CHAPTER 112 Assisted Reproductive Technologies in Swine
Manipulation of animal reproduction probably is as old as domestication itself. Since humans started to keep animals in captivity, they have exerted a profound influence on the natural behavior of domesticated species, including reproduction. Assisted reproductive technologies will allow continued genetic advancement in farm livestock. Artificial insemination (AI), used by the Arabs in ancient times for the dissemination of male gametes and first described for publication by Lazzaro Spallanzani in 1784, probably was the first technique of modern “assisted reproduction.” The first embryo transfer work, in rabbits, by Walter Heape in 1891 and the subsequent successful embryo transfers in many other species over the next century turned out to be the first step in increasing the female role in reproduction. Other assisted reproductive technologies have been developed and applied to swine under research settings. The in vitro fertilization (IVF) of oocytes and micromanipulation of domestic animal embryos, first started in the early 1980s, nowadays are accepted as commonplace and as vital components of several procedures that are rapidly transforming the embryo transfer industry. In addition to these technologies, embryo cryopreservation, intracytoplasmatic sperm injection (ICSI), oocyte transfer, and nuclear transfer are dramatically altering the very manner in which we view our own reproductive capacity. It is important to recognize, however, that assisted reproductive technologies in general do not directly apply from one species to another, as has been well illustrated by the work with pigs.
The first successful pig embryo transfer was reported in 1951 (Table 112-1), the same year as for cattle. Of all of the large domestic animal species, however, the pig is the animal for which implementation of these reproductive technologies on a production basis has been the slowest. Several unique features of the physiology of reproduction in swine, as well as technical limitations, have slowed the widespread use of embryo transfer in pigs relative to its application in the cattle industry. Part of this hesitancy can be attributed to the greater delicacy and sensitivity of swine gametes and embryos to manipulation and in part to the perception that little is to be gained owing to the high reproductive efficiency that this species naturally possesses. An additional impediment to the greater use of reproductive technologies in swine is the highly variable response to synchronization and superovulation of donor animals; furthermore, collection and transfer of porcine embryo thus far have been accomplished only by surgery. The size of the donor and unique anatomic characteristics of the reproductive tract appear to make nonsurgical recovery an unlikely prospect. Although surgical embryo transfer has been successfully used, methods for the nonsurgical transfer of embryos into recipients are still being developed. The swine embryo transfer activity has just started to grow around the world, with an estimated 7000 embryos transferred either experimentally or commercially in 2000.1 Until now, however, the swine industry has never successfully implemented a commercial viable embryo transfer process as an alternative method of improving hog genetics. Despite the limitations, embryo transfer and related reproductive technologies hold promise as a means to accelerate genetic improvement and to take advantage of superior breeding sows, to break the link between health status of customer and source herds, to eradicate disease from a swine herd, and to facilitate inexpensive transport of genetic material around the world. This chapter presents an overview of recent work in this field, to collate existing knowledge and to establish guidelines for future work.
Table 112-1 Key Milestones in Development of Assisted Reproductive Technologies in Swine
Year | Event | Study |
---|---|---|
1951 | First successful surgical embryo transfer | Kvasnickii2 |
1970 | Successful intercontinental transport of embryos | Wrathall et al.3 |
1976 | Embryo transfer used to establish SPF herd | Curnock et al.4 |
1983 | Embryo transfer used to repopulate swineherds in connection with eradication of pseudorabies virus | James et al.5 |
1985 | Production of transgenic piglets | Hammer et al.6 |
1985 | Piglets produced from split embryos | Rorie et al.7 |
1986 | Piglets produced by in vitro fertilization | Cheng et al.8 |
1989 | First cloned piglet born after embryo cell nuclear transfer | Prather et al.9 |
1989 | Piglets produced after transfer of frozen-thawed (− 35° C) embryos | Hayashi et al.10 |
1990 | Piglets produced after transfer of frozen-thawed (− 196° C) embryos | Oguri11 |
1991 | USDA produced first swine offspring with semen sorted for sex, after surgical transfer | Johnson12 |
1993 | Production of piglets by nonsurgical embryo transfer | Reichenbach et al.13 |
1994 | First piglets produced after oocyte transfer (GIFT) | |
1997 | First piglets of preselected sex produced after transfer of embryos fertilized with X- and Y-sorted sperm | Rath et al.14 |
1998 | First piglets born after embryo vitrification—USDA | Dobrinsky et al.15 |
2000 | First piglet born after fertilization of an oocyte by intracytoplasmic sperm injection (ICSI) | Kolbe and Holtz16 |
2000 | First cloned piglets born after somatic cell nuclear transfer | Polejaeva et al.17 |
GIFT, gamete intrafallopian tube transfer, SPF, specific pathogen–free; USDA, U.S. Department of Agriculture.
ARTIFICIAL INSEMINATION STRATEGIES
Reduction in Sperm Number per Dose and Intrauterine Insemination
A conventional insemination technique in swine requires 2 × 109 to 3 × 109 sperm cells per dose and a volume of insemination per dose between 80 and 100 ml. Currently, however, interest has increased in the novel possibility of introducing a lower number of sperm cells per dose or lower volumes of insemination per dose directly into the uterus. In contrast with the traditional intracervical insemination method, instruments and techniques are currently being developed to allow the semen to be deposited beyond the sow’s cervix and into the uterine horns. This deep uterine insemination technology will have obvious economic repercussions in the overall productivity of boar stud facilities, and the cost per dose of semen may be reduced accordingly. Concepts for this technology began evolving in the early 1990s in an effort to help reduce the effects of summer infertility in hot climates by improving conception rates with use of poorer-quality semen. More recently, the main thrust for further refinement of this technique has been to advance the application of sperm sorted for sex and to develop a method for performing nonsurgical embryo transfer. Several manufacturers, in connection with research institutions, have created a growing array of catheter devices that are can be passed through the cervix of the sow to inseminate beyond the cervix. To date, however, research data are not sufficient to confidently establish the necessary cell numbers, semen volume, and semen placement location. Research reports demonstrate that the number of semen per dose could be reduced significantly without a corresponding decrease in either fertility or litter size. Recent results using a fiberoptic endoscope technique for deep uterine horn insemination in sows demonstrated that using only 50 to 200 million sperm cells per dose was now possible.18 This number represents only a fraction of the 2 to 3 billion sperm commonly used in conventional AI. By this calculation, 400 to 500 doses could be potentially prepared from each ejaculate. With this technique it was possible to insert the insemination catheter past the cervix to deposit semen in the anterior region of one uterine horn in about 90% of the sows, with subsequent farrowing rates and litter size of 85% and 9.5 piglets, respectively. Although endoscopic deep uterine insemination is an alternative technique for insemination of a small number of spermatozoa in sows, the flexible fiberoptic endoscope is an expensive and fragile instrument to be used under field conditions.18 For this reason, a new catheter for insemination with flexibility and propulsion force similar to those of the fiberoptic endoscope was used in a subsequent trial.19 Sows were inseminated within the anterior region of one uterine horn 36 hours after administration of human chorionic gonadotropin (hCG) with 150, 50, 25, or 10 million sperm cells in a total volume of 10 ml of Beltsville thawing solution (BTS) diluent. Investigators were able to inseminate and impregnate almost 90% of the sows, and no difference in litter size was observed. Only use of large doses of 50 million and 150 million sperm cells for deep insemination, however, resulted in pregnancy rates comparable with those achieved with insemination using a conventional dose of 3 billion sperm (74.4%, 80.9%, and 79.8% respectively). Apparently, by ligating the noninseminated horn, investigators were able to demonstrate that after deep insemination, sperm cells travel transperitoneally through the opposite infundibulum to fertilize oocytes ovulated from the contralateral ovary.
Several research institutions have experiments under way to validate low-dose, deep-uterine insemination techniques using different catheters for deposition of fresh, frozen-thawed semen with spermatozoa sorted for sex. In a recent commercially based field trial, a 200-mm catheter that extends over the insemination rod (Deepgoldenpig*) was used for deep insemination of sows with reduced sperm numbers.20 During this trial, doses of 1, 2, or 3 billion total spermatozoa diluted in X-cell extender* () to a standard volume of 80 ml in a flatpack bag were used to evaluate the insemination device in more than 3000 sows. At the two higher sperm doses, no difference in farrowing rates was found between the experimental insemination device and a control catheter, and both gave rates exceeding 90%, with a mean litter size of 12.4. At the 1 billion sperm dose with the conventional catheter, however, the farrowing rate dropped to 66% with a litter size of 10.3, but with the Deepgoldenpig, the farrowing rate remained not significantly different from that in the other groups at 87% with a litter size of 12.1. Currently, use of this insemination technique device provides a method to reduce the insemination dose for sows without serious loss in production. Because of difficulties with catheter insertion and increased risk of reproductive trauma and hemorrhage, intrauterine insemination is not recommended for gilts. At present, several practical issues prevent its routine commercial acceptance for farm use. This technique requires trained inseminating staff and good estrus detection to avoid late (postovulatory) inseminations.
Low-dose insemination in combination with a single (fixed-time) insemination has the potential to give an even higher economic advantage in genetic dissemination, with doubling of current boar productivity. Owing to the nature of the estrous cycle in pigs, however, it will be very difficult to predict the right time to perform a single-dose insemination per cycle to achieve a farrowing rate and litter size comparable with those obtained with multiple inseminations. Traditionally, multiple (two or three) inseminations are performed throughout estrus to increase the chances that one insemination will be performed at an optimal time relative to ovulation. In recent years, the use of real-time ultrasonography (transabdominal or transrectal) has helped redefine insemination strategies by characterizing ovulation patterns in the sow retrospectively. As a consequence, optimal time for insemination has been found to be in the interval from 28 hours before to 4 hours after ovulation.21 Ovulation in the pig normally occurs approximately 38 hours after the onset of estrus, but this timing can vary considerably between females (16 to 52 hours) and with genetic makeup and environment.22 In addressing the topic of potentially reducing the number of inseminations and therewith allowing the possible use of fixed-time AI, it is essential to recognize that accurate heat detection and familiarity with general estrous behavior in gilts or sows are essential components to improve the prediction of timing of ovulation and for the implementation of an AI schedule. Although up-to-date ultrasonography does not appear to be effective in predicting time of ovulation,23 defined relationships between the time of ovulation relative to estrous behavior have led to methods that allow producers to more accurately pinpoint the best time for insemination.24
SPERM SEXING TECHNOLIGY
Being able to preselect the sex of offspring at the time of conception ranks among the most sought-after reproductive technologies of all time. Sexing mammalian sperm has several important applications in the swine industry; however, sperm sexing will have a huge impact on the efficiency of existing multiplication systems. We are seeing dramatic changes in swine production with consolidation and vertical integration that affects how swine are produced and marketed. The use of sex preselection has great potential for increasing efficiency. In the future, those not taking advantage of efficiencies such as sex control may be buying animal products from those who do.25
For several years, investigators have attempted more than a dozen approaches to separate the X chromosome– and Y chromosome–bearing sperm based on perceived and assumed differences in physical characteristics or immunologic factors, with little success. Recently however, a new approach based on the well-established differences in DNA content between the two sex chromosomes has been the only consistently successful method of sorting sperm from a variety of species, including swine.26 This DNA fluorescence–activated cell sorting technique involves treating sperm with a fluorescent dye, Hoechst 33342, which binds to their DNA, and then sorting them into separate X- and Y-bearing sperm populations using a flow cytometer–cell sorter modified specifically for sperm to measure the relative fluorescent intensity reflective of DNA content of each sperm as it traverses a laser beam. Flow-cytometric separation of viable X chromosome– from Y-chromosome–bearing sperm is now a well-established technique and has proved to be repeatable at numerous locations and with many species at greater than 90% purity.27 On average, the X-bearing sperm of common mammals contain 3% to 7% (3.6% in swine) more DNA than the Y-bearing sperm.28 The wider the difference, the easier it is to sort with great accuracy.
Offspring of the predetermined sex in swine and other domestic animal species have been born after insemination using this technology since its introduction in 1989.12 The current technology, however, has certain obstacles that prevent a broader application. First, the number of spermatozoa that can be separated per hour is low (about 16 million X-bearing sperm at 73% to 80% purity); second, labeling with the fluorochrome decreases sperm viability, leading to a higher embryonic mortality; and third, the expenses associated with use of an appropriate flow cytometer are enormously high. Nevertheless, new technological advances in high-speed cell sorting technology are being made to make the process produce more viable X and Y sperm per unit time at the highest purity.27 Although the limited numbers of sperm available with flow-cytometric sperm sorting can be circumvented by using IVF or ICSI and subsequent embryo transfer, these are likely to be minor avenues for sex regulation relative to AI because semen can be delivered through different routes and techniques such as surgical (intrauterine or intratubal), deep uterine, cervical, and laparoscopic. In pigs, these sperm numbers are still insufficient for conventional insemination techniques; however, it recently has been shown that a numerical reduction in the number of sperm per insemination and the use of sorted sperm do not necessarily affect the pregnancy rates if semen is deposited surgically closer to the uterotubal junction a few hours before ovulation. It was demonstrated that the number of sperm inseminated can be reduced by several orders of magnitude in a low insemination volume down to 5 × 106 in 0.5 ml of Androhep extender per uterine horn in hormonally stimulated prepubertal gilts29 or in sows30 without significant reduction in rates of pregnancy or farrowing or in litter size.
Currently, sexing sperm is a tool that has matured sufficiently to be valuable for experimental purposes and for certain niche applications related to production animal agriculture. For wide agriculture applications, cost must be low and efficiency and convenience, high. Skewed sex ratios of 85% to 100% of one sex or the other have been repeatedly achieved in most species. In a preliminary experiment, pigs of the desired and predicted sex were born after surgical insemination at rates of 74% females and 68% males.12 Offspring all were morphologically normal and were reproductively capable in adulthood, showing no negative effects of the sorting process. With improved techniques for pig IVF, sexed sperm were used to fertilize in vivo–matured oocytes, and the resulting embryos were used to produce two litters of pigs, all of the desired sex.14 This was the first successful use of sexed sperm to produce offspring of preferred sex after transfer of sexed embryos derived by in vitro embryo production (IVP).
Improvements in IVF protocols led to more investigations in this field but now using ova matured in vitro. In one study, ova inseminations were done within 1 hour of completing the sorting process, and embryos were transferred at the four-cell stage.31 Thirty-four piglets were born from 6 embryo recipient sows; 33 were females and 1 was a male (97% success rate). In another study, in vitro matured pig oocytes were fertilized with either X- or Y-sorted sperm at 20 hours after sorting.32 Three litters were born from embryos produced from Y-sorted sperm, resulting in 9 male piglets (100%), and 5 litters were born from transferred embryos produced from X-sorted sperm, resulting in 23 females and 1 male (97%). Collectively, the results from these experiments demonstrated the effectiveness of using sexed sperm to produce sexed offspring in the pig by first producing sexed embryos. Currently, new flow cytometer machines are being perfected for commercial use through collaborations between the Beltsville Germplasm and Gamete Physiology laboratory and several scientists around the world.
Another approach for sperm sexing, currently under development, is based on the hypothesis that sex-specific proteins present on the surface of the sperm cells of a desired sex can be removed by polyclonal antibodies that, when added to the sperm, will result in agglutination of the cells. The free-swimming sperm cells can be then filtered off and used for fertilization of oocytes in vitro.33 The preliminary results of trials conducted in bovines suggested that this viable immunologic sperm sexing procedure has potential for application in other species, including the pig.
EMBRYO TECHNOLOGY
Embryo Transfer Procedure
Strictly speaking, the term embryo transfer technique refers only to the process that begins with embryo collection and continues through embryo transfer. The basic technique of embryo transfer consists of removal of an embryo from a genetically superior animal and introduction of that embryo into a host surrogate. The resulting offspring will have qualities that duplicate those of the embryo supplier while not retaining any characteristics of the surrogate. Although embryo transfer in pig was first reported more than 50 years ago (see Table 112-1), it has limited applications in commercial swine production. In the swine industries, the primary reasons for recovering and transferring embryos are to minimize the risk of disease transmission, to propagate genetically superior animals, and, in some cases, to simplify shipment of genetic material. In addition, the application of embryo transfer technologies to breeding strategies also will generate significant advancements in selection, by allowing for the constant introduction of new genetic material into nucleus breeding herds. Embryo transfer provides a complete genetic package to the purchaser, resulting in rapid genetic upgrading at the lowest possible cost and lowest risk of disease transmission. Embryo transfer has been well established as an important tool in research, and a great deal of current knowledge regarding reproductive physiology in swine has been a result of research studies using this technique. In practical application, embryo transfer comprises a complex series of measures including selection and stimulation of donor sows, recovery of embryos, embryo handling, and transfer of recovered embryos to recipients.
Superovulation and Donor Selection
Several available gonadotropin preparations have been used in swine to increase the number of ovulated oocytes. Traditional methods of synchronization accompanied by superovulation include the use of 1000 to 1500 IU of equine chorionic gonadotropin (eCG) (e.g., Folligon* or Novormon†) either 24 hours after weaning in sows or 24 hours after a luteolytic dose of prostaglandin F2α (PGF2α) (e.g., Lutalyse‡) administered on days 12 to 15 of the estrous cycle in mature gilts, or administered any time to prepubertal gilts. In cycling gilts, eCG also can be administered after an altrenogest (Regu-Mate*) feeding regimen providing 15 mg/day of altrenogest for 15 to 18 days for estrus synchronization. To induce ovulation, 500 to 750 IU of hCG (e.g., Chorulon*) also is administered 72 to 80 hours after eCG, and the animals are inseminated 36 hours after that. The response after such treatments can be highly variable, however, even in animals of the same weight and age. Factors such as product impurity due to luteinizing hormone (LH) content and lot-to-lot variation in bioactivity also may contribute to the variable response.
Using this hCG-eCG protocol, our laboratory has been able to obtain a mean of 23 ± 5.0 total ova or embryos per donor, compared with approximately 15.2 ± 3.8 from non-superovulated gilts. In a recent review of 12 studies using gilts as donors, however, investigators report superovulatory responses as high as 32 embryos collected, but in some cases no improvement in superovulatory response was observed.34 In addition, with repeated embryo collections, more than one batch of embryos per donor also could be obtained. The methods of embryo collection will determine how often collection from one donor is possible. The stage at which collection takes place (days after mating) has an impact on the type of technology to use. For non-superovulated animals, pubertal gilts and sows can be used successfully as donors. In my experience, a higher embryo yield has been obtained in mature gilts (those undergoing more than three estrous cycles) and sows. More recently, investigators reported that very young prepubertal gilts (approximately 100 days of age) were poor candidates for embryo donors or embryo recipients. Lower pregnancy rates were achieved when these animals were used as embryo donors and lower pregnancy rates and retardation of fetal growth when they were used as embryo recipients.35 It also has been reported that embryos from prepubertal gilts do not have the same in vitro developmental capacity as those from cyclic gilts when surgically collected at early stages of development and culture in vitro to the blastocyst stage.36
Embryo Collection Techniques
Embryo recovery in swine usually is accomplished surgically with the animal under general anesthesia, with the reproductive tract presented through a midventral incision in the caudal abdominal region involving retrograde flushing of the oviducts or uterine horns, or both. Surgery in swine is simple, inexpensive, and reliable once the necessary skills are developed. This surgical technique for embryo collection has been described previously and remains relatively unchanged.37,38
Other, less invasive alternative techniques for embryo collection also have been investigated. These techniques include nonsurgical embryo collection after surgical resection of the uterine horns39,40; endoscopic techniques41,42; and flushing of the reproductive tract after slaughter of the donor animal. Collectively, these techniques have some limitations, and results obtained with these procedures need to be improved. The number of ovulations, the age of the animal, the day of collection, the quality of the semen, and the fertility of the donor sow or gilt all influence the success of embryo collection. Results with traditional surgical embryo collection suggest that a success rate of about 90% recovery can be expected when an average group of donor gilts or sows is used.
Embryo Manipulation and Embryo Yield
After collection, the fluids are left to settle, to allow the embryos to sink in the bottom of the tube. Embryos are morphologically examined in vitro under a stereomicroscope and transferred into a holding or culture medium. Embryos also are evaluated according to their morphologic characteristics at the expected stage of development, and then viable (transferable) embryos are selected. Swine embryos usually are recovered over the first 5 to 6 days after the onset of estrus (about 120 hours after ovulation) to ensure that collection is performed while the embryos are still contained within the zona pellucida. At this stage, morula and early to expanded blastocysts generally are recovered. They also can be recovered at different stages of development, however. Embryos at the one-cell to the early four-cell stage are collected from the oviduct on days 1 to 3 after ovulation, whereas embryos from the four-cell stage up to the hatched blastocyst are flushed from the proximal uterine horn on days 4 to 7. Embryos with a difference of one cell cycle can be accepted as transferable, whereas embryos less developed could be left in culture for an additional 24 hours before transfer. Embryo evaluation 24 hours after culture gives a good indication of their developmental capacity, and embryos that do not develop after culture can be discarded.
In a study performing embryo collection in 229 non-superovulated donor gilts, our laboratory obtained a mean number of corpora lutea of 15.2 ± 3.8, a mean total number of ova or embryos of 13.4 ± 4.2, and a mean number of viable (transferable) embryos of 11.8 ± 4.8. These results translated to an 88.1% recovery rate and a proportion of transferable embryos of 86.0%. When gilts were grouped by the number of estruses detected (one to three or more) before embryo collection, however, a significant increase was demonstrated in the number of corpora lutea, total ova or embryos, and total number of transferable embryos as the number of estruses increased before collection.43 By contrast, when embryos were collected from non-superovulated sows (greater than fourth parity), a higher number of transferable embryos (up to 20) was obtained.
Embryo Transfer
Selection of recipient gilts has a major impact on embryo transfer results. Although prepubertal gilts can be used as recipients, cycling gilts and sows are preferred because of their stable endocrine and uterine development. As in embryo transfer programs in cattle, management of the recipient probably is an important factor affecting pregnancy rate. Recipient animals, in which the embryos are to be implanted, are not inseminated. For embryo transplantation to be successful, however, it is necessary for the recipient animal to be in the same stage of the estrous cycle as the donor. The degree of synchrony between donor and recipient needed during a surgical embryo transfer is not as precise as in cattle. Traditionally, during a surgical embryo transfer, investigators prefer to transfer embryos into recipients at either the same stage of estrous cycle (day 0) as in the donor or into asynchronous recipients (−24 hours) with a less advanced uterine development. It has been previously demonstrated, however, that during a nonsurgical embryo transfer procedure, recipients that ovulated between 24 hours before and 12 hours after the donor achieved a higher pregnancy rate. This finding suggests that transfer to recipients ovulating later than 12 hours after the donor is associated with very low pregnancy rates.44
Although several groups of investigators are making progress in the area of nonsurgical embryo transfer to recipients, traditional surgical transfer is still the method of choice for most embryo transfer programs.45 Surgical embryo transfer is carried out using a ventral midline laparotomy approach, after general anesthesia has been obtained. One of the ovaries and the adjacent proximal portion of the uterine horn are exteriorized from the surgical incision. After examination of the ovary for identification of a sufficient number of corpora lutea, the embryos are transferred, depending on their stage of development, either to the oviduct (one-cell to early four-cell embryos) or into the tip of the uterine horn (four-cell embryo to hatched blastocyst). Embryos are transferred in a small amount of medium by means of transfer pipettes or catheters.
Other procedures have been evolving for transferring embryos including transcervical (nonsurgical) techniques. For a long time, nonsurgical embryo transfer in pigs has been considered a technique that was almost impossible to perform. The reason was the complex nature of the genital tract with its constricted cervical canal and long, coiled uterine horns, as well as the location of the embryos in the proximal area of the horns during early pregnancy. These challenges have discouraged attempts at nonsurgical transcervical introduction of a catheter into the uterine horn. During the last decade, several experiments regarding nonsurgical procedures were reported in detail by five independent research groups, all using different approaches.13,44,46–49 The three main differences among various procedures are the use of sedation, the type of instrument used (AI spirette or special design), and the volume of fluid used for transfer. Collectively, results from these studies have demonstrated that nonsurgical transfer of embryos in swine is feasible and has resulted in pregnancy rates ranging from 9% to as high as 64% and average litter sizes ranging from 3.1 to 10.9 piglets. Although all groups used different approaches for their transfers, the best pregnancy rates were achieved without recipient sedation.44,49 On the other hand, specially designed instruments, which enable deposition of embryos into the uterus with a small volume of fluid, resulted in higher litter sizes (approximately 6 to 11 piglets).44,47,48 More recently, the nonsurgical embryo transfer technique in sows developed by Hazeleger and Kemp has been applied under commercial farm conditions.50 Nonsurgical transfers were performed in 45 synchronized recipient sows, each receiving 25 to 30 blastocyst-stage embryos. The farrowing rate (44%) and litter size (7.4 ± 3.2) obtained were lower than those achieved with the experimental conditions previously reported (59% and 10.9 ± 3.4, respectively).44 In a subsequent small trial, a modified flexible catheter, similar to the ones used with a fiberoptic endoscope, was successfully deployed to penetrate the anterior third of one uterine horn during early diestrus.51 With this procedure, a pregnancy rate and litter size of 72.7% (8/11) and 6.0 were obtained after transfer of 24 to 30 morula-to-blastocyst stage embryos, placed at a depth of 30 to 70 cm into the uterine horn in a small volume of medium (0.8 ml).
Endoscopic procedures for embryo transfer also have been recently developed.42,52,53 This minimally invasive technique has some advantages, including repeatability compared with surgical procedures, but requires expensive endoscopic equipment and very experienced handling and usually takes longer than laparotomy procedures do. The equipment consists of a small cold-light fountain, a light cable, a metal catheter for an oblique optic telescope, a metal catheter for an atraumatic forceps, and a metal catheter for the venous catheter. Five cm caudal to the umbilicus the catheter for the optic is introduced into the abdomen, and 10 cm lateral of the optic the catheter for the optic also is inserted. After observation of the ovary for the presence of corpora lutea, the forceps is used to stabilize the infundibulum and embryos are deposited by advancing the venous catheter. Using this technique, pregnancy rates greater than 40% and litter size ranging between 2 and 10 piglets have been reported.54
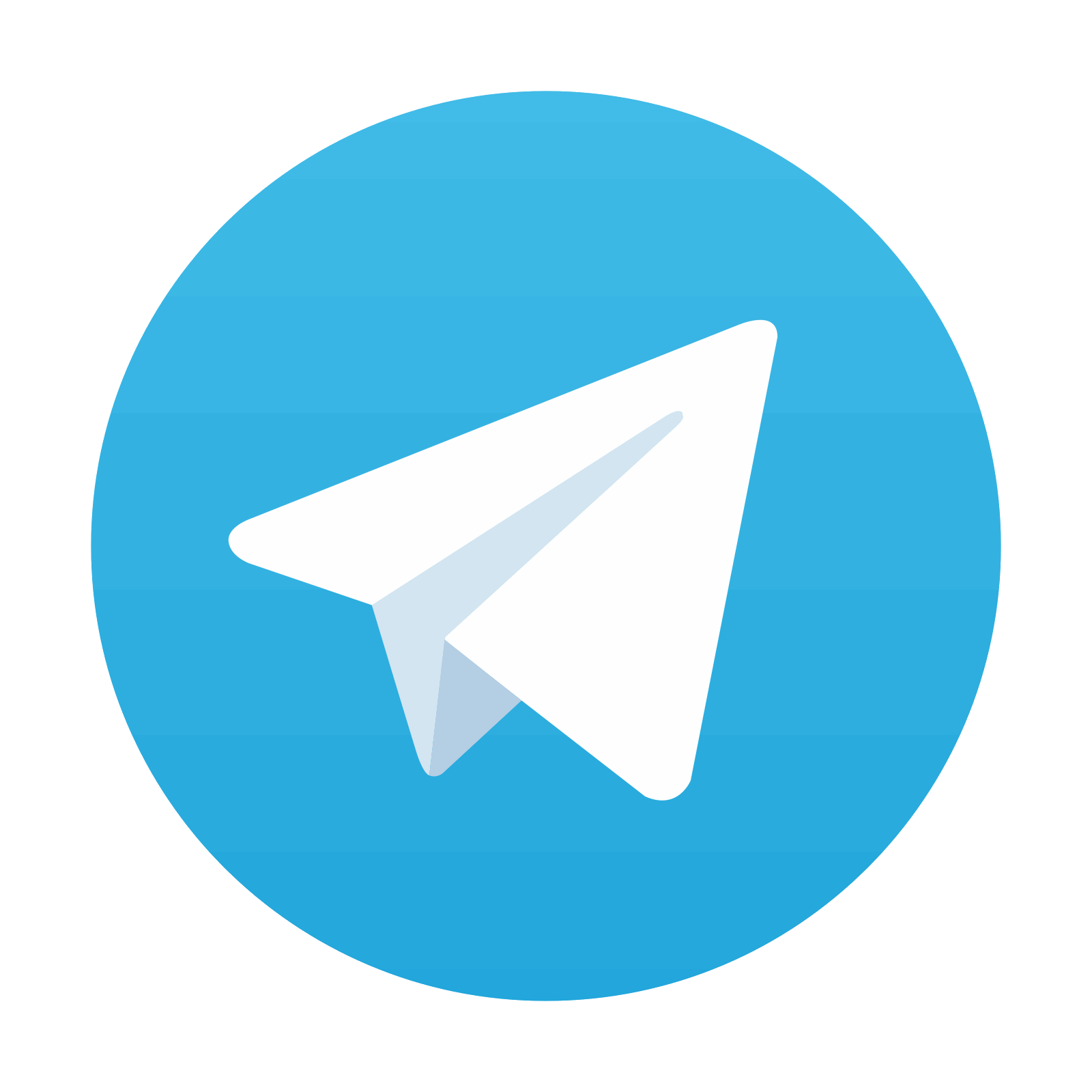
Stay updated, free articles. Join our Telegram channel

Full access? Get Clinical Tree
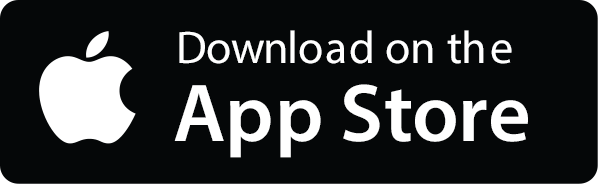
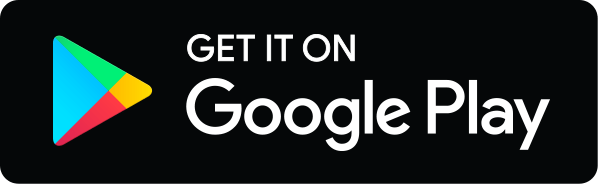