The speed with which these parameters may change depends on numerous factors including the ratio of fish biomass to water volume and the capacity of the filtration system. Therefore no single monitoring regime is appropriate for all systems2. As a minimum, in flow-through systems, measure temperature and dissolved oxygen at least once daily, with pH and ammonia checked at least weekly. In static systems measure temperature and dissolved oxygen daily, and other values depending on the frequency and amount of water exchange.
Feeding
Fish need relatively little food compared with mammals. Short periods of starvation appear not to be detrimental whereas overfeeding has many adverse effects. Different species have different requirements, and specialised texts should be consulted for detailed information. In general, first feeding, when the recently hatched fish have used their yolk sacs and are starting to feed independently, can be a critical period, and care must be taken to ensure appropriate foodstuffs are offered at this time.
Handling and Techniques
Handling is stressful for fishes, although they can become conditioned to handling to some extent. Even routine handling procedures may cause injury or even death if carried out incorrectly. Fishes should be handled only when necessary, and the number of handling episodes should be minimized. Appropriate handling equipment, such as a bucket or knot-free nets, should be used to minimize damage. Fish should be handled very gently, and kept moist at all times. They should always be placed on soft, non-abrasive, wet surfaces2.
Digestion requires energy and increases oxygen demand, so fish will defecate and vomit in response to stress to conserve energy2,3. Fishes should therefore be fasted prior to any procedure, including handling or transport. For small fish (<400 g), 24 h of fasting is sufficient, with 48 h for larger fish2. Fasting also reduces ammonia output and water contamination. High-quality water for procedures and recovery should be provided so that any gut emptying that occurs does not cause welfare problems.
The skin and mucous membranes of fish act as barriers to osmotic stress and infectious agents, and damage can lead to significant morbidity. The epithelium is thin, with a covering of mucus. In some species the skin is delicate and easily damaged by handling, especially prolonged restraint. Handling can also cause myopathy. Anaesthetics and sedatives are frequently used to prevent external damage during procedures.
Procedures should be conducted quickly and competently to minimise stress. Longer procedures may be best done under sedation unless the fish have been conditioned to handling. Recovery from the stress of procedures may take from a few hours to several days. Where fish are to be handled repeatedly, a suitable period of recovery is required between procedures. Some water additives are available to help maintain the integrity of the skin and aid recovery after procedures.
Many fishes are sensitive to visual stimuli, especially light, so handling in a dimly lit area may help lessen handling stress.
In general, fishes should not be kept in air continuously for more than 30 s. The length of time fishes are held out of water should be minimized, and should not exceed 30 s for most species. Even brief periods out of water can cause damage to gills, so these need to be kept moist3.
If fish are to be transported, small groups of fish can be placed in sealed plastic bags or boxes containing one-third tank water and filled with oxygen. During transport temperature should be maintained as far as possible, and on arrival fish should be acclimatised to the new environment: the water conditions should be as similar as possible, and the temperature in the transport water and receiving tank allowed to equilibrate before the fish are released into the new container. Fish should be closely monitored after transport for signs of ill health.
Identification
For some fish it may be possible to identify them from markings, avoiding the need for invasive marking techniques. Anaesthesia or sedation may be required for tagging or marking. No single tag or marking method is suitable for all situations.
Tagging involves stress and injury, from both handling and the wound caused by application of the tag3. Tags should be chosen which allow normal behaviour and minimise risk of entanglement. The weight, shape and size of the device must be considered. Brightly coloured tags may compromise an animal’s camouflage. Various types of internal and external tag, including small tags and passive integrated transponder (PIT) tags for subcutaneous and abdominal implantation, are available. Alternatively, small quantities of coloured compounds can be injected subcutaneously to mark the fish.
Marking techniques that cause significant tissue injury, such as freeze branding, tattooing or clipping important fins, should only be used if alternative methods are not suitable. Removal of even a small part of a fin can be detrimental to the ability of the fish to swim2.
Anaesthesia
Fish should be starved for 12–24 h before anaesthesia to prevent regurgitation. Anaesthesia can be achieved by immersion in a solution of appropriate anaesthetic. Tricaine methane sulphonate or MS222 (Pharmaq MS222, Pharmaq) is licensed in the UK for this purpose. MS222 produces dose-dependent sedation and anaesthesia, depending on the species and with individual variation. The level of anaesthesia depends on water temperature, water hardness, salinity, oxygen concentration and duration of immersion. Smaller fish tend to be more sensitive. Water for anaesthesia should be taken from the holding tank. The required amount of MS222 should be weighed and dissolved in a small quantity of water, then this added to the anaesthetic bath, in to which the fish are then placed. The water should be aerated to maintain oxygen levels and remove carbon dioxide. MS222 can be acidic, and water pH should be measured before and after adding the agent. If there is a reduction in pH of more than 1 pH point buffering may be necessary, using sodium hydrogen carbonate, to raise the pH to 7–7.5. This is less likely to be necessary with hard water at concentrations used to induce anaesthesia7.
Since fish vary in their sensitivity, a low initial anaesthetic concentration should be chosen and tested on a small number of fish, and more anaesthetic added if required. Initially there will be increased opercular movement, followed by a reduction in opercular movement, increasing ataxia and loss of balance before immobility. It may take 10 min for anaesthesia to be induced. Depth of anaesthesia can be judged by respiratory rate and (lack of) response to pinching the tail base. If anaesthesia is to be maintained a lower concentration of anaesthetic can be trickled over the gills (50–100 mg/l).
Following the procedure the fish should be placed in clean water and will return to consciousness within 1–30 min.
Table 16.2 gives suggested induction doses for MS222 in different species. Anaesthesia can also be induced and maintained with isoflurane, or with a mixture of MS222 and isoflurane. Isoflurane can be added to the water at 0.4–0.75 ml/l for induction, reducing to 0.25–0.4 ml/l for maintenance. Other anaesthetics used in fish include benzocaine and clove oil8.
Table 16.2 Induction of anaesthesia with MS222
Administration of compounds
Immersion
The most common route for administration is via the gills. Fish gills have a large surface area and a thin epithelium for gas transfer, which also allows for absorption of other molecules. Compounds for administration by this route are dissolved in a quantity of tank water, usually in a separate smaller vessel. Fish must be observed for signs of ill health during the period of exposure and the water aerated or changed as appropriate to maintain water quality.
Oral
Fishes may be force-fed liquids and semi-solid solutions using flexible rubber tubing and a syringe. Anaesthesia may be necessary to prevent struggling and vomiting. In some species a rigid speculum may be used to aid passage of the tube. Regurgitation may occur, so fish should be carefully observed to ensure the administered agent is retained. In general, no more than 1% body weight should be administered orally in a single dose3.
Injection
Common routes for injection in fish are intravascular, intraperitoneal and intramuscular. Care should be taken during injection to introduce the needle in spaces between the scales. Intramuscular injections may be made into the large dorsal epaxial and abdominal muscles, taking care to avoid the lateral line and ventral blood vessels. Intraperitoneal injections should avoid penetrating abdominal viscera.
Implants and slow release devices can be surgically implanted in the peritoneal cavity or implanted with a trocar into muscle.
Collection of samples
Samples of blood or tissues may be required for study. Some samples may be taken from anaesthetised fish which are then allowed to recover, but often with small fish samples are taken post mortem. Fish deteriorate very rapidly after death, and samples must be taken often within a few minutes to be of value2.
Blood
In general, blood sample volume up to 1 ml/kg of body weight is recommended. The fish must be permitted to recover their haematocrit prior to subsequent blood collection. Haematocrit recovery times are temperature-dependent and highly variable between species.
Methods for blood collection are listed below2,9.
- Tail ablation after euthanasia: fish that are too small to bleed with needle and syringe may need to be sampled after euthanasia. The caudal peduncle is severed with a scalpel and blood collected with a capillary tube.
- Caudal venous puncture: the caudal vessels lie under the spine. The fish is placed on its side and the needle is inserted in the ventral midline just behind the anal fin angled slightly forwards. Alternatively, a lateral approach can be used, inserting the needle between the scales just below the lateral line in the middle portion of the tail base. The needle is inserted slowly upwards towards the vertebral column until the bone is reached, then the needle withdrawn slightly and the sample taken.
- Cardiac puncture: this is a tricky technique and should only be carried out after extensive training and practise on cadavers. The needle is placed perpendicular to the skin and inserted slightly below the gill cover.
Surgery
The mucus and skin of fish contain antibacterial agents, and removal of mucus and disruption of scales during surgical preparation may render the area susceptible to infection, so the surgical scrub procedures used in mammalian surgery are not recommended. Gentle removal of grossly visible dirt and debris may be sufficient, followed by cleaning with dilute aqueous povidone/iodine solutions. Quaternary ammonium compounds may be irritant and should be avoided. If it is necessary to remove scales they should be removed individually by pulling in a posterior direction to minimize damage. Scale removal results in an injury which extends into the vascular tissue. The use of sterile occlusive drapes can help maintain a sterile field. Sterilised cling film can be used for this purpose: this can be wrapped round the fish and the incision can be made through it. Sterile instruments should be used.
The incision should avoid the lateral line and should follow the longitudinal axis of the fish. The epidermis and peritoneal layers are easily torn, but overall fish skin is tough. Abdominal incisions may be made on the ventral midline or lateral to this region. Ventral midline incisions avoid muscle tissue, but the incision may come in contact with the substrate in the tank, causing damage to the incision site. Lateral approaches avoid this problem, but invade muscle and may result in accidental puncture of underlying organs.
When closing incisions, since the skin layers are all closely adherent to each other, a single layer of sutures through all layers is sufficient in most cases. Suture material should be monofilament, inert and placed with an atraumatic needle. Internal layers and organs can be closed using synthetic absorbable materials. Tissue adhesives may not be suitable, as they only bond dry material so may not hold in aquatic environments.
In general, fish skin heals faster than mammalian skin; however, fibrous proliferation can be slow and varies with temperature. Factors that affect wound healing include water quality, presence of tank mates, nutritional plane before surgery and speed of return to normal feeding, hormonal status, integrity of mucus layers and skin, and the presence or absence of opportunistic pathogens.
Post-operative care and analgesia
Little is known about the effect of analgesic drugs on fishes. However, post-operative analgesia should be given where appropriate as suitable analgesic agents become available. Fishes do appear to produce opioid substances in response to pain and fear, similar to higher animals10,11,12,13, and respond to analgesics similarly to mammals.
There may be prolonged metabolic effects following anaesthesia and surgery, therefore careful monitoring and care for several hours in the post-anaesthetic period are needed. A quiet, well-aerated, possibly darkened tank with clean water where the fish can be easily observed is recommended for recovery, where there is minimal environmental stimulation and a consistent temperature. Post-anaesthetic complications may include wound dehiscence and infection, osmotic imbalances related to surgical incisions, and anorexia.
Care is needed when returning the fish to the pre-surgery tank. The benefits of companion fish (social interaction, schooling fish and feeding activity stimulation) have to be weighed against the disadvantages (predation, competition for feed and biting of sutures). Shelters for recovering fish and water-conditioning agents may help speed recovery3.
Health and disease
Fish used for research should be free of diseases, since these can affect results. There should be procedures in place to limit the introduction of disease into the facility, and a programme for the detection and management of disease conditions and water-quality problems. Disease-control measures, such as quarantine, immunisation and prophylactic treatments, and a system of regular health monitoring should be in place3. Handling should be minimal to minimise stress. Many diseases in fish can be prevented through good husbandry and biosecurity measures. New fish are one of the largest risks for introduction of disease, so new stock should be sourced from colonies free from infectious diseases, and quarantined for a suitable period (3 weeks or more2) on arrival. Sick fish should be removed from the colony immediately. Mixing different groups of fish should be avoided. Water is another potential source of infection, so incoming water should be treated if possible to minimise the risk of introducing disease. Ideally separate tanks should receive separate supplies of water: systems which circulate water between different tanks facilitate the spread of disease. An ‘all-in all-out’ policy with disinfection of the system between batches of fish is recommended2. Shared equipment such as nets should be properly disinfected between tanks.
Recognition of pain and distress
It is only recently that studies have been conducted into the cognitive capacity of fish. Recent studies have identified nociceptors in fish, and fishes respond to noxious stimuli with altered behavioural, physiological and hormonal parameters. The responses seen are similar to those seen in more highly evolved vertebrates14. There has been debate whether these responses demonstrate that fish have a capacity for fear and suffering14, or are simply responding passively to stimuli15,16. However, there is increasing evidence that fish have the capacity for long-term suffering17. Either way, there is conclusive evidence of a stress response in fish that can be detrimental to physiological processes, irrespective of the cognitive abilities of the animal. Therefore it is essential to minimise stress when managing fish in the laboratory.
The recognition and evaluation of pain and distress in fishes is not easy. Many fish species are prey animals and do not exhibit overt signs of injury or pain. Time should be taken to observe and record the normal behaviour and appearance of the fish in the particular setting, to facilitate identification of any abnormalities2. Reluctance to eat, unusual behaviour, discoloration of the integument and lesions are signs of possible developing health problems3. Signs of ill health also include reddening around the mouth and vent, swimming close to the surface or erratically, inability to maintain posture, swollen abdomen, lethargy, darkening, blue-grey slime or white patches on body, loss of condition, or a cloudy lens in the eye. One approach is to draw up a checklist of anticipated and potential clinical signs for each study, including ‘population’ clinical signs (such as feed intake) and ‘individual’ clinical signs (such as skin lesions). End points can then be defined for each study (see Chapter 4 for more information on end points).
In the long term, the effects of stress can include loss of appetite, inhibition of growth, impaired reproductive success and impaired immune response.
Euthanasia
EU Directive 2010/63 permits euthanasia of fish by overdose of anaesthetic, electrical stunning or concussion, although electrical stunning is not permitted under Schedule 1 to the Animals (Scientific Procedures) Act 1986. Overdose of anaesthetic is often achieved by use of a buffered solution of MS222 (tricaine methane sulphonate) at a concentration of at least 250 mg/l. Death usually occurs within a minute, but animals should be kept in the solution until death is certain, at least 10 min after opercular movements cease. Fish may also be given an overdose of pentobarbitone (60–100 mg/kg) by intravascular or intraperitoneal injection.
Where feasible, the euthanasia of fishes using physical methods should consist of a two-step process, with initial anaesthesia followed by a physical method to cause brain death, except when animals are in distress and immediate euthanasia is required. A stunning blow is administered to the head, followed by pithing or cervical dislocation. Many species of fish continue to have brain activity when there is cerebral and systemic hypoxia; therefore physical euthanasia techniques such as decapitation alone should be avoided. It is recommended that the brain is destroyed in fishes that have been euthanised using a physical technique.
Various forms of electrocution have been used commercially; however, these can cause spinal fractures and muscle damage.
Zebrafish
Zebrafish, Danio rerio, are teleost fish of the Cyprinid family. They are widely used in studies of developmental genetics, neurophysiology, biomedicine and toxicology. Their genome consists of 1.7 gigabases, which is just over half of that of mammals, divided into 25 chromosomes5. Many wild-type and genetically modified lines are available (see http://zfin.org for details)18.
Zebrafish are found naturally in slow-flowing shallow turbid pools in south and south-east Asia, often in unshaded locations2,19. They appear to inhabit floodplains rather than rivers. They inhabit the whole water column, including open water and among aquatic vegetation.
Zebrafish are small (up to 40 mm long) and robust, and large numbers can be kept in the laboratory. Females tend to be larger than males.
The natural history of the zebrafish is not well understood, thus husbandry protocols are not well developed and will vary between laboratories. Zebrafish tolerate a range of conditions, but production will be optimized within a set of parameters19. The optimum ranges have not been established and the recommend parameters are based on practices that have been found to be successful2.
Sources of fish
Eggs or larvae should be acquired from clean sources, but it is wise to keep new stock in quarantine for 2–3 weeks, checking daily for signs of disease, before moving into the main facility5. Adult fish should not be allowed to leave the quarantine facility: eggs obtained in the quarantine room should be bleached4 before leaving the quarantine room to be reared in main facility.
Behaviour
Zebrafish are a shoaling species, and establish dominance hierarchies that do not seem to be related to sex or size. Zebrafish can be aggressive at low densities, and may chase or bite subordinate fish19. As density increases aggression may reduce, but stress occurs in crowded conditions so they are best kept at intermediate densities. Aggression can be reduced by adding complexity to the environment – for example, plastic grass – so the fish can hide from each other, and fish are less aggressive in a covered habitat. Once dominance is established, aggression reduces. When mating male zebrafish may show territorial defence and active pursuit of females. Zebrafish release a pheromone when there is damage to the epidermis, which causes alarm in other fish. When alarmed there is an increase in shoaling behaviour, and either agitated swimming or freezing on the substratum, reduced appetite and increased aggression. There are differences in behaviour between strains of zebrafish.
Husbandry
Zebrafish are commonly kept in commercially available recirculating systems where several tanks are held on a rack and supplied by a single filtration column. The stocking density depends on the efficacy of filters, the age of the fish and the amount of feeding. In recirculating systems families of adult fish can be kept in serial tanks at five adult fish per litre.
In non-filtering systems fish must be held at a low density. Adult zebrafish can be maintained for up to 10 days without feeding or water exchange at a low density (two fish per litre). For longer periods regular water exchange and feeding is needed. Juvenile fish can be held in static tanks at at 60 per 2.5 litres until they are large enough not to pass through filters (2–3 months).
Zebrafish are surface-living, so tank height of 25 cm is sufficient provided there is adequate surface area. A well-fitting clear plastic lid is needed with a feeding hole smaller than 1 cm; otherwise fish can readily escape.
Zebrafish need temperatures between 25 and 28°C. The room temperature can be set higher to prevent condensation and growth of mould on tanks. Higher temperatures reduce oxygen levels in water, so if heaters fail and water drops to room temperature it is not dangerous. If temperature falls outside 25–31°C they may not breed or may show abnormal development4.
Zebrafish usually have 14 h light/10 h dark cycle. Fish do well in dim light, and bright light encourages algal growth. Red light is often used so researchers can see fish during the dark phase without encouraging algal growth.
Feeding
The exact feeding requirements of zebrafish are unknown19. Zebrafish appear to be omnivorous, their natural diet consisting mainly of zooplankton and insects. Adult fish not being bred require little feed and can be fed twice weekly. For breeding, fish require rich feeding. Typically these animals are fed dry food twice daily, with live food such as Artemia or bloodworms being added twice weekly or more, as dry food may not be sufficient5. Care must be taken not to overfeed the fish as degenerating surplus food impacts on water quality, and tanks need cleaning daily to remove excess food and faeces.
Breeding
Although in the wild zebrafish appear to breed annually, in the laboratory they can breed all year round. Zebrafish mate and spawn at dawn, and females spawn in response to male pheromones. To breed, pairs of fish can be set up in mating tanks in the afternoon or evening, with plastic grass to reduce aggression. Lids are essential as zebrafish can jump. Most fish will spawn the next morning, although some will only lay the second day. The male will chase and nudge the female, and may lead her to a spawning site. He will remain close to her and release sperm as she lays her eggs. Females can spawn every 2–3 days, and a single clutch typically contains 100–200 eggs2, but can contain as many as 700 eggs19. In the laboratory they spawn when the light is turned on in the morning, and the light cycle can be manipulated to produce eggs when required for study5. There is no parental protection2, and indeed eggs may be eaten by their parents. Eggs can be protected by using glass marbles at the bottom of the tank, or by using a mating container, which has a removable inner container with a perforated base.
Eggs should be collected before noon the day they are laid. Unfertilised eggs promote growth of bacteria and mould, so eggs need to be removed rapidly into petri dishes containing suitable medium, and dead eggs removed regularly. Eggs may be bleached prior to raising to reduce the risk of disease4.
In vitro fertilisation can be used to obtain large numbers of synchronously developing embryos. Gametes are expressed from breeding adults by squeezing under anaesthesia4.
Hatching occurs between 48 and 72 h post-fertilization at 28.5°C2,19. Development is very rapid, with organs beginning to be identifiable from 36 h. After hatching, larvae attach to hard surfaces, attaching to progressively higher levels. Once they reach the surface they can inflate their swim bladders, which occurs within 5 days of fertilization (2–3 days after hatching), at which point they begin food seeking19. Larvae are kept in petri dishes up to day 5, when most have inflated swim bladders. They should then be transferred to a tank, and fed within 48 h. From days 6–15 they can be kept in aerated water with no water exchange (note: reverse-osmosed water must be aerated first), and fed twice daily with a pinch of appropriate food. Overfeeding must be avoided: uneaten food falls to bottom and rots. After 2 weeks the fish can be kept in continuous-flow water systems at a low flow rate so they are not flushed out and fed three times daily with live food. At about 5 weeks dry food is given as well. Once the fish reach 2–3 months they are transferred into larger tanks. Fish grow faster at lower densities. They grow very rapidly in the first 3 months then the growth rate reduces. They reach sexual maturity at about 4 months and will then start breeding. Fish continue to grow until about 18 months.
Initially all gonads develop as ovaries, then in males these begin to develop into testes after 5–7 weeks, maturing by about 3 months. Sexual maturity appears to be related to size, rather than age, when approximately 23 mm long. This typically takes around 75 days but is related to temperature and food availability. The mean life span for zebrafish is 42 months19.
Health and disease
Zebrafish are robust fish but can be affected by a number of diseases that can affect the entire colony, for example velvet disease, fish tuberculosis and nematodes. Pseudoloma neurophilia is a common fungal infection in laboratory zebrafish colonies, and the incidence will probably increase as the use of zebrafish in research increases. Appropriate biosecurity measures, husbandry and breeding strategies, and health-screening protocols should be implemented to minimise the effects of these diseases20.
Rainbow Trout
Rainbow trout are a teleost fish of the salmonid family. They are native to the Pacific drainages of North America, but have been introduced widely around the world for recreation and aquaculture. They are commonly used in ecotoxicology studies. Rainbow trout are very hardy, tolerating a wide range of environments. Some strains are anadromous (live in the ocean returning to spawn in fresh water), whereas others live permanently in freshwater lakes. They grow rapidly, with freshwater strains reaching 4.5 kg and anadromous strains reaching 7.5–10 kg within 3 years6. Rainbow trout live for 5–6 years and can reach 10 kg in weight2.
In the laboratory trout may be held in glass or plastic tanks. Circular tanks help maintain a regular current and uniform distribution of the fish, but square tanks are also acceptable. Tanks are usually 50–60 cm deep. Circulation systems are best. Water can be delivered in circular tanks to the side of the tank so as to create a current, with a drain in the centre of the floor: water then forms a vortex towards the centre that accumulates wastes for easy removal. The drain should be protected by a mesh screen.
Feeding
In the wild, adult trout feed on freshwater shrimp, aquatic and terrestrial insects, molluscs, crustaceans, fish eggs and small fishes. Fry can be weaned onto an artificial diet.
Fry are fed specially prepared starter feeds, starting from when approximately 50% have reached the ‘swim-up’ stage when they begin seeking food. When most fish are actively feeding they should be fed 10% of their weight daily for 2–3 weeks. When the fry are 15–25 mm long feeding is based on published charts, related to temperature and fish size. In the early stages overfeeding must be avoided. As growth continues fish are moved to larger tanks to reduce density6.
Breeding
Maturation in salmonids is triggered by decreasing photoperiod2, but spawning times vary between strains. Wild rainbow trout usually spawn once yearly, in spring6, but some cultured strains can spawn all year round. Eggs are laid on gravel nests, covered with gravel and left to mature2. Trout rarely spawn naturally outside their native range, and juveniles may need to be obtained from hatcheries6. Photoperiod can be manipulated to produce mature males and females, and embryos produced by artificial fertilization using eggs and milt removed manually from mature fish (males 2–3 years old, females 3–4 years old) under anaesthesia. Hatching depends on temperature, taking approximately 300 degree days2, for example approximately 100 days at 3°C and 21 days at 14.4°C. Trout hatch with a supply of food in the yolk sac, which lasts 2–4 weeks. Once the yolk is absorbed the fish begin actively seeking food. This is known as the swim-up stage.
After hatching, growth is rapid, with males reaching maturity at approximately 2 years and females 3 years.
Health and disease
Rainbow trout may develop a number of infections in the laboratory, including sleeping disease, furunculosis or rainbow trout fry syndrome. The presence of disease may only be identified through an increase in mortality. Fry often carry these diseases subclinically, and outbreaks of disease may not be seen until the animals are stressed by procedures.
Amphibians
The class Amphibia includes a variety of aquatic, semi-terrestrial and terrestrial tetrapods, which have colonized a number of particular habitats successfully. There are about as many amphibian species as mammals21. Amphibia are ectothermic, their body temperature being determined by the environment, although they can use behavioural methods and evaporation to control temperature to some extent. This means their metabolic rate falls at low temperatures, allowing them to survive on very little food. There are three orders of amphibian: Apoda, which have no legs and resemble worms or snakes; Urodeles, which includes newts and salamanders, and Anurans, which includes frogs and toads. The Anurans are tail-less apart from larval stages, with well-developed hind limbs adapted for jumping. The head and trunk are fused and the toes are webbed.
Amphibian skin is moist and delicate, and covered in mucus. The skin acts as a barrier to infection and secretes numerous chemicals with protective functions. Their skin secretions are toxic to deter predators, and stressed animals will produce an excess white secretion. This can be irritant to the mucous membranes of the handler, and can also adversely affect the frogs themselves, leading to morbidity. Affected animals should be removed to clean water and the excess secretion removed. Amphibians do not drink, absorbing moisture through their skin, although they also lose water by this route. They cannot concentrate their urine, and are susceptible to dehydration if placed in dry environments.
Amphibians are unusual in that they respire through the skin, as well as through their lungs and mouth. Frogs don’t have ribs, so air is pumped into the lungs by the muscles in the mouth. Larval stages respire via gills which degenerate after metamorphosis.
The most common amphibians used in the laboratory are the anurans Xenopus laevis (the African clawed frog) and the smaller Xenopus tropicalis (the western clawed frog). Xenopus are used particularly in studies of developmental biology because they have a large and easily manipulated embryo, with a single pair of adults producing many thousands of offspring with limited genetic variability. Xenopus oocytes are used in molecular biology and studies of DNA replication and repair. The first vertebrate to be cloned was an African clawed frog22, and genetic modification of Xenopus embryos is now relatively commonplace. Xenopus, like other amphibians, can regenerate lost limbs.
Biology and behaviour
For detailed information on the biology of Xenopus species see Tinsley and Kobel23.
Xenopus originate from sub-Saharan Africa. They have three claws on each hind foot, from which their name is derived: Xenopus means ‘strange foot’. They use the claws to tear up large food pieces, then feed by using their hands to push the food into their mouths, where they use a pump mechanism to suck the food in. They are tongueless, toothless and completely aquatic. They have powerful legs and are strong swimmers. They have no ears, but sense movement and vibration via lateral lines running down the length of their body. This skin is smooth, and greenish-grey on their backs with a creamy white underside. Albino varieties also occur. They shed their skin every season, and may eat their own shedded skin.
Wild Xenopus are found in swamps and ditches, where the water is cloudy and affords some protection from predators. X. laevis occurs in the cooler parts of a wide range of habitats from South Africa to Sudan. A number of subspecies are recognized, and most laboratory research has been done on X. laevis laevis from South Africa21.
X. laevis are nocturnal and secretive creatures, preferring dark and cloudy water. They communicate by vocalization and maintain dominance hierarchies. Males call to attract females, and females call back in reply. In the laboratory they are often seen hanging seemingly immobile at the water surface with nostrils protruding. They may even call, particularly in the evenings. They are able to adjust their buoyancy so they can hang like this without effort. Captive X. laevis can become tame and may take food from a handler’s fingers.
X. laevis are robust, and animals can survive and continue to spawn for 15–20 years or more, reaching a length of 12 cm. X. laevis are tetraploid, so there are extra copies of many genes that may or may not be functional.
X. tropicalis is found in the tropical forests of West Africa, suggesting they prefer shade and a relatively constant temperature. It is the only species in the Xenopus genus to have a diploid genome. Its genome has been sequenced24, and it is used in studies of genetics. It has a shorter generation time (<5 months), smaller size (4–6 cm body length) and a larger number of eggs per spawn compared with X. laevis. See Table 16.321,25 for biological data on X. laevis and X. tropicalis.
Table 16.3 Xenopus biological data21,25.
X. laevis | X. tropicalis | |
Average size (cm) | ||
Male | 5–10 | 3.9–4.5 |
Female | 10–15 | 4.8–5-5 |
Clutch size | 300–1000 | 1000–3000 |
Length of metamorphosis | 2–3 months | 1 month |
Age tadpoles begin to feed | 5–10 days | 3 days |
Lifespan (years) | 15–20 | 25–30 |
Temperature range (°C) | 18–20 | 24–26 |
Feeding frequency (per week) | 1–3 | 2–3 |
Heart rate/min | ||
At 2°C | 8 | No data available |
At 25°C | 40–60 | |
Generation time | 1–2 years | 4–6 months |
Chromosome number | 18 | 10 |
Male Xenopus are usually smaller than females, with slimmer bodies and legs. Females are larger, with hip-like bulges above their rear legs. Both males and females have a cloaca, through which the digestive, urinary tract and reproductive systems pass. The cloaca empties through a single opening called the vent. Females have larger ventral flaps, called anal papillae, located immediately above their cloacas. Males have black, spiny nuptial pads on the inner arms and enlarged thumbs to help grip the females during breeding.
Adult Xenopus have traditionally been sourced from the wild. As such they are used to natural conditions and transfer to the laboratory may cause significant stress, and they may adapt poorly. In addition they may be carrying parasites or other disease organisms. It is recommended that amphibians for use in the laboratory be purpose-bred.
In the wild, like most amphibians, social behaviour is mainly restricted to the mating season. However, group housing is advisable, as it improves feeding and reduces fear responses. Group feeding promotes feeding, inducing all animals to feed. At very low stocking densities food is frequently not eaten.
Identification
To identify individual animals there are several non-invasive methods. X. laevis have unique patterns on their backs, and photographs can be used to differentiate between them. This is more difficult in X. tropicalis, for which the markings are less distinct. However, in both species the colour or pattern of the skin, and the pattern of the blood vessels in the toe webs, can be used for identification, either using visual assessment or via electronic scanning devices. Microchip transponders can also be implanted subcutaneously or into the dorsal lymph sac. Ink spots tattooed onto the belly can persist for several years. More invasive methods such as freeze branding or toe clipping are not recommended.
Husbandry
Laboratory housing for Xenopus has to balance the conflict between their natural environment (deep, murky, still water with vegetation or shade to provide cover and protection from predators) and the experimental requirement for easy maintenance and observation, and access to the animals. In addition, there is often no agreed optimum husbandry method. Minimum requirements can be found in reference 26.
The environment in the laboratory should be designed to match their natural habitat as closely as possible. Both species can be kept in similar conditions, with slightly higher temperatures for X. tropicalis, but they should not be kept in the same system. Tanks for Xenopus should ideally have dark sides, being lit from above, with refuges and some cover. Xenopus are found in the wild in deep water, and water that is too shallow may lead to stress. The minimum required depth is between 6 and 12.5 cm depending on the size of the animal26, but for optimum welfare a depth of 30 or even 50 cm may be preferable21. Animals are commonly kept in a water depth of 15 cm with a lid 5 cm above the water surface. Xenopus can jump, so tanks should have well-fitting lids, up to 20 cm above water surface to prevent trauma if the animals do jump. Refuges and cover should be provided, using plastic pipes or vegetation. Recommendations for stocking density for X. laevis vary from 2 litres per animal to 12 litres per animal, and 0.6–0.8 litres per animal for X. tropicalis. Adult animals sourced from the wild may find it difficult to adapt to such intensive systems21.
Xenopus can be kept in static water systems, where water is changed periodically, or recirculating systems, which provide a constant flow of clean water with controlled temperature and composition.
In static systems water should be changed at least weekly. Since regurgitation of food is common if Xenopus are disturbed too soon after eating, this should be done at least 3–5 h after feeding. This facilitates removal of food debris. To avoid the risk of regurgitation water can be changed before feeding, which allows feeding in clean water and reduces stress in the post-feeding period, but food debris then remains in the tank for a longer period. In static systems a substrate of gravel is provided to support a culture of bacteria to denitrify the water.
In recirculating systems the water is filtered and aerated, but the flow rate must be low, so as to produce minimal disturbance to the water, as Xenopus rarely inhabit flowing water in the wild. Manual removal of debris may still be required.
Changes in temperature need to be gradual to avoid stress, so when changing tanks, frogs must go into clean water of a similar temperature, and handling should be minimal.
Water should be dechlorinated (tap water can be left to stand for 24 h), or treatments added to remove chlorine. In self-contained water treatment systems, salts may be added to maintain conductivity. Water quality, particularly ammonia and the pH level, should be regularly monitored. Ammonia is excreted by amphibians and its concentration will depend on the water handling regime. The level should be kept below 5 mg/l, depending on the pH (potential harm increases as the pH rises)26.
Frogs are very sensitive to toxic substances and traces of hand lotions or other chemicals on the hands of handlers may kill the animals. Personnel should always wear impervious gloves to handle frogs or change their water. Many factors affect water quality and water-quality parameters should be checked frequently. Table 16.1 lists appropriate water-quality standards for aquatic species.
Xenopus can adapt to a wide range of temperatures, but spawning seems to be optimal at 20°C for X. laevis and 24°C for X. tropicalis. All aspects of the physiology of Xenopus are affected by temperature, for example immunosuppression occurs below 18°C in X. laevis27 and they become less able to withstand stress as temperature falls. At higher temperatures more food is needed as the metabolic rate rises. The optimum is approximately 18–20°C for X. laevis, and is higher for X. tropicalis (25–30°C)21,26,28. Tadpoles prefer slightly warmer temperatures.
For breeding, ‘daylight’ fluorescent tubes should be used to provide sufficient ultraviolet light to maintain vitamin D levels. A photoperiod of 12 h/12 h or 13 h/11 h light/dark is appropriate26.
Feeding
As juveniles and adults, Xenopus are carnivorous scavengers and will eat both dead and live prey and may cannibalise their own tadpoles. They feed under water, detecting prey by vibrations, odour and sight. Xenopus are unusual in that they will feed communally on large prey. In nature, group feeding on large items can trigger a ‘frenzy’, which encourages all individuals to eat. This may not be advantageous in the laboratory, and offering smaller pieces of food may avoid competition and fighting.
Adult Xenopus can be maintained on one to three high-protein feeds each week depending on temperature and light level, being given just sufficient food to be consumed within 30–60 min, but so all animals are fed to satiation to avoid competition21. They can be fed pieces of beef heart or fish fillet. Commercial diets are also available. Any remaining food should be removed to prevent contamination of water: this is harder with pelleted diets as they disintegrate. Young animals require more frequent feeding: three to four times weekly or even daily.
Tadpoles are filter feeders, eating phytoplankton. They may be fed algae, drops of strained vegetable baby food (green beans or peas), liquified fish food or commercial powdered tadpole food. More food is added only once the previous food has been consumed.
Breeding
Amphibians are unique among vertebrates in that the juvenile and adult forms are different: fish-like tadpoles undergo metamorphosis into the tetrapod juvenile form. Wild X. laevis show seasonal cycles in breeding, spawning from early spring to late summer. Females may spawn up to three times in this period.
X. laevis usually takes 1–2 years to reach sexual maturity, but it can take only 6 months post-metamorphosis in the laboratory21. Spawning can be spontaneous but is usually induced in the laboratory by administering one or two injections of human chorionic gonadotropin (HCG), 48 h apart. This allows for year-round breeding. Adults should be in breeding condition (females well-fed and plump, and males with black nuptial pads on the insides of the arms). Male and female can be put together after the second injection into a spawning tank with a perforated plastic base to protect the eggs. The male grips the female during a mating embrace, known as amplexus, then releases sperm over freshly laid eggs. The pair should be left undisturbed in a darkened tank until mating is over. Fertilisation is external. Alternatively, gravid females can be squeezed after injection to extrude mature oocytes. Fertilisation can be done if required by mixing with testicular extract obtained post mortem from hormone-treated males.
Females in good condition can be bred once per month, although an interval of 2–3 months is typical. Females that have not been bred for a period of 4–6 months may produce poor-quality eggs. Males can be bred more frequently.
Eggs hatch after 48 h, then larvae attach to a vertical surface until the yolk sac is absorbed. They are filter feeders and will begin to feed on suspended food particles, at 5–10 days in X. laevis and 3–4 days in X. tropicalis. They hang, head down, at a 45° angle in the water and use their tails to generate a current in the water to bring their food to them. Xenopus tadpoles are quite large compared to other species, and may need to be separated and re-accommodated several times before they metamorphose. Within limits, the speed of metamorphosis is related to the water temperature, but metamorphosis occurs at about 6–8 weeks. Tadpoles must be kept in clean, aerated water free from chemical contaminants, which is changed frequently. Dead or diseased larvae should be removed as soon as possible.
X. tropicalis have a much shorter generation time than X. laevis, of only 4 months. Breeding can be induced by hormone injection as for X. laevis. Larvae begin feeding from 3 days post-hatching.
Handling
Slippery skin makes handling amphibians difficult. Their powerful hind legs and claws mean they can escape readily and damage the handler. Approach the animal gently. Place one hand over the back of the animal with fingers orientated towards the feet. Place the index finger between the frog’s hindlegs, and use the thumb and other fingers to grasp it around the flanks. Grip the animal firmly and remove from water (Figure 16.1). It may be necessary to improve grip in some cases by holding animals with soft moist paper towel or cloth, but the animal must be kept moist at all times.
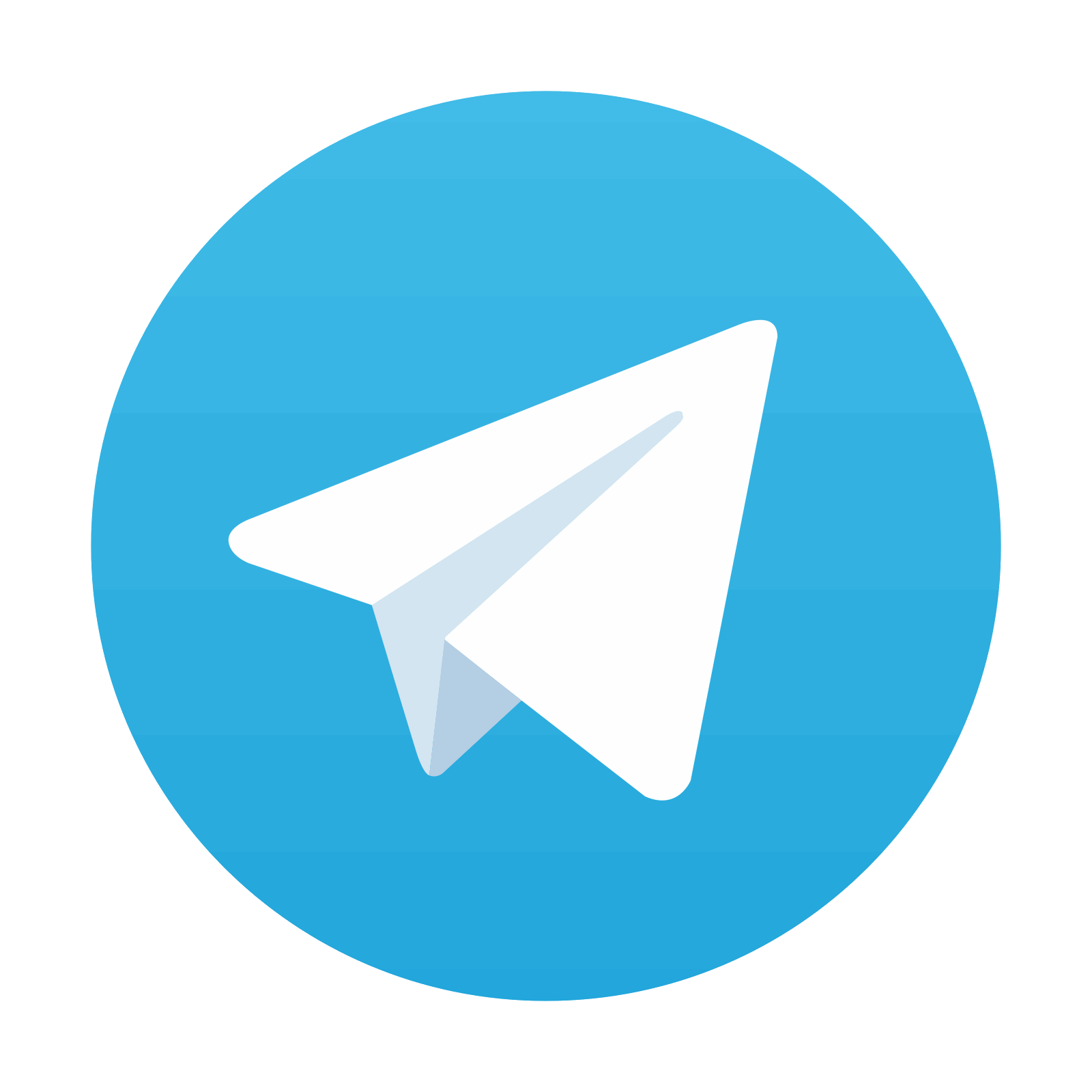
Stay updated, free articles. Join our Telegram channel

Full access? Get Clinical Tree
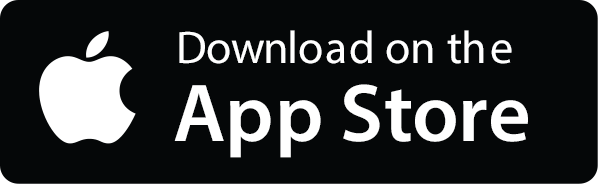
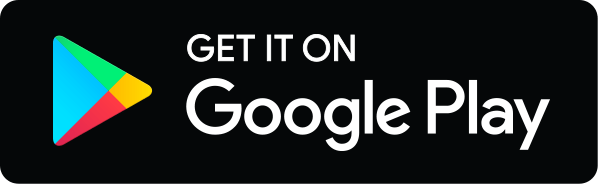