11 Avian Metapneumoviruses 1University of Veterinary Medicine, Hannover, Germany; Avian metapneumovirus (aMPV) is a nonsegmented, negative-strand RNA-enveloped virus that is the primary causal agent of turkey rhinotracheitis (TRT), also known as avian rhinotracheitis (ART). The virus was first isolated from turkeys in South Africa during the late 1970s (Buys and du Preez, 1980; Buys et al., 1989a, 1989b). Thereafter, outbreaks were reported in many European, South American and Asian countries and recently also in the USA (Andral et al., 1985; Alexander et al., 1986; Weisman et al., 1988; Senne et al., 1997; Cook et al., 1999; Seal, 2000). Although vaccines have been developed, outbreaks can also be traced to environmental spread of a vaccine-derived virus (Lupini et al., 2011) and the disease is exacerbated by bacterial infections (Cook et al., 1991). aMPV also causes swollen head syndrome in chickens (McDougall and Cook, 1986; Wilding et al., 1986; Wyeth et al., 1987; Cook et al., 1988; Buys et al., 1989b) and outbreaks among chickens have been reported in various parts of the world (Otsuki et al., 1996). In addition, aMPV can infect ducks (Toquin et al., 1999), pheasants (Gough et al., 1988; Welchman et al., 2002), guinea fowl (Picault et al., 1987) or ostriches (Cadman et al., 1994). aMPV RNA or infectious particles have also been isolated from asymptomatic free-ranging water birds, sparrows and starlings in the USA (Shin et al., 2000a; Bennett et al., 2002). The data on the susceptibility of pigeons to aMPV infection is controversial (Felippe et al., 2011; Gharaibeh and Shamoun, 2012). aMPV causes an acute highly contagious infection of the upper respiratory tract in turkeys that was originally referred to as turkey rhinotracheitis, hence the original name turkey rhinotracheitis virus (TRTV). Disease appeared in the UK during the mid-1980s and spread rapidly, affecting turkey flocks of all ages (Anon, 1985; Naylor and Jones, 1993). The early aMPV isolates from Europe included UK/3B/85 and CVL14/1 strains from the UK (Cavanagh and Barrett, 1988; Collins and Gough, 1988; McDougall and Cook, 1986; Yu et al., 1991), 1556 strain from France, 872S strain from Spain and the 2119 strain from Italy (Collins et al., 1993; Li et al., 1996). All these isolates had high nucleotide sequence and antigenic similarities (Juhasz and Easton, 1994; Li et al., 1996; Randhawa et al., 1996). Nucleotide and amino acid sequence comparisons of the highly variable attachment G gene led to classification of the European viruses as two distinct subgroups, A (aMPV/A) and B (aMPV/B), consistent with the nomenclature adopted for mammalian pneumoviruses (Collins et al., 1993; Naylor et al., 1997). It was also demonstrated that these two subgroups had varied patterns of neutralization by monoclonal antibodies recognizing the surface attachment glycoprotein G (Collins et al., 1993; Cook et al., 1993). Contrary to initial reports indicating that French and UK isolates belonged to subtype A, detailed sequence analyses showed that only the UK isolates belonged to subtype A, whereas other continental European strains, including French isolates formed subgroup B (Collins et al., 1993; Juhasz and Easton, 1994; Naylor et al., 1997). Subsequently subgroup B strains were also isolated in the UK (Naylor et al., 1997; Bäyon-Auboyer et al., 1999). Two aMPV strains isolated in France during 1985 lacked antigenic similarity to the A or B strains (Toquin et al., 2000) and had low nucleotide or amino acid sequence identity with aMPV/A, aMPV/B and aMPV/C viruses, hence were classified into a fourth subgroup D (aMPV/D; Bäyon-Auboyer et al., 2000). However, this subgroup has not been reported again since that time. The USA was considered free of aMPV until the first outbreak occurred among commercial turkeys in the state of Colorado during 1996 (Senne et al., 1997; Cook et al., 1999; Seal, 2000) and prior to that time no serological reactivity was detected among North American commercial poultry (Heckert and Myers, 1993). The outbreak in Colorado, a state with a small commercial turkey population, lasted for only 10 months before eradication, primarily by slaughter and stringent biosecurity measures (Edson, 1997; Goyal et al., 2000; Jirjis et al., 2000). Comparison of the nucleotide and predicted amino acid sequences of the fusion (F) and matrix protein (M) genes from the Colorado aMPV isolate with European isolates indicated that the US viruses had significantly different genetic identity resulting in tentative classification of US viruses as subgroup C (Seal, 2000; Alvarez et al., 2003). At approximately the same time, aMPV outbreaks were reported in the north central US state of Minnesota. During subsequent years (1997–2002), aMPV disease has emerged as a major economic problem for turkey farmers in the state of Minnesota, which is one of the largest turkey-producing states in the USA (Chiang et al., 2000; Goyal et al., 2000; Panigrahy et al., 2000). So far, two distinct sublineages of aMPV/C have been identified in the USA (Padhi and Poss, 2009). Recently, aMPV/C of a different genetic lineage to the US isolates was also detected in Muscovy ducks in France (Toquin et al., 1999) and in Korean pheasants (Lee et al., 2007). This lineage of subtype C aMPV was also later detected in Europe among mallards, greylag geese and common gulls (van Boheemen et al., 2012). The wide geographic distribution and detection of aMPV/C in a variety of wild bird species suggest a fast distribution of this subtype and a potential threat to the poultry industry worldwide (Padhi and Poss, 2009). The aMPV genome consists of eight viral protein genes arranged in the order 3′-N-P-M-F-M2-SH-G-L-5′ flanked by a leader and trailer at the 3′ and 5′ ends, respectively (Fig. 11.1). The linear genomic RNA molecule is tightly encapsidated by the nucleocapsid (N) protein that associates with the phosphoprotein (P) and large polymerase (L) proteins to form a helical ribonucleoprotein complex, which is the biologically functional unit common to all members of the order Mononegavirales. The ribonucleo-protein complex is the template for transcription of mRNAs as well as genome replication (Conzelmann, 1998). aMPV shares several characteristics with other members of the Pneumovirinae subfamily (Cavanagh and Barrett, 1988; Yu et al., 1991), including possession of small hydrophobic (SH) and second matrix (M2) genes (Ling et al., 1992; Yu et al., 1992a, 1992b) and structure of the F protein (Naylor et al., 1998). The M2 gene of aMPV-C contains two overlapping open reading frames (ORFs), encoding two putative proteins, M2-1 and M2-2, which are believed to be involved in viral RNA transcription or replication (Yu et al., 2011). However, aMPV differs from members of the Pneumovirus genus, including human and bovine respiratory syncytial virus (hRSV and bRSV), in that it lacks two non-structural proteins located upstream of the N gene (Randhawa et al., 1997; Marriot et al., 2001). In addition, the SH and G genes of pneumoviruses are located upstream of the F gene, whereas they are located downstream of F gene for aMPV (Ling et al., 1992). As a result of these observations, aMPV was classified into a new genus called Metapneumovirus (Pringle, 1999). Fig. 11.1. Schematic structure and genomic organization of avian metapneumoviruses. European viruses have 97% to 99% nucleotide and amino acid predicted sequence identity within a subtype, whereas strains across subtypes have 56% to 61.2% nucleotide sequence and 33.2% to 38% predicted amino acid sequence identities within the highly variable attachment glycoprotein (G) gene (Juhasz and Easton, 1994). More detailed analyses of nucleotide sequence of N, P, M, fusion glycoprotein (F) and M2 protein genes revealed that these genes could also be used to classify aMPV strains into the four subtypes (Seal, 1998; Bäyon-Auboyer et al., 2000; Shin et al., 2002; Jacobs et al., 2003). For example, US viruses classified as subtype C (aMPV/C) had over 90% nucleotide sequence identity within the five genes, whereas comparison with subtypes A and B revealed between 40% and 70% nucleotide sequence identity (Shin et al., 2002). Phylogenetic analyses of subtypes A, B and C strains demonstrated that A and B viruses were more closely related to each other than either subtype A or B were to C viruses (Seal, 1998; Shin et al., 2002). Typical for paramyxoviruses, the upstream five genes (N, P, M, F and M2) of aMPV/C were easily sequenced at the nucleotide level due to the abundance of mRNA transcripts, whereas SH, G and L genes were more difficult to obtain due to the paucity of RNA. aMPV was the only recognized member of the Metapneumovirus genus, until isolation of a human metapneumovirus (hMPV) in the Netherlands during 2001 (van den Hoogen et al., 2001). Subsequently, hMPV strains were isolated in many countries throughout the world (reviewed by Feuillet et al., 2012). Analysis of the genomic sequences from hMPV demonstrated a close resemblance to aMPV sub-type C rather than to aMPV/A, B or D based on nucleotide sequence and phylogenetic comparisons (van den Hoogen et al., 2002; Toquin et al., 2003). The nucleotide sequence similarity between aMPV and hMPV utilizing N, P, M, F, M2 and L ranged from 56% to 88% (van den Hoogen et al., 2002). The presence of two aMPV N gene-encoded polypeptides was detected in aMPV/C/US/Co- and aMPV/A/UK/3b-infected Vero cells (Alvarez and Seal, 2005) and also in hMPV-infected cells (Tedcastle et al., 2012). Natural aMPV-infection, as indicated by experimental inoculations of susceptible hosts, is via the upper respiratory tract (Jones et al., 1986). As with other related viruses of the Pneumovirinae subfamily, the aMPV G-protein is considered to mediate virus attachment to host cells (Collins and Crowe, 2007), which are mainly ciliated epithelia cells (Fig. 11.2), but macrophages are also hypothesized to be permissive for aMPV replication (Jirjis et al., 2002a). However, potential receptors have not yet been identified (Winter et al., 2008). The F-protein mediates fusion of the viral envelope with the cell membrane, and contributes to host tropism, leading to fusion of infected and non-infected neighbouring cells with typical syncytia formation (Tanaka et al., 1996; de Graaf et al., 2009). Host proteases are necessary for activation of the F-protein by cleavage, but sequence analysis of vaccine and field strains suggests that the F-protein cleavage site may not be an important feature of viral virulence (Sugiyama et al., 2010). Fig. 11.2. Detection of avian metapneumovirus (aMPV) by immunohistochemistry. Arrows indicate aMPV-infected ciliated cells of tracheal organ cultures at 96 hours post-infection. European aMPV strains cause deciliation of the trachea among infected turkeys at approximately 96 hours post-infection, with the virus inducing watery to mucoid exudates in the turbinates and excess mucus in the trachea from 1–9 days post-infection (Jones et al., 1986, 1987). By direct immunofluorescence staining, virus is detected in the trachea between days 1 and 7 post-infection and in the turbinates between days 2 and 5 post-infection; however, no virus was demonstrated in the lungs or air sacs. aMPV replication in ciliated epithelial cells of the female chicken and turkey reproductive tracts has also been demonstrated under field and experimental conditions (Jones et al., 1988; Cook et al., 2000). Viral antigens were detected by immunofluorescence in the epithelium of the uterus on day 7 post-infection, and all other regions of the oviduct on day 9 post-infection (Jones et al., 1988). These infections may lead to significant drops in egg production, egg peritonitis, misshapen eggs accompanied by ovary or oviduct regression (Hess et al., 2004; Sugiyama et al., 2006). Field outbreaks are exacerbated due to secondary bacterial pathogens inducing air sacculitis, pericarditis, pneumonia and perihepatitis (Cook, 2000). Infections with mycoplasma (Naylor et al., 1992) or Escherichia coli, Bordetella avium, Ornithobacterium rhinotracheale or a mixture of all three can increase loss of cilia on the epithelial surface of the upper respiratory tract, allowing deeper penetration of the virus into the respiratory tract and exacerbating infection by aMPV (Jirjis et al., 2004). Microscopic examination of the turbinates from infected turkeys usually reveals increased glandular activity with loss of cilia, congestion and mono-nuclear infiltration of the submucosa (Majo et al., 1995, 1996). The US subtype C aMPV produces multifocal loss of cilia in the nasal turbinates of infected turkeys and immunohistochemistry revealed intense staining of aMPV antigen in turbinates and tracheae of birds infected with wild-type isolates (Jirjis et al., 2002a; Velayudhan et al., 2008). Gross lesions among chickens include a purulent oedema in the subcutaneous tissues of the head, neck and wattles, with varying degrees of swelling in the infraorbital sinuses (Hafez, 1992; Tanaka et al., 1995). Clinical signs such as depression, coughing, nasal exudates and frothy eyes appeared at 4 days post-infection in chickens, followed by swelling of periorbital sinuses at 5 days post-infection and serological conversion occurring within 10 days post-infection (Catelli et al., 1998; Aung et al., 2008). Interestingly, nasal turbinates of hMPV-infected turkeys showed inflammatory changes and mucus accumulation along with positive immunohistochemistry, demonstrating that the human virus closely related to aMPV/C will infect and replicate in turkeys (Velayudhan et al., 2006). Virus neutralizing antibodies following aMPV infection can be detected locally in tracheal washes and systemically in serum within 5–7 days post-infection, independent of the strain, but titres decrease quickly again on local respiratory surfaces, suggesting only a short duration of local protective immunity (Liman and Rautenschlein, 2007; Rautenschlein et al., 2011). Virus neutralizing antibody titres peak at 7 days post-infection and then antibody levels decline, with peak serum ELISA antibody production varying among infected groups, ranging from 14 and 28 days post-infection. Virulent strains may markedly decrease the proliferative response of spleen cells to concanavalin A (Chary et al., 2002). aMPV strains induce an increase in the percentage of CD4+ T cell populations in the spleen and Harderian gland (HG) at days 7 or 14 post-infection in turkeys and of CD4+ and CD8+ cells in the HG in chickens in the early phase (Rautenschlein et al., 2011; Liman and Rautenschlein, 2007). Investigations with chemically bursectomized turkeys indicate that cell-mediated immunity in turkeys is important for protection against aMPV infection (Jones et al., 1992). Furthermore, T-cell-deficient turkeys partially depleted of functional CD4+ and CD8+ T-lymphocytes by cyclosporin A (CsA) treatment showed a delay in viral clearance, recovery from aMPV-induced clinical signs and histopathological lesions compared to T-cell-intact birds (Rubbenstroth et al., 2010). Differences in systemic and local T cell and possibly natural killer cell activity in the HG between turkeys and chickens may explain the differences in aMPV-pathogenesis between chickens and turkeys (Rautenschlein et al., 2011). There are a variety of diagnostic strategies utilized for aMPV detection in clinical samples (Fig. 11.3; Cook and Cavanagh, 2002). The time period for successful virus detection after infection is short and for broilers experimental studies indicate that virus detection may not be successful beyond the peak of clinical signs, which is expected around 6 days post-infection (Aung et al., 2008). Upper respiratory tract tissue, tracheal, sinus and choanal swabs may be most appropriate to detect aMPV (Van de Zande et al., 1999; Pedersen et al., 2001). Isolation of this slowly replicating virus was first successful utilizing tracheal organ cultures (Buys and du Preez, 1980; Buys et al., 1989a); however, this technique was not useful for detecting US aMPV/C replication since it does not induce ciliostasis (Cook et al., 1999). Embryonating turkey or chicken eggs can be used to isolate the virus, but serial passage is usually required before embryo lethality can be detected to confirm isolation (Buys and du Preez, 1980; Senne et al., 1997). Chicken embryo fibroblasts and Vero cells in culture can be utilized to successfully propagate aMPV, but blind passage may be necessary prior to observing cytopathic effect for confirmation of virus replication (Goyal et al., 2000; Coswig et al., 2010). The virus visualized by electron microscopy is a characteristic Paramyxovirus with a pleomorphic, spherical (100–600 nm) or filamentous (to 1000 nm) particle having helical nucleocapsids (14 nm) with glycoprotein projections of 13–14 nm. Immuno-gold labelling of TRT/aMPV in Vero cells was also used to confirm morphology and morphogenesis (O’Loan et al., 1992). Fig. 11.3. Diagnostic strategy for detection of avian metapneumovirus infections. *Serum samples should be obtained at 2–3-week intervals for detecting an increase in antibody titres. Immunofluorescence of tissues and clinical smears were used to detect European strains as well as aMPV/C isolate of the virus (Usami et al., 1999; Van De Zande et al., 1999; Jirjis et al., 2002b). An ELISA method was developed for detecting antibodies to TRT utilizing antigen from a virus isolated from an outbreak of the disease in Europe (Grant et al., 1987; Chettle and Wyeth, 1988) and this assay was improved upon by utilizing a streptavidinbiotin detection protocol (O’Loan et al., 1989). The choice of antigen may have a negative effect on the ability to detect viral antibodies in turkey sera (Eterradossi et al., 1995). Consequently ELISAs based on recombinant M (Gulati et al., 2000) and N (Gulati et al., 2001a) proteins of aMPV/C were developed. A nucleoprotein (N)-peptide ELISA was created capable of detecting antibodies to all three aMPV types with a reliable antigen (Alvarez et al., 2004b) and polyclonal or monoclonal antibodies reactive to a conserved region of the aMPV/C N cross-reacted with the hMPV N protein, but not with RSV N protein by ELISA, Western blot and immunohistochemical assays (Alvarez et al
2The Pirbright Institute, Compton Laboratory, Compton, UK;
3Agricultural Research Service, USDA, Athens, USA
11.1 Introduction
11.2 Molecular Biology of aMPV Isolates
11.3 Pathobiology of aMPV
11.4 Diagnostics and Prevention of aMPV Disease
Stay updated, free articles. Join our Telegram channel

Full access? Get Clinical Tree
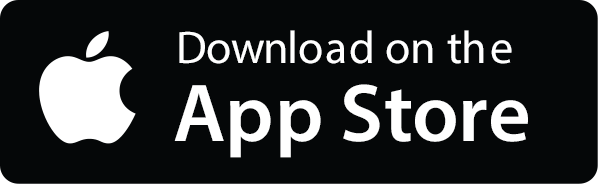
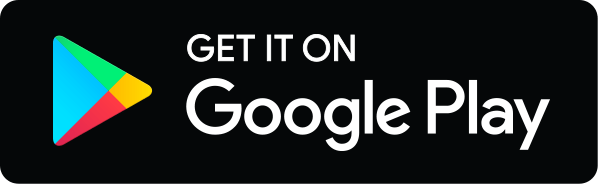