Fig. 10.1
Actograms and periodograms of representative Intact, Nursing-ZT03, and Nursing-ZT19 groups. (a, c, e) Double-plotted actograms of Intact (a), Nursing-ZT03 (c), and Nursing-ZT19 (e) groups. Black and white bars at bottom represent light–dark (L/D) condition. Shaded areas represent geographical night. Black vertical line represents time of nursing. (b, d, f) Periodograms of locomotor activity of actograms of Intact (b), Nursing-ZT03 (d), and Nursing-ZT19 (f) females indicate a rhythm of 24 h (X 2, P < 0.001) (Meza et al. 2008)
Whether nursing is scheduled in the day or the night, locomotor behavior is maximal at around the same hours and consequently the does behave mostly as diurnal or as nocturnal, depending on the time at which nursing is imposed (Meza et al. 2008). This shift in locomotor behavior suggests an alteration in the circadian oscillations of the SCN. Indeed, as shown in Fig. 10.2, in intact control females, PER1 in SCN has a rhythm that reaches a peak at ZT11 (ZT0 = 7 a.m., lights on), but timing of nursing decreases PER1 at times of their maximal expression when compared to intact does (Meza et al. 2008).
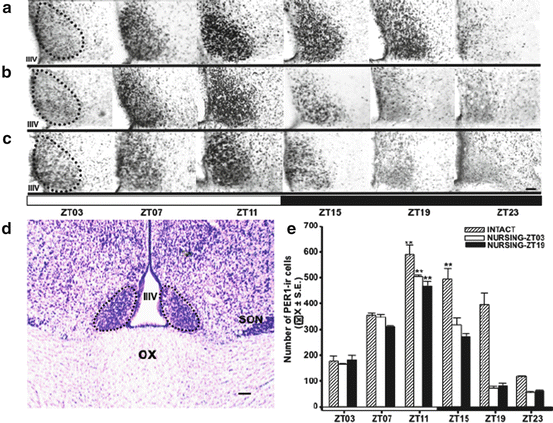
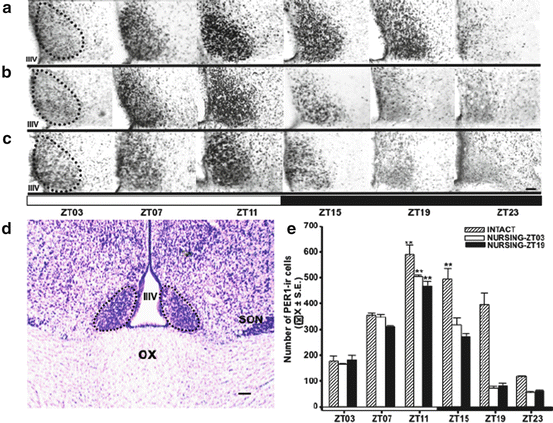
Fig. 10.2
Rhythmic expression of PER1-ir in the suprachiasmatic nucleus (SCN) in intact and nursing does. (a–c) Micrographs of representative sections illustrating the expression of PER1 protein at the level of the middle portion of the SCN in Intact (a), Nursing-ZT03 (b), and Nursing-ZT19 (c) groups at six different time points throughout a complete 24-h cycle. (d) Micrograph (thionine stain) denotes the level at which analyses were performed. Dotted line delimits SCN. IIIV third ventricle, OX optic chiasm. (e) PER1-ir cells in Intact, Nursing-ZT03, and Nursing-ZT19 groups. **P < 0.001 for differences between the higher and the lower within the same group. Values are mean ± SEM. Black and white bar at bottom of c and e represents L/D condition. Bar = 100 μm; ZT zeitgeber time, ZT0 = light on at 07:00 (Meza et al. 2008)
In contrast, nursing deprivation for 48 h significantly increases PER1 levels at peak time, in comparison to nursed females (Meza et al. 2011; Fig. 10.3). In the Syrian hamster, exposure to a novel running wheel downregulates clock genes in the SCN (Maywood et al. 1999). This stimulus is considered a nonphotic cue that induces a decrease, contrary to the upregulation of clock genes by a light pulse (Yan and Silver 2004). On the basis of this evidence we propose that suckling stimulus is a nonphotic stimulus for the rabbit SCN.
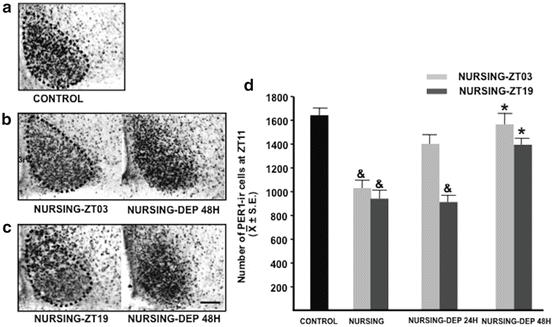
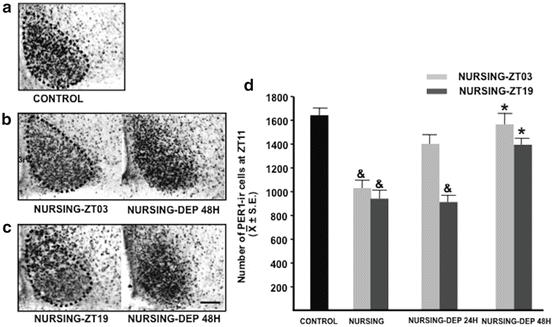
Fig. 10.3
Expression of PER1 in the suprachiasmatic nucleus (SCN). Left: Micrographs of representative sections illustrating the expression of PER1 at the level of the middle portion of the time of their maximal expression (ZT11) in control (a), Nursing-ZT03 (b), and Nursing-ZT19 (c) does and in corresponding nursing-deprived subjects for 48 h. Right: (d) Quantitative analysis of PER1 in control, Nursing-ZT03, Nursing-ZT19, and nursing-deprived subjects for 24 and 48 h. *P < 0.05, significant difference between nursing group and respective nursing-deprived group.& P < 0.05, significant difference versus the control group. 3V third ventricle. Values are given as the mean ± SE. Bar = 100 μm, ZT zeitgeber time, ZT0 = light on at 07:00 (Meza et al. 2011)
In other species, such as the Djungarian (Phodopus campbelli) and Siberian (Phodopus sungorus) hamsters, during lactation there is a significant decrease in amplitude of the circadian activity and wheel-running rhythms as the result of an abrupt decrease in mean dark-phase activity during around the first two thirds of lactation (Scribner and Wynne-Edwards 1994). Also in the rat, free-running activity decreased during lactation (Rosenwasser et al. 1987). This decrease in activity is correlated with an increase in the time spent in the nest, but does not implicate a phase shift as in the rabbit doe.
10.3.2 Body Temperature
In rodents, during lactation the dam shows hyperthermia, in comparison to cycling and pregnant subjects (Woodside and Leon 1980; Scribner and Wynne-Edwards 1994). Perhaps this hyperthermia occurs because the mother has frequent contact bouts with her pups. Indeed, she nurses her young every 24–54 min for a duration of 20–30 min each bout, without an apparent 24-h rhythm (Lincoln et al. 1973 ). However, even though dams have longer contact bouts during the day, their temperature continues to reach a peak during the night (Leon et al. 1984; Scribner and Wynne-Edwards 1994). In contrast, in the rabbit the peak of temperature does not occur in a phase-dependent manner but depends on the timing of lactation. During pregnancy, temperature has a circadian rhythm with a peak that free-runs with a period length > 24 h (Jilge et al. 2001). After parturition, however, the temperature peak coincides with the only once-daily lactation bout. Temperature starts to increase around 3 h before nursing, reaching highest values at the time of nursing (Jilge et al. 2001). This result supports the proposal that rabbit mothers have a circadian clock mechanism that “anticipates” nursing time, which seems to affect behavioral, neural, and physiological parameters (Caba and González-Mariscal 2009). Then, it is possible that daily suckling modulates the circadian temperature rhythm, although this had not been explored.
10.3.3 Mammary Gland
Recently, studies in several species have led to the discoverery that the mammary gland has a circadian clock. In mice, clock genes and their proteins change their expression in the mammary gland according to the development and reproductive cycle of females. For instance, PER1 protein was not detected in the mammary glands of virgin mice but was robustly expressed in ductal epithelial cells during lactation (Metz et al. 2006). Moreover, the changes of clock genes in the mammary epithelial cell lines are associated with expression of specific differentiation markers. In virgin and pregnant females, the mammary glands show a high expression of Per2 mRNA, which coincides with high levels of c-Myc and Cyclin D1 mRNA. These genes are downstream of the circadian-controlled genes that regulate cell-cycle progression (Nagoshi et al. 2004) and indicate growth and proliferation in undifferentiated cells of the mammary gland (Metz et al. 2006). Furthermore, Per1 and Bmal1 mRNA levels were elevated in late pregnant and lactating mammary tissues, and this was correlated with high levels of β-casein mRNA, which indicates differentiation. Also, alteration of the amplitude, but not of the phase, in the circadian expression of clock genes was found in the mammary gland during lactation when compared with that of virgin females (Metz et al. 2006).
Similar changes have been also found in other species. In rats, during lactation there is a coordinated change in the expression of core clock genes and their regulators, with an increase of the proteins of the positive limb in relationship to those of the negative limb during lactation in comparison to pregnant females (Casey et al. 2009). In cows at mid-lactation, there is also a circadian pattern expression of core clock genes mRNA isolated from milk fat globules, which correlates with the percentage of milk fat (Plaut and Casey 2012). In humans, genes specific to the mammary epithelial cells from milk fat globules show a circadian expression during lactation. Approximately 7 % of genes, including circadian core and clock-controlled genes involved in cell development, growth, proliferation, and cell morphology, showed highest expression at different hours through the day (Maningat et al. 2009). As a whole, the evidence indicates that expression of clock genes is important for the observed changes in morphology and metabolic capacity of the mammary gland according to the reproductive state of the females. As in the mammary gland, the transition from pregnancy to lactation implicates parallel changes in other peripheral tissues such as the liver. During this transition, an upregulation of genes related to P450 pathways catalyzes many reactions involved in synthesis of cholesterol, steroids, and other lipids, while at the same time a downregulation of genes related to the breakdown of fatty acids allows fats to be spared for milk synthesis (Casey et al. 2009).
The circadian oscillation of clock genes in the mammary gland suggests that this is important for the regulation of diurnal variations in milk composition in mammals (Nielsen et al. 2003; Cubero et al. 2007; Lubetzky et al. 2007), and this has been confirmed in human neonates. Analyses of proteins, carbohydrates, nucleotides, and amino acids indicate that the composition of breast milk follows a circadian pattern (Cubero et al. 2007). The consequences of these differences had been tested in relationship to consolidation of the sleep–wake rhythm. About 30 % of bottle-fed infants present difficulties in sleeping during the night, but enrichment of formula milk according to its differential day-versus-night components significantly improves the development and consolidation of the normal sleep–wake rhythm (Aparicio et al. 2007). It had been proposed that a key component related to this effect is the amino acid tryptophan, which is related to the synthesis of the hormone melatonin, which is secreted only during the night (Aparicio et al. 2007).
Besides these changes in peripheral tissues, there is also evidence of changes in clock genes and their protein products at brain level. The rabbit doe offers a unique opportunity to explore the physiological significance of clock genes in neuroendocrine cells. As mentioned, lactation in the rabbit is circadian; hence it is possible that neuroendocrine cells associated with the production of hormones related to milk production and yield could be affected by the periodic suckling of pups.
10.4 Entrainment of Neuroendocrine Systems During Lactation
10.4.1 Entrainment of Neuroendocrine Oxytocinergic System
In the rabbit, oxytocinergic cells are located in the supraoptic (SON) and paraventricular hypothalamic (PVN) nuclei and in the lateral hypothalamic area (LHA), as in other mammals (Caba et al. 1996; Hou-Yu et al. 1986). In the rabbit, oxytocinergic cells undergo dramatic changes between estrus and the transition period from pregnancy to lactation. The number of oxytocin (OT) cells increases significantly between estrus and late pregnancy/postpartum day (PD) 1 in the LHA, with a twofold increase in the PVN between estrus and PD1 (Caba et al. 1996). Moreover, there also is a significant increase in the OT somal area at PD1 compared with both estrus and late pregnancy (Caba et al. 1996). Also, in the rat, the oxytocinergic system undergoes morphological changes in the number of cells and their processes from pregnancy to lactation (Jirikowski et al. 1989), sustained by a significant increase in the synthesis of OT mRNA (Caldwell et al. 1987). These morphological changes around and after parturition are related to the behavioral and physiological changes induced by OT during these periods, as the onset of maternal behavior and mother–pup bonding (Lévy et al. 1992; Pedersen et al. 1994 ; Febo et al. 2005). After parturition, there are profound changes in the mother as lactation implicates a complex reorganization of the mother’s physiology to support the energetic demand of pups, and as maternal behavior implicates profound organizational changes in the brain.
In the rabbit doe the brief suckling stimulus of less than 5 min daily is enough to maintain the amount and rate of milk secretion, in contrast to rodent species, which need a more frequent suckling stimulus through the day (Grosvenor and Mena 1974). This suckling stimulus elicits a massive release of both OT and PRL into the blood every 24 h (Fuchs et al. 1984), and pups ingest around 30 % of their body weight in milk in just less than 5 min at PD7 (Caba et al. 2003). In considering that, in contrast to rodents, the rabbit lactation is circadian, we aimed to explore first whether SON and PVN express PER1 and, if so, then to evidence a possible rhythm and its possible synchronization in these two structures by periodic nursing. To this aim we explored the effect of suckling in neuroendocrine cells by immunohistochemistry of PER1 and OT, by scheduling nursing at two different times: one group nursed during the night and another nursed during the day.
Under light–dark (L/D) conditions, both SON and PVN in control does (nonpregnant and nonlactating females) expressed a rhythm of PER1 protein with a peak at the early night at ZT15 in the PVN and at ZT07–11 in the SON. This rhythm is affected by nursing. Whether nursing was scheduled in the day or in the night, this rhythm shifted and PER1 peaked 4–8 h after suckling by pups. This effect was specific to these nuclei as PER1 rhythm in the SCN did not shift in any condition (Meza et al. 2008; Fig. 10.2). Moreover, lactation also synchronizes PER1 rhythm in oxytocinergic cells. In both SON and PVN, PER1 in OT cells had a rhythm that reached a peak at ZT15, but again this peak shifted to 4–8 h after nursing (Meza et al. 2008; Fig. 10.4).
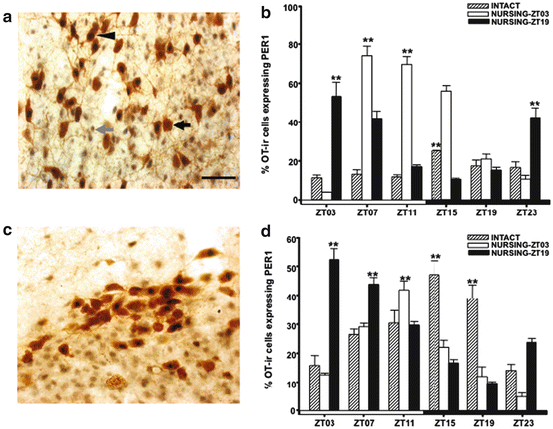
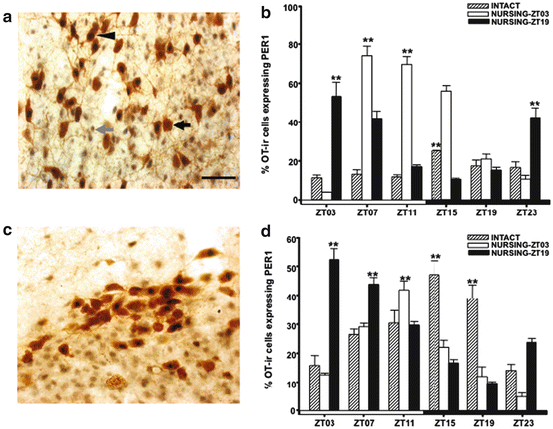
Fig. 10.4
Induction of PER1-/OT in the paraventricular nucleus (PVN) and supraoptic nucleus (SON). Micrographs of representative sections of PVN (a) and SON (c) in nursing does. Gray arrow, PER1; black arrow, OT; arrowhead, PER1/OT. (b, d) Percentages of PER1-/OT in (b) PVN and (d) SON of intact, Nursing-ZT03 and Nursing-ZT19 groups. **P < 0.01 for differences between higher and lower values within the same group. Values are mean ± SEM. Black and white bar at bottom of b and d represents L/D condition of female rabbits. ZT zeitgeber time (ZT0 = light on at 07:00). Bar = 50 μm (Meza et al. 2008)
10.4.2 Entrainment of Neuroendocrine Dopaminergic System
Prolactin (PRL) secretion is under an inhibitory control by dopamine, although some releasing factors, as oxytocin, have been also identified (Grattan and Kokay 2008). In the rat, three dopaminergic populations release dopamine (DA) into the hypothalamo-hypophyseal portal blood: they are tuberoinfundibular (TIDA) and tuberohypophyseal neurons with cell bodies in the arcuate nucleus (AR), and periventricular hypophysial (PHDA) dopaminergic population with cell bodies in the periventricular region, to the sides of the third ventricle (Ben-Jonathan and Hnasko 2001). PRL secretion has a robust circadian rhythm that persists under constant lighting conditions (Mai et al. 1994), perhaps supported by clock genes oscillations. In fact, dopaminergic cells in the arcuate nucleus express Per1 and their proteins differentially during the day versus the night (Kriegsfeld et al. 2003; Sellix et al. 2006). The Per1 rhythm is in phase with that of the light–dark cycle and shifts accordingly to advances or delays of the light schedule (Abe et al. 2002), suggesting that this rhythm is controlled by the SCN. Indeed, neuroanatomical evidence indicates SCN projections to the AR, and destruction of the SCN abolishes circadian rhythms of PRL (Bethea and Neill 1980).
The presence of clock genes in dopaminergic cells, however, also suggests that besides the SCN, these cells could be entrained by a specific timed stimulus other than the L/D cycle. To explore this possibility we evaluated the effect of suckling stimulus on PER1 expression in three dopaminergic populations: TIDA, PHDA, and incertohypothalamic dopaminergic (IHDA) populations. Both TIDA and PHDA, but not IHDA, have been found to be involved in the control of PRL. Then, we explored two populations that project to the adenohypophysis and one population, IHDA, that projects to other regions of the brain (Cheung et al. 1998). Dopaminergic populations were identified by tyrosine hydroxylase (TH), the rate-limiting enzyme of catecholamine synthesis, in considering that DA is the only catecholamine produced in these three cell groups (Björklund and Lindvall 1984). Similar to the foregoing experiment with OT, we explored the effect of suckling on dopaminergic cells by immunohistochemistry of PER1 and TH, scheduling suckling at two different times, with one group nursing during the night and another nursing during the day.
Double-labeled PER1/TH cells were identified in all three nuclei in all conditions. However, their number changes according to the physiological condition of the subjects and timing of nursing. In control nonlactating females, PER1/TH cells reach a peak at ZT15, but this maximal expression shifts to 4–8 h after timing of nursing in TIDA and PHDA neurons; data on TIDA neurons are presented in Fig. 10.5. In contrast, in IHDA the number of PER1/TH cells was scarce and did not change significantly in any of the conditions explored (Meza et al. 2011).
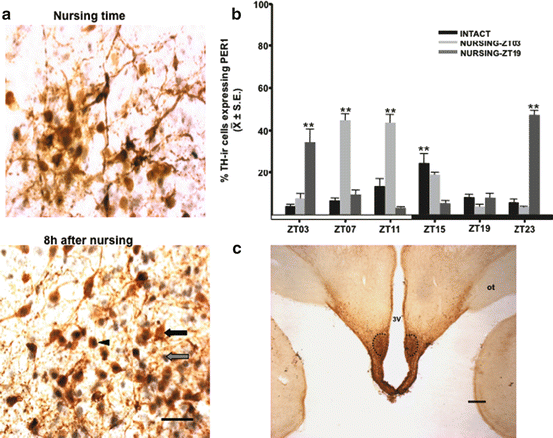
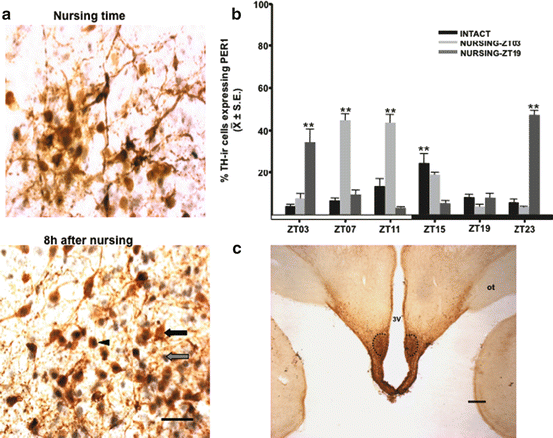
Fig. 10.5
Coexpression of tyrosine hydroxylase (TH) cells with PER1 protein (PER/TH) in the female rabbit brain. (a) Micrographs of representative sections of tuberoinfundibular dopaminergic (TIDA) populations at the time of nursing and 8 h later in does nursing at ZT03. Grey arrow, PER1 staining; black arrow, TH staining; arrowhead, PER1/TH double-labeled cells. Bar = 50 μm. (b) Percentages of PER1/TH cells in TIDA in Nursing-ZT03 and Nursing-ZT19 groups compared to intact subjects. **P < 0.01 for differences between higher and lower values within the same group. Values are mean ± SEM. Black and white bar at the bottom represents light/dark (L/D) condition. ZT zeitgeber time (ZT0 = light on at 07:00). (c) Micrograph of representative section of tuberoinfundibular dopaminergic (TIDA) population Dotted line delimits the area where analysis was performed. 3V third ventricle, ot optic tract. Bar = 1 mm (Modified from Meza et al. 2011)
10.4.3 Which Is the Entraining Signal to Neuroendocrine Cells?
We hypothesized that suckling is the signal for entraining of the oxytocin and dopaminergic neuroendocrine cells. Concerning OT, PER1 in OT cells significantly decreased at peak time in both SON and PVN in lactating females that did not receive this stimulus during 48 h (Meza et al. 2008). Regarding TH, we observed a similar effect in PHDA but not in TIDA neurons, which suggests a differential effect of suckling on TH populations (Meza et al. 2011). In contrast, at PD7 the suckling stimulus induces FOS, a protein considered an index of neural activation, in the SON and PVN in comparison to nonnursing females (González-Mariscal et al. 2009). In the rat, suckling by pups also induces FOS in OT cells in both SON and PVN and OT mRNA in PVN cells (Eriksson et al. 1996). Moreover, in the rat, absence of suckling induces an upregulation of TH mRNA in arcuate neurons, observed as early as 1.5 h after the pup’s removal. Furthermore, prevention of suckling by covering one side of the dam’s teats induces an upregulation of TH mRNA in the contralateral side of the arcuate (Berghorn et al. 2001).
At behavioral level, experiments in the rabbit support the importance of suckling stimulus on the doe. It was found that there is a critical threshold, that is, amount of nipple stimulation, that determines the “turn-off” of the nursing bout and consequently the time in the nest box (González-Mariscal et al. 2012). Importantly, the time that the mother spends inside the nest is normal when provided with 6–8 pups and occurs just once a day with circadian periodicity, but when fewer pups are provided she shows multiple entrances to the nest (González-Mariscal et al. 2013). This experiment confirms that not only suckling, but also its intensity, are critical to maintain the circadian rhythmicity of lactation.
10.4.4 What Is the Significance of the Shift of the PER1 Rhythm in Neuroendocrine Cells in the Lactating Rabbit?
Evidence from several species indicates that the suckling stimulus is transmitted through the spinal cord and brainstem to the hypothalamus, to both PVN and SON (Wakerly et al. 1994). Also, FOS is induced in the PVN as a consequence of suckling (Tsingotjidou and Papadopoulos 1996). It has also been proposed that afferent stimulus from the nipples affects TIDA neurons, regulating TH mRNA (Berghorn et al. 2001). We proposed previously that the suckling stimulus shifts clock gene central core oscillations in neuroendocrine cells in the rabbit during lactation (Meza et al. 2008, 2011). Several lines of evidence support this proposal. It is well known that oscillations of these genes are reset by a photic stimulus (Yan and Silver 2004). However, experiments in vitro and in vivo indicate that some stimuli, other than light, can also affect oscillations of these genes. A serum shock, administration of various compounds such as cAMP, protein kinase C, glucocorticoid hormones, or Ca2+ to a cultured rat-1 fibroblast cell line, can all trigger a surge of Per1 transcription and elicit and shift circadian expression of clock genes and other genes (Balsalobre et al. 2000). In adult rats, exposure to stressors (Takahashi et al. 2001) or scheduled feeding (Caba et al. 2008; Feillet et al. 2008) also induces a similar effect in several brain nuclei. Overall we consider that suckling seems to be the main stimulus that entrains clock gene oscillations in neuroendocrine cells in the rabbit. During normal conditions, the SCN sends signals to the brain and peripheral tissues to maintain synchronization, and the clock gene oscillations are in phase with that of the master clock (Abe et al. 2002; Granados-Fuentes et al. 2006; Kriegsfeld et al. 2003). However, it had been proposed that, during special conditions, some local cells in specific nuclei uncouple from the SCN oscillations and now respond to specific needs (Kriegsfeld and Silver 2006). These local clocks then shift their oscillations and drive the expression of particular clock-controlled genes that act as outputs to regulate particular physiological conditions. By using the rabbit doe we confirmed that dopaminergic cells contain the molecular machinery necessary to oscillate independently and also that clock genes oscillations seem to uncouple from the SCN and now are entrained by a different stimulus, specifically suckling.
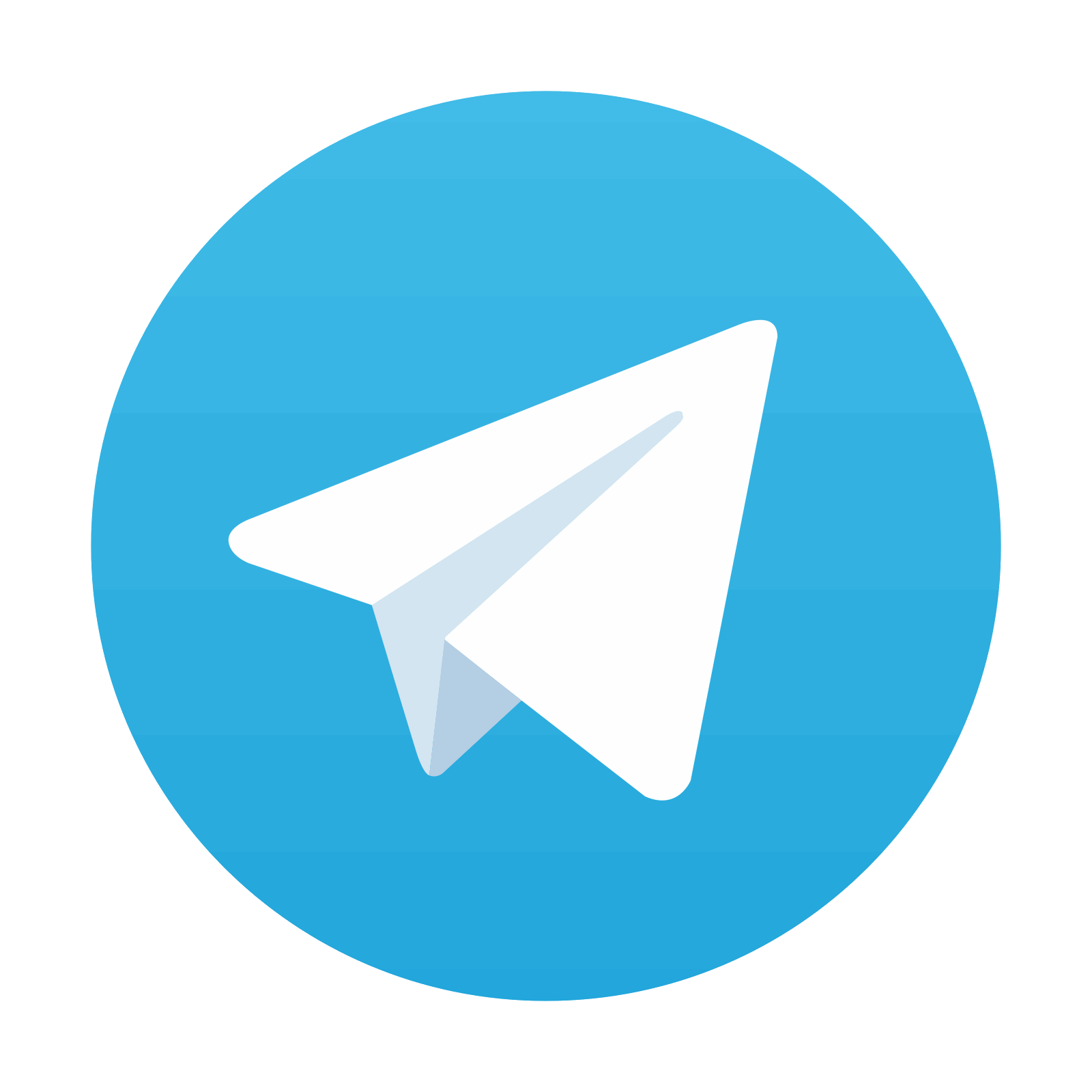
Stay updated, free articles. Join our Telegram channel

Full access? Get Clinical Tree
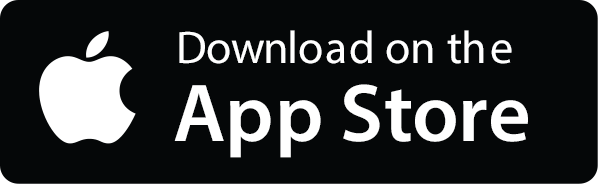
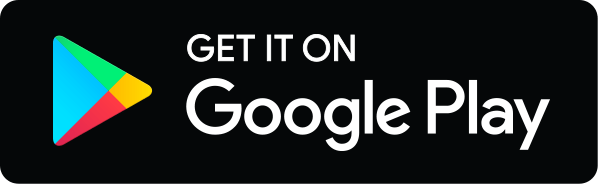