be unlikely, since oocytes and early embryonic cells are not susceptible to infection with those agents (Gregg and Polejaeva 2009).
To reduce the risk of producing infected cloned embryos, using defined media free of biologically derived supplements [e.g. fetal bovine serum (FBS), porcine trypsin] for culture of somatic cell lines and reconstructed embryos is advisable (Givens et al. 2004; Bielanski 2007; Wang et al. 2012; Schiewe and Hasler 2010). A major concern is the introduction of BVDV to culture system through FBS. Other most common viral contaminants in FBS are BHV-1, and parainfluenza virus-3 (Ericson et al. 1991). Standards for quality testing of FBS have been defined in WHO Technical Report Series No. 673 (Anon, WHO 1982). Use of bovine serum albumin (BSA) may reduce considerably the risk of contamination due to the way that BSA is manufactured (Schiewe and Hasler 2010).
While there are steps in the SCNT technique which differ from the in vitro fertilisation procedure, no specific health risks related to oocyte enucleation, the fusion of oocyte with a somatic cell nucleus or the injection of the somatic cell nucleus directly into the cytoplasm of the enucleated oocyte have been reported.
The animal health risk associated with SCNT cloning technology has been addressed in the Terrestrial Animal Health Code (OIE, Chapter 4.11.) and by European Food Safety Authority (Anon 2008a, 2011). Both publications provide a scientific basis for recommendations on health and welfare of animals involved in SCNT cloning with other assisted reproductive technologies. In general, the guidelines for sanitary precautions of collection of oocytes and processing of reconstructed embryos are similar to those recommended by IETS with regard to the production in vivo and in vitro fertilized embryos (Stringfellow and Givens 2010). In particular, the importance of sanitary precautions in the following contexts is highlighted:
1.
During oocyte collections—to minimize blood contamination, to test each pooled batch of follicular fluid for the presence of infectious agents, to use pathogen-free serum and protein components, and proper antibiotics in culture media to control bacterial microbes.
2.
During donor cell processing—to harvest cells under proper sanitary conditions, to test master cell lines for the presence of bacteria, fungi, mycoplasmas and viruses.
3.
During cloning—if a co-culture system (e.g. oviductal cells) is used to culture reconstructed embryos, proper screening of the cells should be performed. A sample of cells should be tested for bacterial, fungal, mycoplasmal or viral components (e.g. BVDV). Proper washing and cryopreservation of the reconstructed embryos should be followed as recommended by IETS (Stringfellow and Givens 2010).
Although the above listed sanitary recommendations were put together for processing somatic cells and embryos of livestock, it appears they also may find practical application in cloning procedures for wildlife species.
Other parts of publications deal with issues of animal health risk related to surrogate dams, born clones and their offspring.
So far the risk for disease transmission by SCNT has been determined only for a few pathogens of livestock animals. It was concluded that if strict sanitary precautions, as recommended by OIE and IETS, are followed, the hazard of EIAV (and probably other lentiviruses), porcine reproductive and respiratory syndrome virus (PRRSV), and BVDV would be small or absent (Gregg and Polejaeva 2009; Gregg et al. 2010a, b, 2011; Asseged et al. 2012). The risk of transmission of other infectious agents by SCNT, which may affect health status of clones of both livestock and wildlife species, remains to be investigated.
3 Fundamental Methods of Rendering Gametes Free of Pathogens Prior to Cryopreservation
3.1 Washing Procedure for Embryos
Procedures and requirements for washing of ova/embryos are outlined in detail in the Manual of the IETS (Stringfellow 2010). Zona pellucida-intact embryos free of adherent material are transferred through 5 washes of phosphate–buffered saline (PBS), containing antibiotics and 0.4 % bovine serum albumin (BSA), and then through 2 aliquots of 0.25 % sterile porcine-origin trypsin for a total 60–90 s. Next, embryos are transferred through an additional 5 sterile washes of PBS containing antibiotics and 0.4 % BSA or 2 % FBS (pathogens free). Where washing did not remove an agent from the ZP, enzymes have been used to inactivate the agent or loosen its attachment to the ZP (e.g. herpes viruses) (Bielanski 2007).
The embryos should be washed with at least 100-fold dilution between each wash, and a fresh pipette should be used for transferring the embryos through each wash. An example of embryo washing efficiency on reduction of pathogenic agents associated with ZP is illustrated in Fig. 17.1.
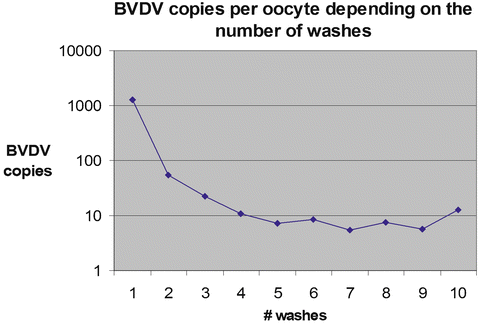
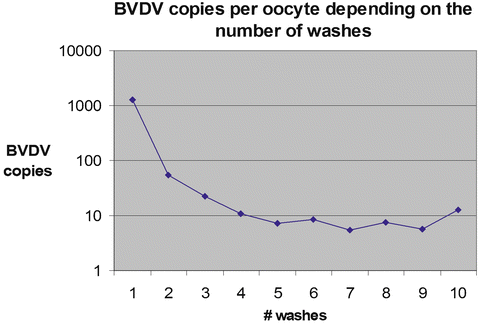
Fig. 17.1
Effect of sequential washing of contaminated oocytes with bovine viral diarrhea virus (BVDV) on a number of viral copies remained in association with a single oocyte (based on data Lalonde and Bielanski 2011)
3.2 Washing Procedures for Semen
When raw semen is diluted and extended for commercial use, the number of potential micro-organisms per unit volume can be practically decreased to below the minimum infective artificial insemination (AI) dose (Hare 1985). In contrast to AI, for IVF only motile sperm has been used, which requires application of further antimicrobial procedures to eliminate the potential for disease transmission via semen (Bielanski 2007).
Over the years different forms of washing have been developed for both human and animal spermatozoa, and, as with embryos, washing has become the most important procedure for the control and elimination of microorganisms in ART clinics.
Although the transmission of infectious agents to embryos by contaminated semen was only proven experimentally (BHV and BVDV), such potential should not be overlooked when cryopreserved semen is used for the generation of embryos using superovulation and AI or IVF (Wrathall et al. 2006; Bielanski et al. 2008, 2009). At present, to reduce such a risk, it is commonly the practice to use a discontinuous gradient centrifugation and/or swim up procedures to remove or reduce the load of various viral and bacterial agents from frozen-thawed semen prior to fertilization. It has been shown that this procedure diminishes the risk of disease transmission to recipients and contamination of in vitro fertilization systems, e.g. HIV, HCV, porcine reproductive and respiratory syndrome virus (PRRSV), equine arteritis virus (EAV) (Maertens et al. 2004; Morfeld et al. 2005; Loskutoff et al. 2005; Morrell and Geraghty 2006). It appears that these procedures may be less effective in removing pathogens from animal semen than from human semen because patients can be treated with antiviral agents before ART (Bielanski 2007).
3.3 Disinfection Procedures for Semen and Embryos
Besides trypsin treatment, a variety of other antimicrobial procedures (e.g. antiviral agents, photosensitive dyes, immunological methods) are currently available for disinfecting semen and embryos, but some of them are still at an experimental stage of development. However, none of them seems to fulfil the requirement for a universal disinfectant (for review see Bielanski 2007).
In addition to the IETS sanitary protocol and OIE health codes for collection and processing of semen and embryos, general sanitary recommendations for cryoconservation of animal genetic resources have been summarized in FAO guidelines (Anon 2010).
4 Factors Facilitating Contamination of Gametes During Cryopreservation
There are several critical factors which may influence the contamination of embryos with pathogens during cryopreservation and some of them are also applicable to semen processing. These include the integrity of the embryonic ZP, the cooling method, loading and sealing of the freezing container and the sterility of the LN and the Dewar storage container.
4.1 Integrity of ZP
Unfertilized oocytes, as well as oviductal and uterine-stage embryos, up to approximately day 8 after fertilization, are surrounded by an acellular, glycoprotein shell with a sponge-like surface, the ZP (Herrler and Beier 2000). The specific structural and chemical nature of the ZP is a major factor with regard to interaction with pathogenic microorganisms and its role in disease transmission (Van Soom et al. 2010b).
It is known that the intact ZP of uterine stage and IVF embryos is an effective barrier against penetration by most pathogens even though some may adhere firmly to the surface (Fig. 17.2). The ZP protects the entire surface of the embryo until more advanced blastocyst (expanded) stages. Soon after, the ZP surface is stretched to such a degree that microvilli of the embryonic trophectoderm project through gaps in ZP and thus enable direct contact to occur between the embryonic cells and potential microorganisms (Fig. 17.3). New IVF related ART procedures such as ICSI, embryo sexing, embryo cloning and gene transfer involve cracking and puncturing the ZP or removing the entire ZP from embryos to permit manipulation of the embryonic cells. This is often followed by an extended period of in vitro culture. These procedures increase the risk of exposure of embryonic cells to pathogens and their contamination.
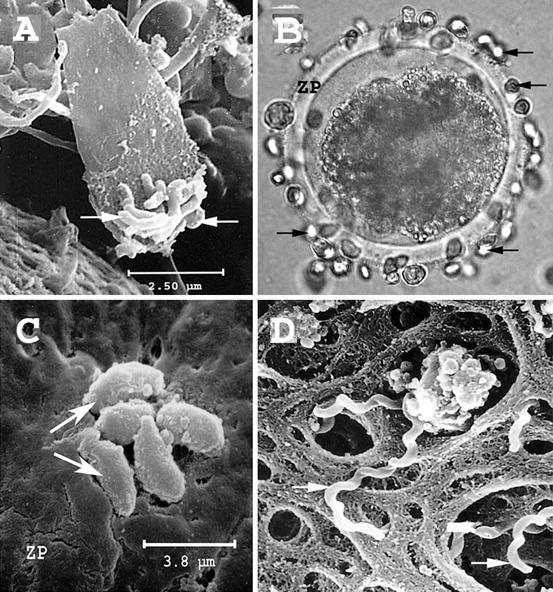
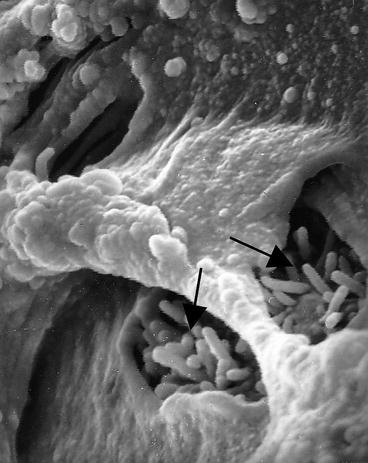
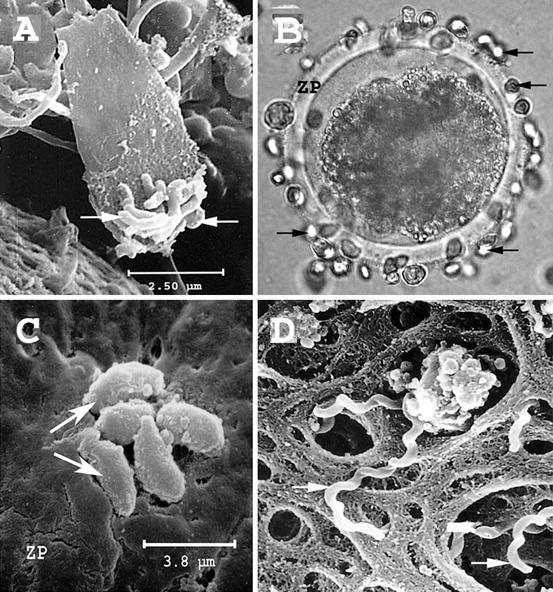
Fig. 17.2
Scanning electron microscopy (SEM) figure of Campylobacter fetus (arrows) attached to the acrosomal region of a sperm cell on the zona pellucida (ZP) of bovine embryo (a); Tritrichomonas foetus (light micrograph) (b); Neospora caninum (c); and Leptospira borgpetersenii serovar hardjobovis (d) (arrows) on the surface of ZP (from A. Bielanski collection)
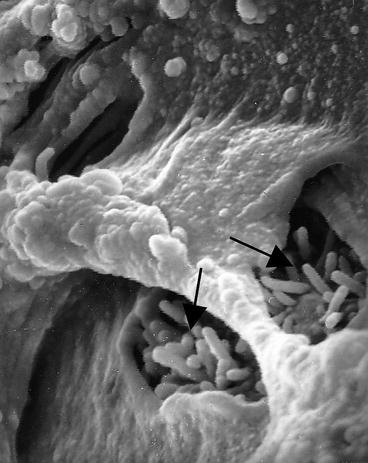
Fig. 17.3
Bovine expanded blastocyst showing gaps in the zona pellucida penetrated by trophectoderm microvilli (arrows) (from A. Bielanski collection)
Maintenance of the intact ZP throughout freezing and post-thaw manipulation is crucial for the prevention of embryonic cell contamination and infection. Under certain freezing and thawing conditions, more than 50 % of mammalian embryos may have ZP fractures (Bielanski et al. 1986; Rall and Meyer 1989). These conditions depend on the speed of cooling and warming, the type of freezing container and the cryoprotectant used (Schiewe et al. 1991). In addition to the risk of embryonic cell contamination, damage to the ZP may have a negative influence on further embryonic development. The short exposure of straws in LN vapour (−150 °C) before plunging into LN may reduce fracture of the ZP. Similarly, during warming, the exposure of straws for a few seconds in air before immersion into warm water may have a beneficial effect (Kasai et al. 1996). More damage to the ZP was observed when low cooling rates and glass containers were used than fast cooling by vitrification and 0.25 ml straws (Schiewe et al. 1991). A small sample volume and adjusted post-freeze warming temperature parameters may protect the ZP against fractures. It appears that vitrification procedures cause less damage to the ZP as compared to standard, slow cooling methods (Schiewe et al. 1991; Kasai et al. 1996). Thus, from a practical viewpoint, the choice of a method of freezing-thawing which ensures maximum embryonic survival and minimum ZP damage should be considered, especially when the embryos are produced for international movement.
It has been shown that in vitro culture and cryopreservation processes may cause alterations in the structure of the ZP (Moreira da Silva and Metelo 2005). It was observed that the number and the diameter of the pores in the ZP decrease due to slow cooling and it is even more reduced after vitrification. Whether it has any effect on microbial adherence to the ZP or their protection from penetration is unknown. Nevertheless, it is conceivable that before cryopreservation, rapid changes in osmotic pressure due to the high concentration of cryoprotectant may cause the passage of a viral agent through an intact- ZP. However, it was shown that the exposure of embryos to BVDV (60 μm) in a 30 % suspension of either DMSO, ethylene glycol, glycerol, or 2 M sucrose for 10 min did not cause the contamination of embryonic cells (Bielanski et al. 1999a). Whether this observation is valid for other smaller viral agents, e.g. foot-and-mouth disease virus (FMDV, 24 μm) or porcine circo virus (PCV, 27 μm) and various mixtures of vitrification solutions remain to be investigated. Further modification in ZP structure during cryopreservation might be influenced by a high concentration of cryoprotectants used for vitrification of oocytes. This may induce hardening of ZP and prevent its penetration by sperm required for fertilization (Vincent et al. 1990). Whether these alterations in ZP surface influence an interaction with microorganisms during cryopreservation is unknown.
It should also be noted that studies on pathogen interaction with ZP-intact and ZP-free camelid embryos (e.g. Dromedary Camels, Llamas, Alpacas, Guanacos, and Vicunas) have not been carried out. Since camelid embryos have already hatched from the ZP prior to entering the uterus (Picha et al. 2013), it would be unrealistic to stipulate that such embryos can be used for cryopreservation without any risk of contamination. Similarly, little is known about transmission of pathogens by cryopreserved embryos of some species of perissodactyla, marsupialia and lagomorpha, which are enclosed in capsule, shell membrane or mucin coats respectively (Selwood 2000; Herrler and Beier 2000; Stout et al. 2005).
4.2 Cooling Methods
Comparatively, cryopreservation by a modified slow cooling method of farm animals embryos as described by Willadsen (1977) and by vitrification originally described by Rall and Fahy (1985), as well as by Stachecki et al. (2008), offers a relatively lower risk of contamination of samples during cooling due to lack of direct contact with potentially infected LN, than the recently invented, so called “open system vitrification”. In both preceding methods, embryos in hermetically sealed vials or straws are exposed to vapours of LN before their plunge in LN for storage. Efficiency of sealing and quality of the freezing containers as well as their resistance to cracking at low temperatures will determine the risk of contamination of embryos during their banking.
During application of vitrification using “open system” methodology, embryos at first can be cooled in a small volume of “clean” LN, followed by placing the original sample into a secondary sealed container before plunging into LN for storage [e.g. open pulled straws (OPS) (Vajta et al. 1997), cut standard 0.25 ml straws (Isachenko et al. 2007), Cryotops® (Kitazato BioPharma Co., Japan) (Kuwayama 2007), Cryoloops (Lane et al. 1999), hemi-straw system (Vanderzwalmen et al. 2003) and a plastic blade (Sugiyama et al. 2010)]. As an example, the risk of contaminating embryos vitrified in OPS using the commercial kit Vit-Set™ (Minitube Canada, Ingersoll, Ontario, Canada) was tested under experimental conditions (Bielanski and Hanniman 2007). The Vit Set™ consists of three stainless steel chambers for cooling the 0.5 ml protective straws, vitrification of OPS straws, and for loading OPS into the protective straws respectively. Bovine embryos were vitrified and protected against contamination as described originally by Vajta et al. (1998). Briefly, when embryos contaminated with cultures of Escherichia coli (E. coli), Pseudomonas aeruginosa or non-cytopathic New York (NY) strain of BVDV, were vitrified in OPS straws and then protected by 0.5 ml Cryo Bio System (CBS™) straws, no cross-contamination to clean embryos or LN was detected. It was concluded that the potential for cross contamination of samples by application of the Vit-Set™ for vitrification of embryos using OPS is negligible if: (1) LN in the chambers is frequently replaced and the chambers are disinfected between embryo donors, (2) the protective straws are applied over OPS and are hermetically sealed. The straws should be thermally sealed and used without a cotton plug.
More recently, many researchers, recognizing the strict sanitary requirements for the generation of cryopreserved oocytes/embryos free of infectious agents, have developed entirely “closed systems” of vitrification without the necessity of exposing embryos or semen to LN (Kuleshova and Shaw 2000; Isachenko et al. 2005a; Kuwayama et al. 2005; Hirayama et al. 2007; Schiewe 2010; Larman and Gardner 2011; Criado et al. 2011). Using such a system, embryos are positioned on Cryoloops, Cryotips® (Irvine Sci.) into OPS, CBS™ straws, microcapillary tubes or on a stainless steel needle chip and then into pre-cooled cryovials or 0.25 ml plastic straws (“straw in straw”) to accommodate the sample during vitrification by super-cool air inside the container before immersion in LN. Similar techniques have been reported earlier for the freezing of sperm, embryos and ovarian tissue in small drops on supercooled aluminium foil or steel blocks and cubes (solid surface vitrification); Cryologic vitrification method™ (Cryologic; Mulgrave, Australia) before placement into a protective container and immersion in LN (Dinnyes et al. 2000; Lindemans et al. 2004; Isachenko et al. 2005b; Aerts et al. 2008). Furthermore, Yavin et al. (2009) applied liquid nitrogen slush for vitrification of murine embryos in sealed pulled straws (SPS), to facilitate a high cooling rate and reduced toxicity of cryoprotectants.
Presently, there are cryo-sets allowing avoidance of contact with LN available on the market, which include LN containers, straws, sealers and reagents required for vitrification of embryos [e.g. Vitrolife Rapid-I™ vitrification system, Vitrolife, Goteborg, Sweden; Cryologic CVM Kit™; OPS, RVT, Cairns, Australia; Ultravit, Criado, Spain; CBS™ High Security Straws (IMV, France)]. At this point it is relevant to note that some of the “closed” vitrification systems, which require exposure of embryos or protective containers to LN vapours, may pose some risk of sample contamination when LN is not sterile (Parmegiani and Vajta 2011). Above described methods of cryopreservation, however, involve troublesome LN handling and its danger for contamination of specimens. On the contrary, Faszer et al. (2006) and Morris et al. (2006) described the application of a liquid nitrogen-free Stirling Cycle Cryocooler (EF 600, Asymptote Ltd, Cambridge, UK) for the cryopreservation of mouse and stallion spermatozoa and human embryonic stem cells by slow cooling rates. Survival rates of all cell types frozen in the Stirling Cycle freezer were similar to samples frozen in the LN freezers. The freezer works based on the expansion and compression of helium in a sealed cylinder and can be used for the freezing of large volume samples (e.g. up to 15 ml semen bags) without the risk of contamination. A similar programmable LN-free freezer, the “Pulse Tube” cryocooler with a special low vibration engine, is under development at the “Sapienza” University of Rome (Lopez et al. 2012).
Whether cooling/thawing rates and the cryoprotectant used are relevant to the survival of microorganisms associated with embryos was investigated (Bielanski and Lalonde 2009). It was found that both methods, namely cryopreservation by slow cooling and vitrification, significantly reduced titers of BVDV and BHV-1, but did not however, render embryos free from infectious viruses after thawing.
In general, it can be assumed that procedures which involve the step-wise dilution of the cryoprotectant provide an opportunity to inspect the ZP for fractures and wash the potential pathogens from the embryos. Thus, the one-step dilution of cryoprotectants in straws followed by direct ET should be considered as more hazardous from the sanitary point of view.
4.3 Freezing Containers
In general, regardless of the type of containers used, the operator’s safety and prevention of cross-contamination are key factors, which must be considered. For such reasons, glass ampoules previously used for germplasm cryopreservation have become obsolete due to the explosion hazard when not sealed properly.
4.3.1 Cryovials
All of the above-mentioned hazards, however, may also, to a lower extent be applied to various plastic cryovials (Cryo Tube™, Nunc A/S, Roskilde, Denmark; Nalgene Nunc International, Naperville, IL, USA) which—despite packages marked “for vapour storage only”—are often stored in LN. The most common cause of contamination is the faulty seal, leak or breakage of these containers in LN. Clarke (1999) reported that 45 % of cryovials without O-ring (Nunc) and 58 % of cryovials with an O-ring (Iwaki, Japan) absorbed LN during 3 h immersion in LN. Although manufacturers strongly recommend the use of a second skin (Cryoflex™ tubing, Nunc; or Nescofilm™, Merk Ltd, Dorset, UK), these measures are rarely applied in everyday practice.
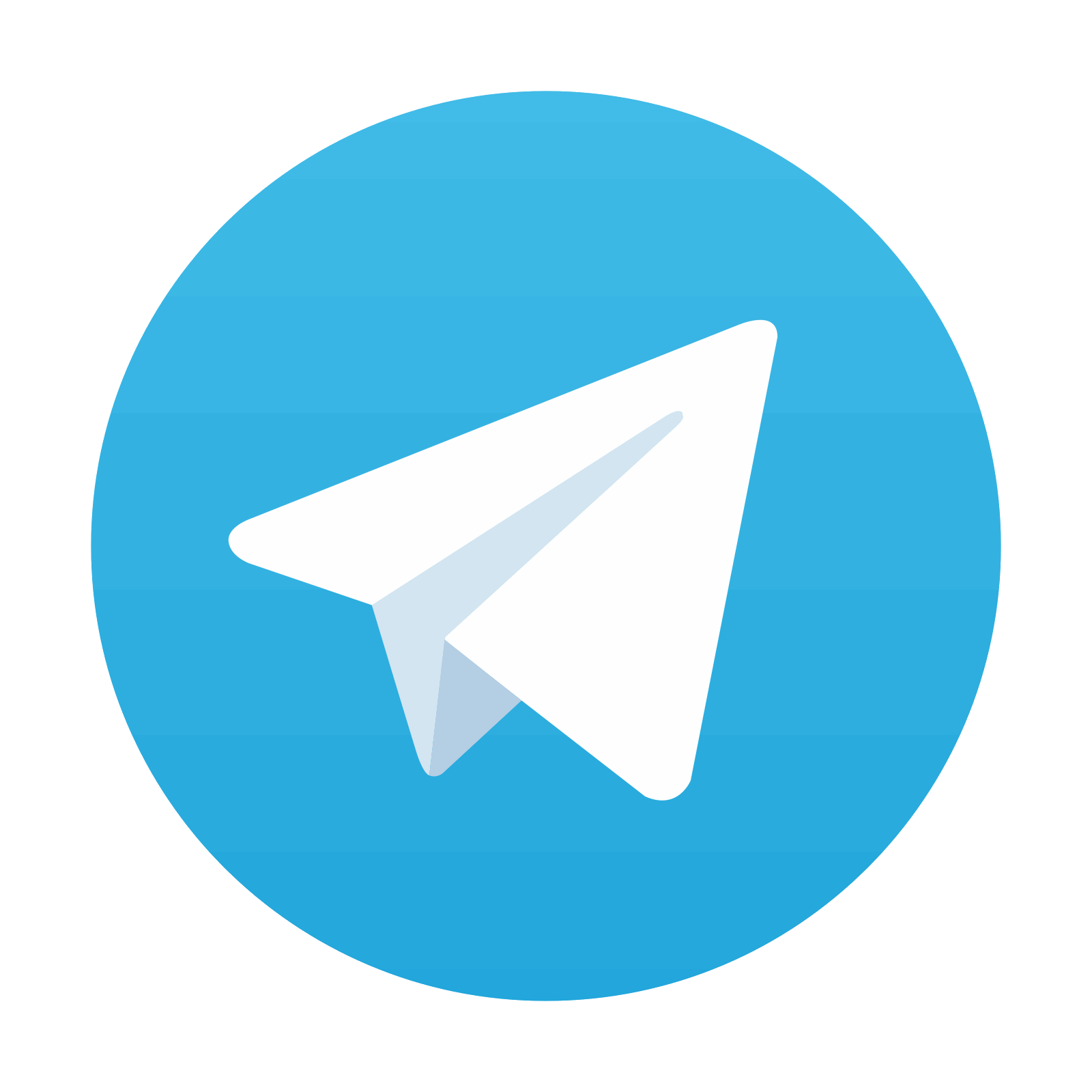
Stay updated, free articles. Join our Telegram channel

Full access? Get Clinical Tree
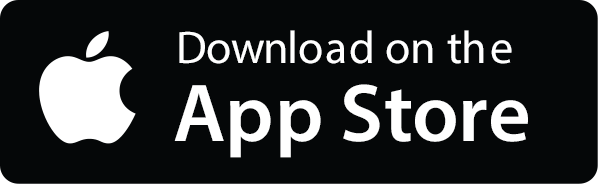
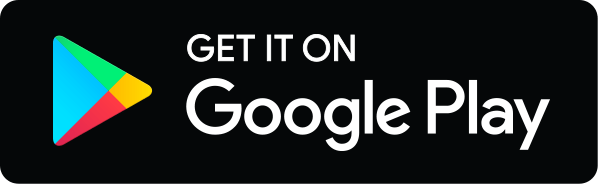