Fig. 1.
Perfusion deficits after SAH: (a) Representative coronal brain sections from FITC-dextran injected animals sacrificed at 3 h after sham or SAH surgery. FITC-dextran was circulated for 10 s only. Note uniform labeling of vessels with FITC-dextran in sham and partial, incomplete labeling in SAH animals. (b) Representative high magnification images of FITC-dextran labeling in right striatum after sham and SAH surgery. Note partial filled vessels in striatum of SAH animal. Also note the clear back ground. Scale: 20 μm.
2.2.2 Time of Tracer Circulation
Time requirement for filling a perfused and nonperfused vessel is different. Given enough time both vessels will be filled, however, a perfuse vessel will be filled before the nonperfuse vessel. Hence, intravascular tracer injection technique can only distinguish between perfused and nonperfused vessels if the time allowed for tracer circulation is short enough to allow this distinction to be achieved. We have found that 10 s circulation is plenty to achieve this distinction (Fig. 1) (17).
2.2.3 Brain Processing
Fixing the tracer injected brain so that tracer does not leak out of the vessels can be challenging. In addition, if fixation is not complete tracer leakage can also occur during brain sectioning and give faulty results. Perfusion fixation is not an option as this procedure will clear the tracer out instead of fixing it within the vessel. Snap freezing works well but can cause tracer diffusion during sectioning which appears as small fluorescent streaks around the vessels. We and others have found that immersion fixation is the best way to preserve the tracer in a vessel (see Procedures) (17, 21). Moreover, since immersion fixation is performed in 4% paraformaldehyde with increasing sucrose (10, 20, and 30%) gradients, ice crystals that can form during freezing process and damage the tissue are avoided.
2.2.4 Alternative Methods for Examining Perfusion Deficits
2.3 Material and Instruments
2.4 FITC-Dextran Solution
To prepare the FITC-Dextran solution the followings are needed:
1.
Normal saline.
2.
FITC-dextran powder (Sigma, St. Louis, MO).
In addition, to fix the tracer in the vessels following solutions are needed:
3.
4% Paraformaldehyde.
4.
4% Paraformaldehyde + 10% sucrose solution.
5.
4% Paraformaldehyde + 20% sucrose solution.
6.
4% Paraformaldehyde + 30% sucrose solution.
2.5 Brain Sectioning Instruments
To perform the sectioning, following equipment is required:
1.
Cryostat (Micron HM505 E).
2.
Knife K (16CM, C Profile Steel).
2.6 Photography and Processing Tools
1.
Fluorescence or confocal microscope (Ziess).
2.
Image analysis softwares: MetaMorph (Molecular Devices, CA, USA), IPLab (Signal Analytics, USA).
3.
A statistical program.
3 Procedures
3.1 Preparation of FITC-Dextran
1.
Dissolve FITC-Dextran in normal saline at a concentration of 50 mg/ml.
2.
Store in the dark till use.
3.2 FITC-Dextran Injection
1.
Anesthetize the animal (Ketamine–Xylazine; 80 mg/10 mg; Sigma).
2.
Catheterize (Intrmedic-PE-50) femoral artery.
3.
Inject 500 μl of 50 mg/ml FITC-dextran.
4.
Sacrificed the animal 10 s later.
3.3 Brain Processing
1.
Remove whole brain from the skull and immerse it in cold 4% PAF solution, incubate overnight at 4°C.
2.
Continue immersion fixation with increasing sucrose (10, 20, and 30%) gradients each performed over night at 4°C.
3.
Embed the brain in Tissue-Tek OCT (Miles, Elkhart, IN), and freeze in 2-methylbutane (Sigma) cooled in dry ice.
4.
Store the brain at −70°C till use.
3.4 Brain Sectioning
1.
Cut serial coronal brain sections (8 or 20 μm) thick using cryostat.
2.
Thaw-mount onto microscope slide (Colorfrost Plus; Fisher, USA) one section every 1 mm starting; bregma at +2.7 to −6.7.
3.
Fix sections on to the slide with chilled PAF 4% for 5 min.
4.
Wash with PBS for 5 min.
5.
Mount using aqueous mounting medium (Vector, Burlingame, CA, USA) and cover slip.
3.5 Imaging
1.
Examine FITC-dextran labeling in sections via fluorescence microscope.
2.
Take continual images of the whole coronal sections using a fluorescence microscope equipped with automated stage and camera.
3.
Alternatively, separate images (at least three per area) of striatum, basal and frontal cortex per right and left hemisphere can be taken.
3.6 Image Analysis
1.
Whole coronal sections: a montage of images showing the whole coronal sections is created using MetaMorph (Molecular Devices) and used for analyses (Fig. 1a).
3.
For analysis, in IPLab software (MetaMorph can be used for this purpose as well).
1.
Select whole image as ROI.
2.
Segment the image by intensity to select tracer-labeled vessels.
3.
Determine the number, total area, and area fraction of select vessels.
4.
Export the data to excel for statistical analysis.
3.7 Presentation and Statistical Analysis
2.
Comparison is made between the results from SAH and the time matched sham-operated animals.
3.
Data is analyzed via two-way ANOVA followed by a pair wise comparison by a suitable (such as Fisher’s PLSD) post-hoc test.
4 Cerebral Microdialysis
4.1 Introduction
Cerebral microdialysis is a sampling technique that measures extracellular concentration of substances by means of a small probe equipped with a semipermeable membrane inserted into the brain. A carrier solution (physiological solution called perfusate) is continuously circulating in the probe and substances present in the interstitial space diffuse into the perfusate making its chemical composition similar to that of the cerebral environment. As the solution exits the probe (now called dialysate), it is collected and analyzed for its components.
This technique is often used to detect cerebral ischemia in animals (23, 26–30) and in humans (31–34), after SAH. It does so by measuring the markers of glucose metabolism (energy-related metabolites) and of excitotoxicity. Since during ischemia there is an increase in anaerobic glucose metabolism (glycolysis) leading to rise in lactate and fall in pyruvate and glucose, the concentration of these metabolites in dialysate is used to detect ischemic cerebral environment. In addition, lactate to pyruvate ratio (L/P) and lactate to glucose (L/G) ratios are used to reflect the intracellular redox state, and hypoxic/ischemic glycolysis; respectively. Glutamate rise is considered an index of cerebral ischemia and excitotoxicity; especially in animals. Table 1 lists normal concentration of energy-related cerebral metabolites in rat.
Analyte | Concentration |
---|---|
Glucose (mM) | 2–3 |
Pyruvate (μM) | 8–15 |
Lactate (mM) | 0.6–0.8 |
Lactate:pyruvate ratio | 15–30 |
Glutamate (μM) | 2–6 |
Using this technique, in animals, decrease in cerebral glucose, increased in lactate/pyruvate ratio and glutamate is noted within minutes after SAH (Fig. 2) (23, 26). In patients, increased cerebral lactate/pyruvate ratio, and glutamate is found 24–48 h after SAH (31–34).
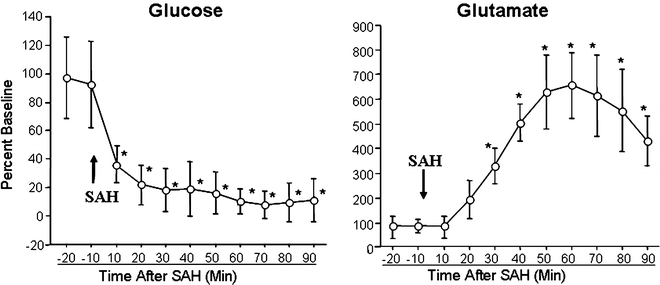
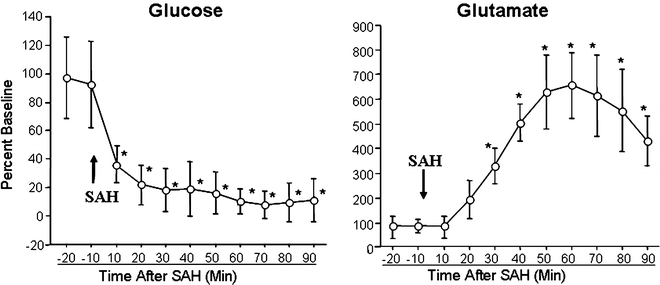
Fig. 2.
Changes in cerebral environment during the after 90 min after SAH. Cerebral glucose concentration falls and glutamate concentration increases minutes after SAH. Data is presented as percent baseline; mean ± SEM, n = 6, *P < 0.05.
Advantages of cerebral microdialysis are: (1) it is a simple way of sampling cerebral environment, (2) it does not require removal of body fluids and hence allows repeated sampling on same animal. Thereby, animal requirement is decreased and measurements are not tainted by changes in fluid balance or disturbance in blood homeostasis, (3) it allows sampling in anesthetize and in awake freely moving animals (chronic sampling), and (4) since dialysate does not contain proteins a protein clean up or protection of sample against ex vivo enzymatic degradation is not required.
Disadvantages of this technique are that: (1) probe implantation procedure is invasive and may cause injury to the brain tissue, (2) depending upon the method used, detection of dialysate can be costly, (3) it provides relative concentration of substrate and to calculate true concentration in vivo recovery of substrate need to be determined, and (4) sensitive analytical method for detection is required for detection of substance that are present is in small quantity.
4.2 Main Considerations
4.2.1 Choice of Probe and Membrane
Since probe is implanted into the brain stereotactically, a stiff probe is a better choice then a flexible probe. Selection of probe membrane depends upon the molecular weight and permeability to substance of interest. Probe membranes are made up of cellulose, copolymers, acrylic copolymers, or polysulfone. Typically, membranes with a pore size that allows diffusion of water soluble molecules with a cut off weight of 5–50 kDa are used for cerebral microdialysis.
4.2.2 Perfusate (Composition, pH and Temperature)
The movement of substances across microdialysis probe membrane relies on the concentration gradient between the interstitial fluid and the perfusate. Hence, it is important that the composition of perfusate mimics the fluid being sampled. A number of physiological salt solutions, including Krebs–Ringer solution and artificial cerebral spinal fluid (CSF) have been used for cerebral microdialysis (27, 29).
The temperature is also an important consideration as circulation of perfusate at room temperature will create temperature gradients across the probe that may modify immediate environment. Hence, prewarming of perfusate to body temperature before use is recommended (36).
4.2.3 Flow Rate and Sampling Interval
Flow rate and sampling interval are determined by the sample size required for analysis and the temporal resolution of molecules being sampled. Flow rate is directly proportional to recovery; the slower the flow rate greater the recovery. For cerebral microdialysis, perfusion flow-rate typically ranges from 0.1 to 5 μl/min (Table 1) (27–30).
4.2.4 Relative Recovery
The data obtained from microdialysis measurements is semiquantitative and is expressed as relative recovery. The term relative recovery is defined as the ratio between substance concentrations outside the probe to the concentration in the dialysate (37). Recovery is influenced by the size of the membrane pore size, membrane area, flow rate (a longer membrane and a lower flow rate will give a higher recovery) and diffusion speed of the substance (38). Recovery of each substance should be measured in vitro and mentioned when presenting data (see Methods).
< div class='tao-gold-member'>
Only gold members can continue reading. Log In or Register a > to continue
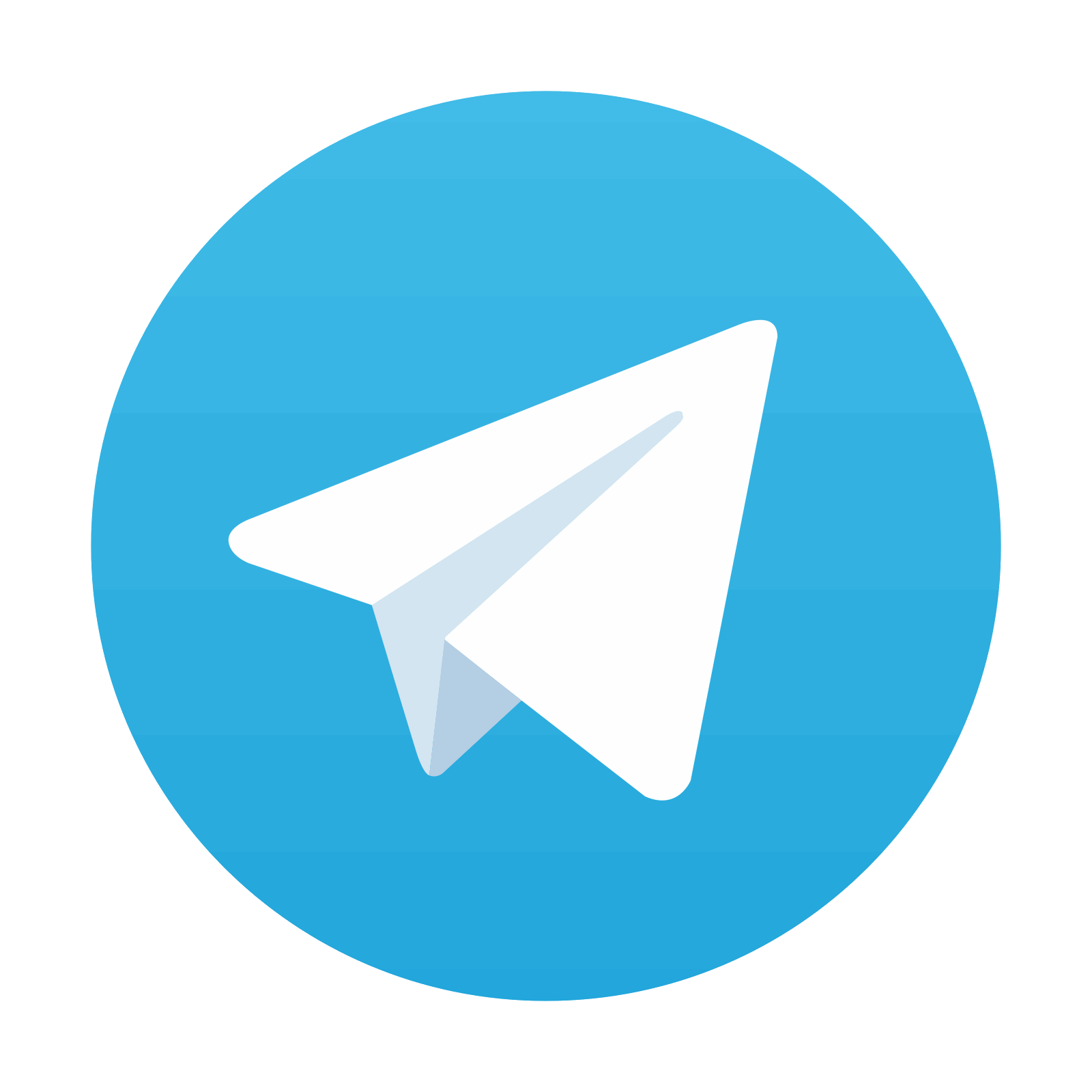
Stay updated, free articles. Join our Telegram channel

Full access? Get Clinical Tree
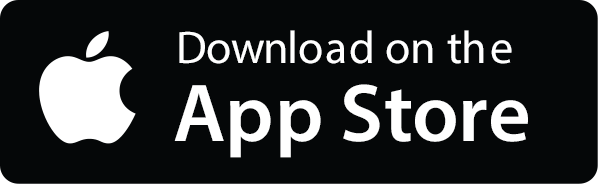
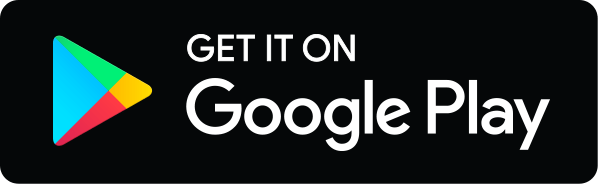