MICHAEL MENDL AND ELIZABETH S. PAUL Bristol Veterinary School, University of Bristol, Bristol, UK What is it to be mentally healthy and well? We all know that many aspects of our lives can impinge on our mental health including the ups and downs of our daily existence, how well or otherwise we are getting on with friends and family, how much control we have over our choices, and how well we function, both cognitively and socially. In this book, a similar range of influences on nonhuman animal (hereafter animal) mental health and well-being are identified; the extent of control that an animal has, the quality of its social relationships, the opportunities it has to express a wide behavioral repertoire and to choose how to allocate its time, and the degree to which it is prey to apparent disorders of cognitive function such as compulsions, hyperaggression, and forgetfulness. One potential consequence of this perspective is that in order to comprehensively assess mental health and well-being, we need to monitor the status of each individual with respect to all these factors. How supportive are their social relationships? Do they show symptoms of cognitive and behavioral disorders? Do they have outlets that allow expression of different needs and desires? However, a different view, also articulated in this book, is that what ultimately really matters to the animal is how it feels – whether it is in a positive or negative emotional or affective state. Thus, a hyperaggressive sow in a group who is able to access resources freely due to her unusually despotic style may actually be in a relatively positive affective state. Likewise, a stereotyping animal may be in a more positive state than a nonstereotyper in the same captive environment (Mason and Latham, 2004). A similar discussion is evident between psychological theorists studying human quality of life. Norman Bradburn’s (1969) seminal research viewed the balance between positive and negative affect as the critical determinant of how well a person felt. However, more recent theories propose that a range of other factors including ‘accomplishment’, ‘positive relationships’, ‘engagement’, ‘environmental mastery’, ‘life satisfaction’, and ‘meaning and purpose’ are also important determinants of well-being (Diener et al., 1999; Ryff and Singer, 2008; Seligman, 2011; Franks and Higgins, 2012). One possibility that unites both views is that it is the impact these factors have on an individual’s emotional state that is key (Fig. 23.1). Affective state may be thought of as a ‘common currency’ (McNamara and Houston, 1986; Cabanac, 1992) which integrates the influence of a range of experiences and provides the best indication of an individual’s current well-being. A semantic resolution might consider ‘well-being’ as principally determined by affective state, while ‘mental health’ is also affected by cognitive (dys)function including, for example, cognitive or behavioral disorders that are not necessarily linked to well-being in a predictable way. The purpose of this brief discussion is to illustrate that assessment of animal mental health and well-being is conceptually as well as technically challenging, but also that the affective state of the animal will be one of its key determinants. Accurate assessment of emotional or affective state therefore provides a foundation for scientific investigations into the mental health and well-being of animals and is the focus of the rest of this chapter. We begin by discussing what emotion actually is and the inherent challenges of studying a phenomenon that has its roots in human conscious experience. We then briefly describe two ways of conceptualizing emotion in humans and the pros and cons of translating these to animals. At this point, we clarify the animal emotion terminology that we will employ in the rest of the chapter, and then discuss ways in which we can infer the occurrence of different affective states in animals, including the use of theoretical and operational definitions that can provide a framework for studying animal affect. In the second half of the chapter, we go on to summarize a variety of methods that have been used to assess animal affect, focusing in particular on new developments in the use of expressive behavior and cognitive markers. Our concept of animal emotion inevitably stems from our own experience of emotions – feelings that we label with terms such as ‘happy’, ‘serene’, ‘anxious’, ‘embarrassed’, ‘guilty’, ‘angry’, and so on. Emotions are therefore a category of subjective mental experience, the key defining characteristic of which is that we experience them as positive or negative, pleasant or unpleasant, rewarding or punishing – they have ‘valence’. Transferring this concept to other species is of course tricky and open to the accusation of uncritical anthropomorphism. We cannot know for certain what private mental states, including emotions, another individual is experiencing, or even whether they are capable of having such subjective experiences (see ‘Problem of other minds’ [Farah, 2008]). When considering other people, language helps us to tackle this obstacle (although people can of course lie), but this option is not available for nonhuman animals. So, can we proceed beyond this potential impasse to study animal emotions? One approach is to take an alternative philosophical stance, and this has been followed by Wemelsfelder (1997), who argues that animal subjectivity can be revealed to an observer through the expressive quality of the animal’s behavior and hence can be studied directly. Another more commonly agreed upon approach is to accept that mental experiences are not directly accessible in other species, but that evidence from behavioral, cognitive, and neurophysiological studies is sufficient to argue that at least some species are highly likely to be consciously aware (Griffin and Speck, 2004). The 2012 Cambridge Declaration on Consciousness by consciousness researchers and neuroscientists (Low, 2012) is a good example in which the signatories argue, in contrast to others (Macphail, 1998; Key, 2016), that possession of a neocortex is unlikely to be necessary for conscious experience, and that all mammals and birds, and some other species such as octopuses, possess the neural substrates of consciousness, show the capacity for intentional behaviors, and hence are capable of conscious experience. If we accept that certain taxa and species have the capacity for conscious experience – and there are differing views as to the range of species that should be included (Panksepp, 1998; Rolls, 2005; Barron and Klein, 2016; Key, 2016) – we next need to develop measures that can give us an insight into what emotional state an animal may be experiencing at any one time (the contents of its putative conscious experience). This requires us to become more specific about what we mean by emotion. Human psychologists have adopted two main approaches to conceptualizing emotion. The discrete emotions approach reflects our usage of ‘emotion words’ such as ‘sad’, ‘happy’, ‘angry’, and so on, by arguing that these words describe the subjective experience of distinct emotional states that are instantiated in the activity of specific neural circuits in the brain. Advocates argue that there are a limited number of such discrete emotion systems and that these form the building blocks for all emotional experience. Although researchers vary in their beliefs about how many and which basic emotional states exist (Ekman, 1992; Plutchik, 2001; Izard, 2007), they concur that each state is grounded in the functioning of a modular neurobehavioral system that is activated by particular stimuli and generates behavioral changes such as specific facial expressions, and a distinctive profile of physiological responses. An alternative dimensional view of human emotion stems from studies that use factor analysis to analyze the clustering in time of reported emotional experiences, or the categorization of discrete emotion words. Most studies detect two or three underlying dimensions that account for much of the variation in the data. Valence (positivity or negativity of the experience) is one of these dimensions and, as mentioned above, can be thought of as the defining characteristic of subjective emotions (Cacioppo and Berntson, 1999; Watson et al., 1999; Carver, 2001; Russell, 2003). Another commonly detected dimension is arousal, the degree of activation experienced. Both these dimensions characterize the prominent core affect model of emotion (Russell, 2003; Fig. 23.2). Both models of human emotion have their supporters. They can be combined by, for example, visualizing discrete emotions as being located in dimensional space. For example, ‘sadness’ would lie in the low arousal/negative valence quadrant of the core affect model (Q3 in Fig. 23.2). However, ongoing arguments contest the primacy of each view. For example, do core affect processes combine with other information about current context and past experience to construct the experience of an emotion that falls in the ‘sad’ category, or is ‘sadness’ a basic discrete emotion that contributes to a ‘higher level’ abstraction of overall valence and arousal? Whether one set of processes gives rise to the other, or vice versa, or both influence each other, remain the subject of vigorous debate (Izard, 2007; Panksepp, 2007; Adolphs, 2017; Barrett, 2017). For example, reliable co-occurrence of particular reported discrete emotions and specific behavioral, physiological, and neural changes is found in some reviews of the literature (Kreibig, 2010; Vytal and Hamann, 2010; Kragel and Labar, 2016), but others suggest that such changes map more closely to dimensional concepts (Mauss and Robinson, 2009; Lindquist et al., 2012). In terms of translating these ideas to animals, our view is that the dimensional core affect model has some advantages. One reason is that the discrete emotions approach relies on the use of human emotion words. This is problematic because these words are not universal across human cultures (e.g., age-otori – the Japanese feeling of having had a bad haircut), hence questioning the wisdom and validity of generalizing them across species. While it is plausible that, for example, fear-like states are widespread across the animal kingdom, as species’ sensory, perceptual, and cognitive abilities become increasingly different from ours, and their evolved behavioral repertoires also diverge, the chances that they will share other discrete emotions are likely to diminish. Second, human emotion words inevitably imply felt subjective states whereas, as we have discussed, we cannot be sure that such states are consciously experienced in other species. Consequently, in a comparative context, the use of discrete emotion words like ‘happiness’, ‘sadness’, and especially terms such as ‘jealousy’ or ‘guilt’, becomes increasingly questionable as we move away from humans along the phylogenetic tree. The dimensional core affect model avoids some of these problems by identifying four simple states that are not founded on human emotion words (Q1–4 in Fig. 23.2), and which can be readily translated to other species. Although this might seem too simple a perspective from which to summarize the emotional lives of at least some other species, the four states can be viewed as the source from which a potentially wide range of affective states can be constructed in combination with other contextual information (Barrett, 2017). Such states will be influenced by species’ sensory, perceptual, and cognitive capacities and hence species-unique and not always easily captured by human emotion words (Bliss-Moreau, 2017). Furthermore, the core affect model provides a framework for conceptualizing how different types of emotional states are related to each other (e.g., joy and contentment may primarily differ in arousal), while the discrete emotions approach treats individual emotional states separately and in a piecemeal fashion. Finally, as we will describe below, the core affect approach also fits closely with a simple operational definition of animal emotion that avoids the complicating issue of conscious experience and provides a behavioral grounding that makes studying animal affect empirically tractable. Although we personally favor the dimensional perspective, we will consider both views in the rest of this chapter. Given the above discussion, we propose the following use of terms. We will use ‘animal emotion’ and ‘animal affect’ as umbrella terms for the general area of study. Because ‘emotion’ and emotion words (‘happy’, ‘sad’, ‘angry’, etc.) are often assumed to imply conscious feelings, we add the suffix ‘-like’ when using these to indicate that we cannot be certain of whether they are subjectively experienced in other species (cf. ‘episodic-like memory’ in animals [Clayton and Dickinson, 1998]). We also restrict their use to short-term states generated by specific stimuli or events, as is often the case in human psychology research (Russell, 2003; Scherer, 2005). Similarly, we add the ‘-like’ suffix when we use the word ‘mood’, which we reserve for longer-term states that are ‘free-floating’ and not caused by a specific object or event. We use ‘affect’ or ‘affective state’ as overarching terms for both short- and long-term valanced states. These therefore cover emotions and moods, but also sensations (e.g., pain) that have a valanced component. Although affective states are usually considered to be consciously experienced in humans, the technical as opposed to colloquial provenance of the term ‘affect’ means that it is less likely to be assumed to imply conscious experience in animals. For ease of use, we therefore employ this term without a ‘-like’ suffix, but also without any implication that it denotes a conscious experience. Finally, we use ‘feelings’ only when referring specifically to the felt component of an affective state. Having laid the groundwork, we now consider how we might measure the concepts that we have discussed. In doing so, we follow the componential view of emotion espoused in human psychology that affective states comprise subjective, behavioral, physiological, and neural ‘components’ (Paul et al., 2005; Scherer, 2005). While we cannot directly assess the subjective component, we can objectively measure the others. The challenge is to establish which changes in these components are reliable indicators of which discrete or dimensional affective states. This requires an inferential or theoretical framework and we briefly identify three inter-related approaches: translating human affective responses to animals, inferring affect from behavior (responses) or situations (events), and using prescriptive theories or definitions (Paul and Mendl, 2018). A similar schema is discussed and developed in more detail by Mason and Mendl (2019, manuscript in preparation). Irrespective of the details of the schema employed, the main point here is that a rigorous science of animal emotion requires clearly stated theory and/or rationale for exactly how emotion-like states in animals are to be inferred. A ‘translation from humans’ perspective involves identifying the behavioral, physiological, and neural indicators of specific reported emotional states in humans and then monitoring these biological markers in animals under the assumption that they are associated with the same affective states. The advantage of this approach is that people provide our best model of how objectively measurable indicators co-vary with subjectively experienced feelings. The disadvantages, as discussed earlier, include that there is still debate as to whether there actually are repeatable and reliable indicator profiles for specific discrete emotions or emotional dimensions in humans, and that other species may not share the same discrete emotion-like states with humans. An alternative approach is to infer affective states from the animal’s behavior or the situation that it is in (Paul et al., 2005). An example of ‘behavioral inference’ is the common assumption that playing animals are in a ‘happy’, ‘joyful’, or otherwise positive affective state. However, the links between play and positive affect are not always straightforward or well understood (Held and Spinka, 2011; Ahloy-Dallaire et al., 2018). An example of ‘situation inference’ is the notion that exposure to a brightly lit open field or the unprotected arms of an elevated plus maze induces ‘fear-like’ or ‘anxiety-like’ states in burrow-dwelling nocturnal rodents. This can be reasonably supported by arguments based on knowledge of the biology of these species, but our anthropocentric perspective makes us prone to errors. For example, we may be unaware that a certain olfactory stimulus generates a ‘pleasure-like’ state in our animals and, consequently, misinterpret or misattribute behavior in situations where the odor is present. Overall, the utility and validity of these, often intuitive, inferences are likely to depend on how well we know the biology and behavioral ecology of our animals, and on the quality of our argument for why a particular set of circumstances is likely to lead to a particular emotion-like state. Increasing phylogenetic distance between ourselves and our study species is likely to diminish the accuracy of our inferences. Proposing a prescriptive theoretical framework or operational definition can provide an explicit rationale for our behavioral or situation inferences about animal affect (Paul and Mendl, 2018). One example is Wemelsfelder’s (1997) argument that animal subjectivity, including affective states, can be directly observed in the expressive behavior of animals. This provides a basis for behavioral inference grounded in a particular philosophical theory. Another example is the ‘cognitive bias’ approach that translates the relationship observed between human affective valence and cognitive function to animals and combines it with arguments from evolutionary theory to generate a rationale for inferring affective valence from decision-making behavior (Mendl et al., 2009, 2010b; see later). A third example translates human appraisal theory ideas which posit that particular discrete emotions result when a person appraises a stimulus or event in a specific way. For example, fear arises if a situation is appraised as sudden and of low predictability, pleasantness, or familiarity (Scherer, 2001). Desiré et al. (2002) proposed that a fear-like state would therefore occur in animals exposed to sudden, unpredictable, unpleasant, and unfamiliar events, with other discrete emotion-like states occurring in response to other event profiles based on appraisal theory (Veissier et al., 2009). If one accepts translation of the links between stimulus appraisal profiles and human discrete emotions to animals, this provides a theoretical grounding for situation inference of discrete emotion-like states. Finally, reinforcement theory concepts of emotion (Millenson, 1967; Gray, 1987; Rolls, 2005) offer an operational definition of emotion which has been articulated by Rolls (2005, p. 72): ‘emotions are states elicited by rewards and punishers’, where rewards are things that animals will work to acquire and punishers are things that they will work to avoid. This prescriptive definition allows researchers to infer that an animal is in a positive state if exposed to situations that it will work to acquire, and in a negative state if exposed to situations that it will work to avoid. It thus provides a theoretical basis for situation inference of affective valence which sidesteps the question of whether the identified affective states are consciously experienced and hence has clear value in the study of animal emotion. Strengths and limitations of this definition are discussed by Paul and Mendl (2018), alongside prescriptive definitions of animal emotion in terms of ‘emotion primitives’ or emotional building blocks (Anderson and Adolphs, 2014), and states that mediate goal-directed learning (Dickinson and Balleine, 2009). We believe that clear and logical arguments for how we infer discrete emotion-like states or affective valence in animals are essential for development of a rigorous science of animal emotion. This may best be achieved by combining theoretical frameworks with translation of findings from human studies to generate operational definitions and/or a clear rationale for why particular behaviors or situations indicate particular affective states. Scientists in a range of disciplines including neuroscience, psychopharmacology, drug development, and animal welfare science have been using a variety of indicators to assess animal affect for many decades. Prominent among these are measures of stress physiology (Mormede et al., 2007; von Borell et al., 2007; Palme, 2012), reflecting the long-standing notion that bodily physiological changes are intimately involved in human emotional experience (James, 1884; Critchley et al., 2004). Behavior in standardized testing paradigms such as the open field, elevated plus maze, startle, novel object, sucrose consumption, tail-suspension, and forced-swim tests (Pellow et al., 1985; Ramos and Mormede, 1997; Cryan and Mombereau, 2004; Forkman et al., 2007), and neural markers of affective processes involved in, for example, fear-conditioning, and responses to reward (LeDoux, 1996; Panksepp, 1998; Rolls, 2005) have also been used. Because there are many excellent reviews of these ‘traditional’ measures, including those cited above, we focus on approaches that have been developed more recently. These have emerged partly because of limitations of existing indicators, including that physiological stress responses are better indicators of arousal than affective valence (Paul et al., 2005; Buwalda et al., 2012), behavioral tests developed for rodents often have limited relevance in other species due to differences in species’ behavioral biology (Paul et al., 2005), and the invasive techniques needed to tap the undoubted potential of neural markers are often not appropriate in animal emotion studies. A number of the new measures considered here have been conceived by animal welfare scientists with a specific interest in animal well-being, including the neglected area of positive affect, and hence are particularly relevant for this book. We identify two main categories – expressive behavior and cognitive markers of affective valence – and briefly consider the rationale underpinning these measures and their pros and cons to illustrate the exciting work that is going on in this field. In his book The Expression of the Emotions in Man and Animals, Charles Darwin (1872) explored the idea that discrete emotion-like states are expressed behaviorally through postures, vocalizations, and movements. His ‘principle of serviceable habits’ posited that expressions reflect adaptive movements associated with the emotional state; for example, opening eyes wide to gather more information when fearful, or closing the nostrils to avoid aversive stimuli in disgust (Susskind et al., 2008). If discrete emotions have particular adaptive functions that can be facilitated by behavioral expression, then links between affect and expression may occur across species. In humans, the psychologist Paul Ekman (1992) argued that there were specific human facial expressions reflecting discrete emotions such as happiness, sadness, fear, disgust, anger, and surprise, and that these were conserved across countries and cultures. In animals, there has been increasing interest in the utility of expressive behavior as a marker of affective state. Here we briefly consider facial expressions, vocalizations, and whole-body expressive behavior. Facial expressions have been studied in nonhuman primates (hereafter primates) for many years, often using a ‘situation inference’ rationale. In seminal studies, van Hoof (1976) observed that the primate bared teeth ‘grin face’ often occurred when animals were under threat and appeared to communicate a submissive state. The open-mouthed ‘play face’, on the other hand, was expressed during bouts of play fighting and hence might function to communicate nonserious intent. van Hoof argued that human smiling and laughter evolved from these expressions, raising the possibility that the ancestral displays might also be linked to emotional experience. Objective methods for recording and categorizing primate expressions, based on Ekman et al.’s (2002) human facial action coding system (FACS), have allowed systematic description of the precise shifts in facial musculature that occur in particular contexts, providing solid groundwork for further studies of the emotional, communicative, and functional significance of different expressions (Parr et al., 2007; Waller et al., 2012; Caeiro et al., 2013). For example, a chimpanzee FACS was used to demonstrate that the same facial movements occur during both primate play faces and human laughter (Parr et al., 2007), just as van Hoof argued and supporting a ‘translation from humans’ argument. Further research has investigated whether these expressions may be linked to emotional states. Parr (2001) demonstrated that chimpanzees were able to match the positivity or negativity of a scenario (e.g., image of hypodermic needle or favorite food) to images of facial expressions assumed to share the same emotional valence (e.g., bared teeth ‘grin face’ or open mouth ‘play face’). Such findings support the idea that primate facial expressions may be useful indicators of affective valence, although Waller et al. (2017) caution against assuming that primate facial expressions have evolved solely to reflect current emotion-like states which can be ‘read’ by others. The use of facial expressions as indicators of affective states now extends to nonprimate species. A particularly active area of research has focused on assessing facial expressions as indicators of pain, including in mice (Langford et al., 2010), rats (Sotocinal et al., 2011), rabbits (Keating et al., 2012), horses (Dalla Costa et al., 2018), cattle (Gleerup et al., 2015), and sheep (McLennan et al., 2016). Most of the resulting ‘grimace scales’ are based on a ‘situation inference’ rationale linking facial changes to, for example, naturally occurring conditions assumed to be painful, postoperative recovery, and the presence or absence of analgesia. Some of the observed facial changes (e.g., orbital tightening) occur in putatively painful situations in several species including humans, suggesting that they may be conserved across mammalian taxa. Facial changes in other situations also show similarities across species. For example, Berridge and Robinson (2003) argued that expressions of gustatory ‘liking’ (e.g., tongue protrusion, lip-licking) and disgust (e.g., mouth gaping) are conserved across humans, primates, and rodents. Similarly, orbital widening appears to occur in threatening or otherwise aversive situations in species including humans (Susskind et al., 2008), sheep (Reefmann et al., 2009), and cattle (Sandem et al., 2006), and open mouth ‘play faces’ are observed in dogs (Palagi and Scopa, 2017) and other carnivores (Poole, 1978) as well as primates and humans. Cross-species similarity of expressions suggests that they may have adaptive value, for example in detecting danger (Susskind et al., 2008), and this allows theoretical arguments to be built for why particular expressions should be associated with particular affective states. Facial expressions thus hold considerable promise as indicators of animal affect. Many questions remain to be resolved including whether specific expressions map to particular discrete emotion-like states and/or can be used as markers of affective valence, whether they reliably co-occur with such states, are sometimes not expressed, are expressed in other contexts in the absence of such states, whether they reflect longer-term as well as short-term affect, and whether they can tell us anything about emotional feelings in other species. In terms of this last question, Langford et al. (2010) showed that mice with damage to the rostral anterior insula, an area of the brain implicated in human conscious experience of the affective unpleasantness of pain, showed attenuated grimace expressions but continued to display other behaviors such as abdominal constriction that also co-occur with assumed pain states. One speculative interpretation of these findings is that facial expressions are more than just reflex behavioral responses to nociceptive stimulation, and instead reflect an affective and even conscious pain state. ‘Situation inference’ approaches have been used to investigate whether vocalizations reflect animal affective states. For example, rats emit characteristic 22 kHz calls in aversive contexts such as social defeat and exposure to noxious stimuli, while they produce 50 kHz calls in response to rewarding stimuli including social play, sex, and manual ‘tickling’ by a human for which individual-housed animals in particular will learn to work (Panksepp and Burgdorf, 2000). Clear avoidance of and preference for these contexts accords with the operational definition of affective valence described earlier and hence provides a theoretical basis for these inferences from situation. It is therefore plausible that these ultrasonic vocalizations are reliable indicators of negatively and positively valanced affect, respectively, although there appear to be some exceptions, for example the production of 50 kHz calls during aggressive interactions. The notion that vocalizations reflect affective state is also supported by studies of audible calls in a variety of mammalian species. In a review of research in which affect was principally inferred from situation, Briefer (2012) concluded that vocal features such as elevated call rate, fundamental frequency range, and peak frequency were reliable cross-species indicators of high affective arousal. However, due to a relative lack of study, there was less evidence for any conserved vocal markers of affective valence. Recent studies of pigs have detected a tendency for animals exposed to aversive situations such as castration and isolation to emit higher frequency calls (Weary et al., 1997; Puppe et al., 2005), particularly calls that are modulated (Tallet et al., 2013), than pigs in more positive situations. However, there may be a nonlinear link between affective valence and call characteristics in this species, with high frequency vocalizations occurring in both strongly positive and negative states and lower frequency calls in less intense states (Tallet et al., 2013). Further research is required to determine whether there are universal vocal markers of affective valence in mammalian species, or whether calls are more context, and perhaps emotion, specific as suggested by Dupjan et al. (2008), who showed that pig call structure changed in response to the same aversive stimulus depending on whether it was anticipated or unexpected. The complexity and flexibility of vocal expressions, coupled with between-species differences in vocal tract morphology, make it challenging to establish simple rules for the relationship between affect and calls. Nevertheless, vocalizations remain a very promising topic for research into behavioral expressions of animal affect (Briefer, 2012). Wemelsfelder (1997), along with her colleagues, pioneered the development of a method (QBA) for assessing whole-body expressive behavior in animals. Human observers watch the animal and either rate it against specific affective descriptors such as ‘happy’, ‘calm’, ‘bored’, ‘fearful’, and ‘frustrated’ on a visual analog scale (Camerlink et al., 2016), or generate their own terms to describe the animal’s behavior (Wemelsfelder et al., 2001). Analysis of the resulting ratings often generates factor dimensions or consensus axes that show similarities to core affect valence and arousal dimensions (Camerlink et al., 2016; Minero et al., 2016). Numerous studies on a range of mammalian species including pigs, sheep, cows, horses, goats, dogs, and elephants have indicated good interobserver agreement in ratings of specific affective descriptors, even when observers have different backgrounds (Wemelsfelder and Mullen, 2014; though see Bokkers et al., 2012). Evidence from studies relying on ‘situation inference’ and investigating links between QBA and other affect indicators supports the notion that QBA can pick up on behavioral changes that reflect affective states. For example, Hintze et al. (2017) adopted a ‘situation inference’ rationale using grooming to induce an assumed positive state in horses, and a waved plastic bag to induce an assumed negative state. They found higher ‘calm’ and ‘relaxed’ observer ratings in the former context and higher ‘stressed’ and ‘nervous’ ratings in the latter. Similarly, observers rated pigs that had been given an anxiolytic drug to be higher on an emergent dimension of ‘curiosity’ and ‘confidence’ than control animals (Rutherford et al., 2012). QBA has been adopted by welfare assurance scheme protocols (e.g., EU Welfare Quality®) as a practical indicator of animal affect. It remains to be seen whether this innovative approach can be extended to taxa whose expressive behavior humans may find more difficult to ‘read’ (e.g., reptiles, fish, crustacea). The study of expressive behaviors such as those discussed above and others including inactivity (Fureix and Meagher, 2015) and play (Held and Spinka, 2011) represents a growing area of animal affect research that has already yielded new markers of affective state. These are particularly valuable because they occur spontaneously and hence do not require specific testing situations to be imposed, can be recorded noninvasively, and offer opportunities for automated monitoring, which are already being developed (Schön et al., 2004; Sotocinal et al., 2011; Dalla Costa et al., 2018; Tuttle et al., 2018). One disadvantage of expressive behaviors is that many are likely to be species specific, making the search for generalities challenging and the translation of findings across species difficult. Cognitive markers are a new category of animal affect indicator that stem from empirical and theoretical links between affective states and objectively measurable cognitive functions such as decision making, anticipation, and attention that may hold across species and even across diverse taxa. Here we briefly consider anticipatory behavior, lateralized information processing, and cognitive biases. A ‘cognitive’ marker of affective valence based on a theoretical rationale has been proposed by Spruijt et al. (2001). The approach draws on ideas about the neuroscience of reward (Berridge and Robinson, 2003; Cabib and Puglisi-Allegra, 2012) and posits that relatively mild or short-term negative states can elevate an animal’s reward sensitivity. Spruijt et al. (2001) hypothesized that this increases an animal’s chances of reward acquisition and ‘re-balancing’ of state toward ‘neutral’ or positive, and can be detected by measuring ‘anticipatory behavior’ expressed during presentation of a Pavlovian cue that signals arrival of reward after a short time delay (e.g., 30 seconds). Animals in a short-term negative state are predicted to show enhanced ‘anticipatory behavior’ while the cue is present. ‘Anticipatory behavior’ is determined empirically and is quantified as an increase in activity and transitions between behaviors in rodents, horses, and chickens (van den Berg et al., 1999; Peters et al., 2012; Moe et al., 2013), but a decrease in these transitions in cats (van den Bos et al., 2003). Several studies indicate that induction of putatively negative short-term states do indeed appear to result in increased anticipatory behavior (van der Harst et al., 2003; van der Harst and Spruijt, 2007; Makowska and Weary, 2016). However, in longer-term negative states, there is evidence from some rodent studies (van den Berg et al., 1999; von Frijtag et al., 2000) that sensitivity to, and valuation of, reward decreases (cf. anhedonia) and hence anticipatory behavior in response to a Pavlovian cue actually reduces (van der Harst and Spruijt, 2007). This biphasic relationship between negative affect and anticipatory behavior means that using the approach as a measure of affective valence is best done when there is knowledge of the time course of the subject’s experiences. In addition, a reliable measure of anticipatory behavior needs to be determined for each species. Given this information, anticipatory behavior can be a useful indicator of affective valence. Understanding the role of anticipation in affect may also enable us to generate positive affective states or protect against negative ones. It has been proposed that signaling the arrival of reward activates the mesolimbic dopaminergic ‘reward system’ and that the consequent release of dopamine during the reward anticipation phase can act to induce a relatively positive affective state and to counteract depression-like states (van der Harst and Spruijt, 2007). For example, announcing food arrival or transfer to an enriched cage prevented the onset of depression-like anhedonic symptoms in rats subjected to social defeat, and appeared to be more effective than presenting the reward without announcement (van der Harst et al., 2005). In a study of pigs, cueing the arrival of enrichment was found to increase play in newly weaned pigs, and to decrease stress-related post-weaning behavior (Dudink et al., 2006). Empirical findings from human studies have generated a variety of hypotheses about potentially different functions of the left and right hemispheres of the brain, including emotional processes. One such hypothesis is that the right hemisphere plays a dominant role in processing negatively valanced, novel, or threatening events, while the left hemisphere is dominant in processing more positive events (‘emotional valence lateralization’) (Davidson and Irwin, 1999; Leliveld et al., 2013). Following a ‘translation from humans’ rationale, these ideas have been investigated in animals (Rogers, 2010; Leliveld et al., 2013). While direct recording of brain activity (e.g., using EEG or MRI) would be the gold standard measure of hemispheric functional lateralization, this is impractical in most contexts and instead researchers use proxy measures such as whether the animal turns to the left or right when inspecting a rewarding or aversive stimulus. Because signals from the left visual and auditory fields project contralaterally to the right hemisphere and vice versa, the prediction is that punishing events will be inspected and detected more readily in the left sensory field, and rewarding stimuli in the right sensory field. The opposite prediction is made for olfactory stimuli because neurons in the right and left nostrils project ipsilaterally to the right and left hemisphere, respectively. Leliveld et al. (2013) reviewed evidence from studies that inferred affective valence from contexts that involved threat (‘fear-like’), aggression (negative), or food, sex, and positive social interactions (positive). The work encompassed a range of taxa, including mammals, birds, reptiles, and fish, and used proxy measures of laterality. Support for the emotional valence lateralization hypothesis was found in ‘fear-like’, aggression, and food contexts. For example, lizards preferentially used their left eye when inspecting predators (Martin et al., 2010), dogs showed right-hemisphere bias by favoring use of the left visual field when faced with novel or threatening stimuli (Siniscalchi et al., 2010), and black winged stilts preferentially used their right eye to inspect food (Ventolini et al., 2005). However, there were exceptions to these findings and no clear lateralization was observed in the sex and positive social interaction contexts. As well as reflecting the affective impact of the current situation, lateralized brain activity or behavior has also been suggested to indicate an individual’s background affective state. For example, lower left relative to right frontal activity is characteristic of depressed human patients (Bruder et al., 2017). In animals, there is some evidence for similar associations. For example, right-handed marmosets, suggesting left-hemisphere dominance, were bolder in their response to novel food and showed shorter ‘fear-like’ freeze responses to a predator call (Braccini and Caine, 2009), perhaps indicating a more positive background affective state. Likewise, pigs showing consistent right biases in the use of their snouts and how their tails curl were bolder and more exploratory (Goursot et al., 2018). However, links between putative background affective state and laterality are not always observed (Kappel et al., 2017). Measures of lateralization as markers of affective valence have advantages that include not usually requiring any training of the animal and hence being potentially quick to assess. They can also be used in a range of vertebrate taxa, and potentially in any species with bilateral symmetry of brain structures. However, there is still uncertainty in both the human and animal literature as to the strength of the relationship between affective valence and lateralization, as evidenced by the range of hypotheses about the functions of brain asymmetry (Brancucci et al., 2009) and the increasing focus on functional networks of activity within the brain that involve communication between hemispheres and hence may not be inherently predisposed to lateralization (Shanahan, 2012). The rationale for the most well-used cognitive bias method for assessing animal affective valence – ‘judgment bias’ – is based on both ‘translation from humans’ and theoretical arguments. The former includes findings that people in negative states make more negative judgments (‘pessimistic’) about ambiguous events and situations than happier people (Mathews and MacLeod, 1994; Mineka et al., 1998; Paul et al., 2005). The latter include arguments that, following the reinforcement theory definition of animal affect described earlier, an animal’s affective valence reflects its experience of past rewarding or punishing events. Affective valence may also, therefore, act as a Bayesian prior for the likelihood of these events occurring in future; it makes adaptive sense for individuals who have experienced a preponderance of aversive events and hence are in a more negative state to also predict that such events are more likely to occur in future ambiguous situations, and hence to make negative judgments under ambiguity (Mendl et al., 2010b; Nettle and Bateson, 2012). Over 100 studies have been published using the judgment bias assay initially developed for rats by Harding et al. (2004; Fig. 23.3). Because the theoretical basis of the approach generates predictions that are expected to hold across taxa, studies have been performed in a wide range of species including mammals (e.g., rats, mice, sheep, dogs, pigs, horses, cattle, monkeys, humans), birds (e.g., starlings, chickens), and even insects (e.g., bumblebees, honeybees) – see reviews by Mendl et al. (2009), Gygax (2014), Baciadonna and McElligott (2015), Bethell (2015), and Roelofs et al. (2016). The majority of studies support the hypothesis that induced affective states are associated with the expected judgment bias, although some studies have null or even opposite results. Our ongoing meta-analyses are identifying this heterogeneity in findings, and seeking to evaluate the influence of different moderators on results.
23.1 Introduction: Mental Health, Well-being and Emotional States
23.2 What is Animal Emotion: Consciousness and Concepts
23.2.1 Emotions as conscious subjective experiences: a potential barrier to their study in animals?
23.2.2 Discrete emotions and the dimensional view of emotion
23.2.3 Translating discrete and dimensional concepts to animals
23.3 Animal Emotion Terminology
23.4 From Animal Emotion Concepts to Animal Emotion Assessment
23.5 Assessing Animal Affect: New Developments in the Use of Expressive Behavior and Cognitive Markers
23.5.1 Expressive behavior
Facial expressions
Vocalizations
Qualitative behavioural assessment (QBA)
Conclusions
23.5.2 Cognitive markers of affective valence
Anticipatory behavior
Lateralization
Cognitive bias
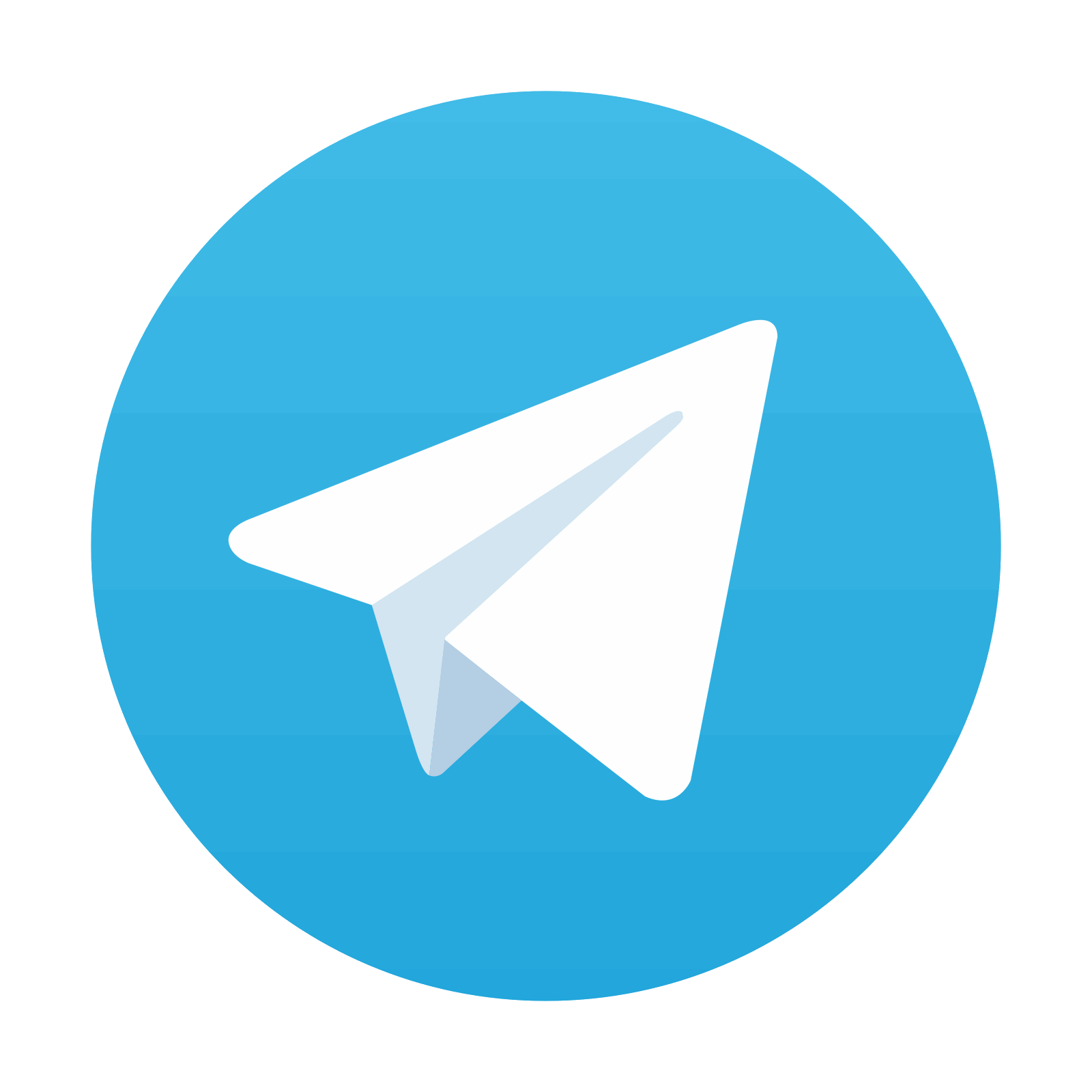
Stay updated, free articles. Join our Telegram channel

Full access? Get Clinical Tree
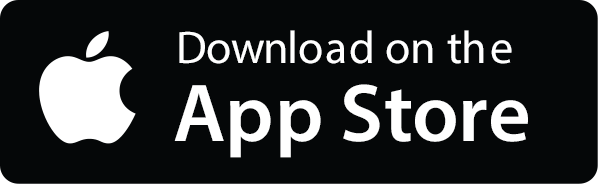
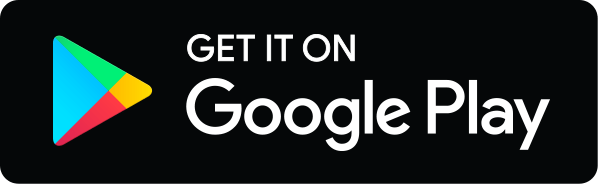