Fig. 1.
Expression of PKC isoforms in native tissues. PKC isoforms expressed in rat brain (RB), canine brain (CB), and canine basilar artery (BA) were examined by Western blotting. Samples were separated by SDS–PAGE (10% acrylamide gel) and immunoblotted with each PKC isoform-specific antibody. To examine the antibody specificity, antigen peptides were incubated with corresponding primary antibodies, shown in the right of each blott (shown as “Peptide (+)”). Arrows indicate the bands corresponding to each PKC isoform. Numbers on the left side of blot represent molecular weights (kDa). The following PKC isoforms were detected; α, β1, β2, γ, δ, and ε isoforms in rat brain, α, β1, γ, δ, and ε isoforms in canine brain, α and δ isoforms in canine basilar artery. From Nishizawa et al. (19) with permission.
5 Intracellular Distribution of PKC Isozymes: Implication of PKC Isozyme Activation
5.1 Background
As shown in Fig. 1, several PKC isoforms are usually expressed in an arterial tissue; e.g., α, δ, ζ, and η isoforms in canine basilar artery (19), α, δ, ε, and ζ isoforms in porcine coronary artery (22). Thus, it is important to clarify which PKC isoforms are involved in specific physiological/pathological phenomena, such as cerebral vasospasm following SAH. In Sect. 3, we described methods to measure PKC activity using radioimmunoassay and enzyme-immunoassay. However, these kits are not useful for measuring the activity of specific PKC isoform(s) due to the usage of a general PKC substrate. One way to examine the activity of specific PKC isoform(s) is to measure the intracellular distribution as PKC translocates from the cytosol to membrane fraction (23). Here, we outline a method used to examine the intracellular distribution of PKC isoforms using SDS–PAGE and Western blotting.
Recently, it has been reported that different stimuli activate specific PKC isoform(s) causing translocation to distinct subcellular compartments, which contributes to different physiological phenomena (24–26). In the future, detailed fractionation of cell membrane may provide precise information regarding the role of PKC isoform(s) in cerebral vasospasm after SAH.
5.2 Fractionation of Cerebral Artery
Canine basilar arteries are collected, and homogenized using a polytron in 400 μL of ice-cold homogenization buffer in 1.5-mL plastic tube. The homogenate is then ultracentrifuged at 100,000 × g at 4°C for 30 min. The supernatant (cytosolic fraction) is removed and placed into new tube and kept on ice. The pellet (membrane fraction) is resuspended in homogenization buffer containing 0.1% Triton-X, and incubated on ice for 30 min to extract proteins from membrane. Protein concentrations in samples of each fraction are measured by protein assay (details of protein assay are in Sect. 1.2). After protein assay, ×2 modified Laemmli sample buffer (400 μL) is added, and the sample is boiled for 5 min and then quenched in ice-cold water. Samples are stored at −20°C until use.
5.3 SDS–PAGE/Western Blotting and Analysis
Samples of each arterial fraction are examined using the same procedure used for “identification of PKC isoforms” described in the previous section. To make a comparison between fractions, the same amount of protein (10 μg) is run through the gel, and cytosol and membrane fractions from same artery are applied to adjacent lanes. After SDS–PAGE and followed by Western blotting using PKC isoform-specific primary antibodies, the immunoreactive bands are detected on X-ray film. The densities of each band are quantified by densitometric analysis using Image J software (NIH). The quantity of PKC isoforms in each fraction is expressed as “percentage of total PKC” calculated by following equation:
PKC in the fraction (%) = BD in the fraction/(BDcytosol + BDmembrane).
BD: band density; BDcytosol: band density in cytosol fraction; and BDmembrane: band density in membrane fraction.
Figure 2 shows the chronological changes in the intracellular distribution of PKC α and δ isoforms in canine basilar artery in canine “two-hemorrhage” SAH model.
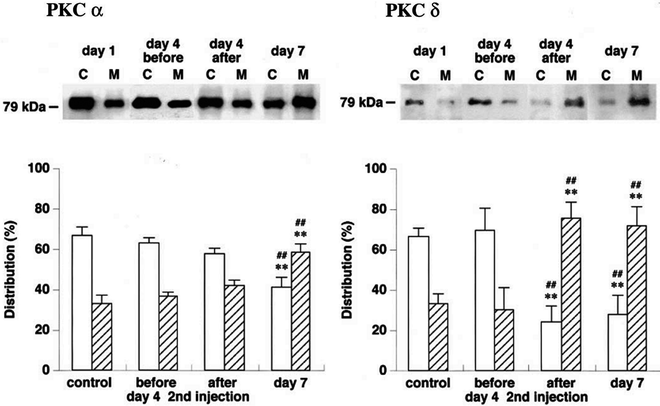
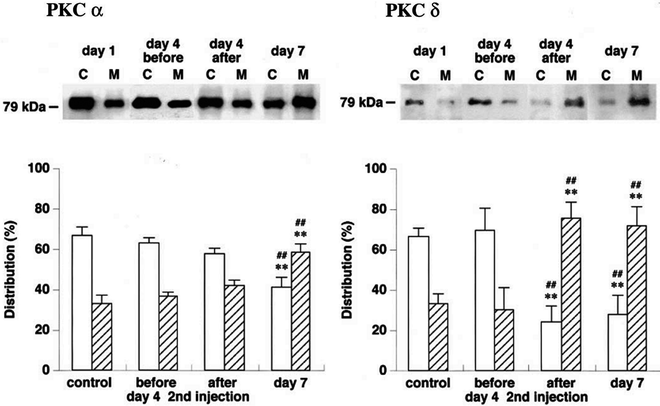
Fig. 2.
Intracellular distribution of PKC α and δ isoforms in canine basilar artery following SAH. Basilar arteries were obtained from a “double-hemorrhage” dog SAH model. The arterial homogenate was ultracentrifuged at 100,000 × g for 30 min, and supernatant was used as cytosol fraction (C, opened column), the pellet was resuspended and used as membrane fraction (M, shaded column). The immunoreactive bands were obtained using the same procedure used for “identification of PKC isoforms” shown in Fig. 1. The quantitative summary is expressed as a percentage of the total amount of each PKC isoform. **P < 0.01 vs. control, ## P < 0.01 vs. day 4 before second injection in same fraction. From Nishizawa et al. (19) with permission.
6 Measurement of Protein Tyrosine Kinase Activity
6.1 Background
Protein tyrosine kinases are enzymes that catalyze phosphorylation of specific tyrosine residues and are classified into two families; (1) membrane-spanning (receptor-type) tyrosine kinases and (2) nonreceptor type tyrosine kinases. Receptor-type PTKs are transmembrane single polypeptide chains, consisting of two domains; an extracellular ligand-binding domain and an intracellular tyrosine kinase domain. Receptor-type PTKs remain inactive until their ligand(s) binds to the extracellular domain, which generally leads to receptor dimerization, autophosphorylation, and increased tyrosine kinase activity. Examples of receptor-type PTKs include epidermal growth factor receptor (EGFR), fibroblast growth factor receptor, platelet-derived growth factor receptor, and vascular endothelial growth factor receptor. Nonreceptor PTKs are located in the cytoplasm and nucleus, and function as second messengers. Nonreceptor PTKs are divided into ten subfamilies; Src, Abl, Jak, Ack, Csk, Fak, Fes, Frk, Tec, and Syk (27).
Receptor-type and nonreceptor PTKs play crucial roles in various physiological phenomena (e.g., cell differentiation) and pathophysiological phenomena (e.g., carcinogenesis) (27, 28). In the vasculature, PTKs are involved in smooth muscle proliferation, migration, and contraction (29, 30), and are known to be activated in cerebral arteries after SAH (31–33). In this section, we describe a method to measure PTK activity using a commercially available nonradioactive assay kit, which was used in our previous study (32). Using canine basilar arteries from double hemorrhage SAH model, total PTK activity was measured using Universal Tyrosine Kinase Assay Kit (Takara Bio Inc.). It is also possible to measure the activity of some specific PTK (e.g., EGFR and FAK) using this assay system by adding immunoprecipitation protocols (details are described in kit instructions).
6.2 PTK Assay
Canine basilar arteries are obtained as described in Sect. 1.1, and minced in ice-cold extraction buffer provided in the kit, containing 1 mM sodium orthovanadate and 50 mM NaF. After sonication for 20 s (six times), the extract solution is centrifuged at 10,000 × g for 5 min at 4°C, and the supernatant is used to measure PTK activity. The standard solution of PTK activity provided in the kit is serially-diluted to obtain a standard curve. Diluted PTK standard solution or prepared samples (40 μL) are placed in an assay plate immobilized with general PTK substrate. Solution containing ATP (40 mM, 10 μL) is added to each well, and incubated at 37°C for 30 min. After washing each well with PBS (in mM: 137 NaCl, 2.68 KCl, 8.1 Na2HPO4, and 1.47 KH2PO4) containing 0.05% Tween 20 (PBS-T), blocking solution (provided in the kit) is added and incubated at 37°C for 30 min. The blocking solution is then discarded, and 50 μL of horseradish peroxidase-conjugated anti-phosphotyrosine antibody is added and incubated at 37°C for 30 min. Following a washing of the wells with PBS-T, 100 μL of horseradish peroxidase substrate solution is incubated at 37°C for 15 min to develop color. The reaction is terminated by adding 1 N H2SO4, and the absorbance of each well is measured at 450 nm using a microtiter spectrophotometer. Values are calculated using a standard curve, and normalized by protein concentration. PTK activity is expressed as U/μg protein.
7 Conclusions
Cerebral vasospasm, an abnormal and severe constriction of cerebral arteries, is frequently observed several days after SAH. Irrespective of the exact identity of specific spasmogens, enhanced contractile signaling and attenuated vasodilatory signaling may both contribute to the development of cerebral vasospasm following SAH. Here, we described procedures to examine intracellular signaling involving cGMP, PKC, and PTK to determine their contribution to cerebral vasospasm after SAH. These procedures can often be adapted and used to examine the activity of other molecules known to regulate smooth muscle contraction. Further research on the actions of these intracellular signaling molecules is likely to provide a greater understanding of contractile mechanisms and novel therapeutic targets for cerebral vasospasm following SAH.
< div class='tao-gold-member'>
Only gold members can continue reading. Log In or Register a > to continue
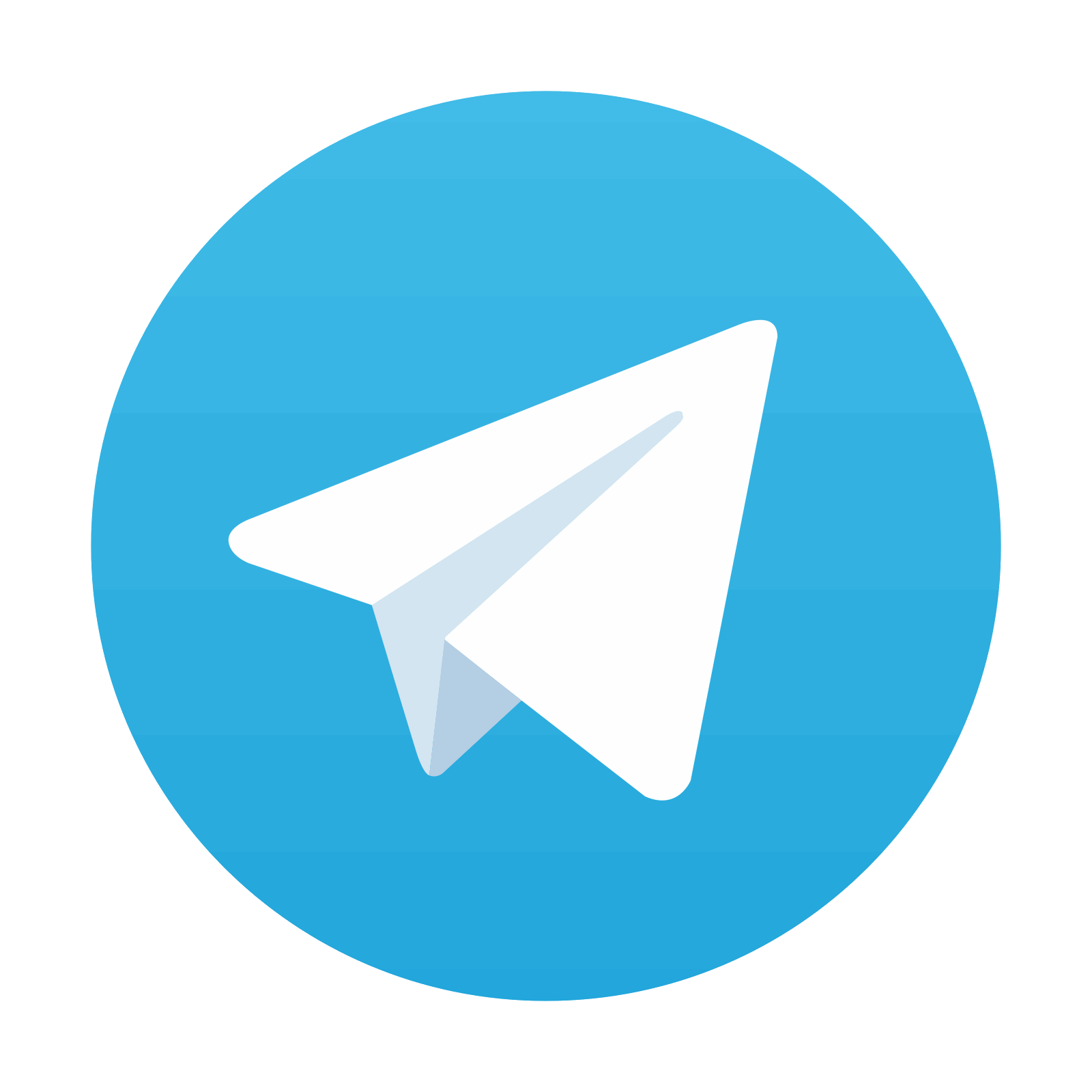
Stay updated, free articles. Join our Telegram channel

Full access? Get Clinical Tree
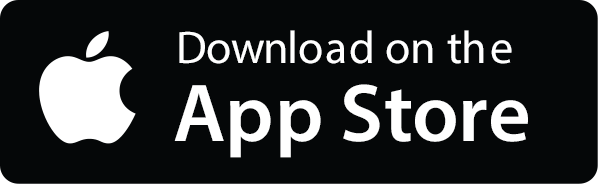
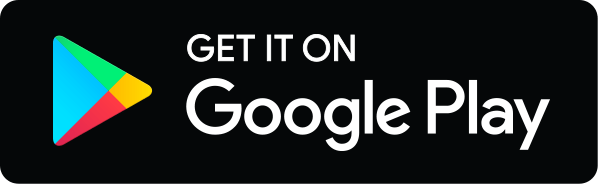