17 Applied Andrology in Endangered, Exotic and Wildlife Species Rebecca Spindler,1* Tamara Keeley2 and Nana Satake2 The long-term viability of any animal population relies on the reproductive success of the constituent animals. In turn, reproductive success is intrinsically linked to an individual’s health and well-being. As such, understanding reproductive physiology is essential to improving the genetic management, reproductive output and overall success of insurance populations of rare and important wildlife species. In addition, reproductive assessments should be incorporated into holistic programmes assessing the health and viability of wildlife populations. Assisted Reproductive Techniques (ARTs) can be extremely useful tools in the genetic management of these populations (Pukazhenthi et al., 2006a), but should be used in a manner that will support population sustainability in the long term. The relative ease of access to spermatozoa compared with oocytes results in greater opportunities to study andrology and, consequently, gain a better understanding of male gamete biology in a range of wildlife species. This has also led to the asymmetrical efforts and success in sperm banking compared with storing oocytes and embryos, in both domestic and wildlife species. While efforts are required to address this imbalance, the current capacity to collect, assess, cryopreserve and use spermatozoa to achieve targeted conception has great implications for the genetic management of species and conservation of biodiversity. Long-term cryopreservation of spermatozoa from wild animals, especially, can facilitate zoo-based breeding programmes by replenishing genetic material without the associated cost and anxiety of animal translocations (Wildt, 1997). Despite this potential application, detailed knowledge of species-specific methods for studying and manipulating male reproductive activity in wildlife species is limited. There is a wealth of andrological information available on domestic species (see other chapters in this volume, and Marshall’s Physiology of Reproduction, ed. Lamming, 1990, for summary) and humans (Campbell’s Urology, eds Walsh et al., 2002) that informs our study and control of male reproduction in wildlife species. Investigations of analogue domestic species have provided valuable launching points for investigations into wildlife reproduction and have led to the development of appropriate technology and equipment. However, it must be remembered that even closely related species often exhibit significant and biologically meaningful variations in reproductive and endocrine parameters. A successful approach to studying or manipulating male reproduction in one species will often not work in another species without significant modification. Thus, a significant amount of effort must be exerted to develop appropriate techniques for each wildlife species, regardless of the weight of research in taxonomically close species. Efforts to investigate wildlife andrology have focused on evaluating the semen of selected species and establishing baseline concentrations of circulating hormones (Spindler and Wildt, 2010). The numbers of animals typically included in such studies are extremely low in comparison with similar studies conducted on domestic species. This is largely due to the inability to collect longitudinal reproductive information from free-ranging animals that do not have a known life history. Investigations have relied on the availability of conspecific animals in zoos to provide baseline reproductive data, improve overall understanding of species biology and develop field techniques appropriate for the collection of samples and data from the field (Spindler and Wildt, 2010). Data collection from zoo-based animals is more laborious than it is for domestic species owing to the low numbers of animals held in each facility, the geographically dispersed locations of zoos and the diversity of environmental conditions experienced by each individual. Sourcing animals and collecting data in the field involves even greater expense and level of difficulty, and is often performed over many years, thereby introducing additional elements of variation. Understanding the andrology of wildlife species requires close observation, physical examination, sample collection and, possibly, ultrasound or radiography of males. In most cases, this will require anaesthesia in order to avoid stress to the animal and injury to the technicians. As a result, a great deal of research is invested in developing non-invasive measures of reproduction in wildlife species. Hormone monitoring of faecal samples has greatest application in the female to determine reproductive cycling, seasonality and maturity; it also has potential to assist the reproductive management of males (Morai et al., 2002; Pereira et al., 2005; Hesterman and Jones, 2009). These non-invasive techniques have the added benefit of reducing the number of anaesthetic events required to gain an understanding of male reproductive physiology, and so reducing the potential impact on animal well-being. However, most assessment of, and research into, male physiology requires the collection of semen samples and, in wildlife species, this necessitates anaesthesia. Modern anaesthetic regimes, developed to address species-specific physiology, have reduced the potential impacts for many species. The complete physiological impact of any anaesthetic regime should be considered fully before undertaking semen collection so as to ensure animal safety and well-being, as well as maximizing the chances of collecting a good-quality sample (Zambelli et al., 2007). Inbreeding depression most likely influences reproductive fitness in a variety of ways across a diverse range of species. For example, reduced genetic diversity is closely correlated with decreased testicular sperm concentrations in oldfield mice (Peromyscus polionotus) (Margulis and Walsh, 2002), decreased sperm quality in Cuvier’s gazelles (Gazella cuvieri) (Roldan et al., 1998), increased juvenile mortality in ungulates (Ralls et al., 1979) and small mammals (Ralls and Ballou, 1982), and reduced sperm quality in clouded leopards and lions (Wildt et al., 1986, 1987). Population management is essential to the reproductive health of populations and can be assisted by increased reproductive knowledge and the development of techniques to ensure gene capture and the maintenance of genetic diversity. It is well established that reproductive success is affected by environmental stressors (Ramaley, 1981; Moberg, 1985, 2000; Lasley and Kirkpatrick, 1991; Rivier and Rivest, 1991; Pottinger, 1999; Dobson et al., 2001, 2003). Wildlife species and, in particular, specialized carnivores, appear to be particularly susceptible to novel environments and stressors (Mellen, 1991; Carlstead et al., 1993a,b; Jurke et al., 1997; Carlstead, 2002; Moreira et al., 2002; Wielebnowski, 2002; Wielebnowski et al., 2002). These stressors result in the stimulation of the hypothalamic-pituitary-adrenocortical (HPA) axis. Both beneficial situations (e.g. courtship, copulation, obtaining prey, giving birth) and detrimental situations (e.g. fighting, capture, (some) transport) can elicit an adrenal response (Colborn et al., 1991; Moberg, 2000). Subsequent acute (short-term) elevations in glucocorticoids can help an animal to react appropriately to the challenge through rapid energy mobilization. In contrast, a chronic (long and sustained) glucocorticoid rise can reduce fitness through a variety of mechanisms, ranging from immunosuppression to poor reproduction and offspring survival (Moberg, 1985, 2000). These potential stressors must be managed or eliminated to ensure the optimal reproduction of wildlife species. Disease can be defined as any impairment that interferes with or modifies the performance of normal bodily functions; this includes non-infectious, infectious and parasitic diseases. Health and nutrition are intimately linked and many health issues are either directly related to poor nutrition, or are compounded by poor immune function due to poor nutrition. Owing to the tight regulations over the origin and quarantine of wildlife species, infectious diseases that directly affect reproduction are unlikely to be of concern in captivity. In free-ranging populations, diseases of concern that could be encountered include canine distemper virus, feline infectious peritonitis, genera-specific immunodeficiency viruses, rabies, herpesvirus, calicivirus, feline parvovirus (panleukopenia), feline leukaemia virus, Leptospira interrogans, Chlamydia spp., Salmonella spp., Toxoplasma gondii and Dirofilaria immitis (Spindler et al., 2007). In many such cases, the spermatozoa of valuable males may still be used after sperm washing and the verification of disease-free status before artificial insemination (AI) (Loskutoff et al., 2005). Nutrition plays a fundamental role in overall animal demeanour, hormone production, sperm production and fecundity. For example, malnutrition alters luteinizing hormone (LH) and testosterone secretion patterns in rhesus macaques (Macaca mulatta) (Lado-Abeal et al., 2002) and reduces overall fecundity in the marmoset (Tardif and Jaquish, 1994) and the water buffalo (Oswin-Perera, 1999). More specifically, diets lacking in specific micronutrients will have an impact on reproduction. For example, calcium influences erectile function (Mills et al., 2001), spermatogenesis (Andonov and Chaldakov, 1991), oocyte maturation (Machaca and Haun, 2002), fertilization (Stricker, 1999) and embryo development (Stricker, 1999). Further, deficiency in vitamin A causes male infertility, embryonic loss, decreased neonatal survival and fetal malformations (Clagett-Dame and DeLuca, 2002), while vitamin D deficiency reduces fertility by controlling the onset of puberty and fertility in both males and females (Halloran and DeLuca, 1979). Attention to the specific dietary requirements of wildlife species must be paid to avoid risks to health and reproduction. For example, felids have a unique and specific requirement for micronutrients such as arachidonic acid, which is essential for reproduction and sperm-atogenesis (MacDonald et al., 1984). Reproductive strategies employed by wildlife species range from asexual reproduction through promiscuity, polyandry and polygyny to monogamy. The mating strategy of the species in question will have a significant influence on male reproductive characteristics, including testis size, sperm characteristics, and even semen content. Knowledge of these strategies also allows some assumptions to be made when sampling from a species for the first time, and may also assist with developing sample collection and handling methods. For example, males in competitive breeding situations (polyandry) will tend to produce more semen, more spermatozoa and have larger testes than males with limited competition (Parker, 1998). Further, some primate, rodent, marsupial and reptile species ejaculate a coagulated ‘plug’ to prevent easy access to females by subsequent mates, which may cause damage to tissues if not completely expelled from the male reproductive tract during stimulated ejaculation. Wildlife species often employ mate selection strategies that avoid inbreeding and ensure the best possible mate to avoid neonate or infant mortality. Selection tools are used to select appropriate matings in globally managed zoo programmes, and these have been demonstrated to maintain excellent levels of genetic diversity in populations over many generations (Ballou and Foose, 1996). However, the loss of genetic diversity is almost inevitable over generations. Supplementation of an insurance population with genes from wild males (using cryopreserved spermatozoa) between three and five times a year is predicted to maintain the long-term viability of even small populations of Eld’s deer (Panolia eldii), Przewalski’s horse (Equus ferus przewalskii) and the Sumatran tiger (Panthera tigris sumatrae) (Harnal et al., 2002). Species biology will often dictate that offspring gender will be skewed under specific environmental conditions. While the specific conditions triggering this imbalance may be difficult to tease apart, the impact may be resolved through the application of advanced sperm gender-sorting technologies developed using domestic species (Johnston, 1992). These technologies are still in their infancy for wildlife species. Active gender selection of offspring would resolve many issues around the management of populations with a gender imbalance (usually towards the male) that is not representative of wild populations. The ability of human spermatozoa to withstand sub-zero temperatures was realised in the 19th century by the work of Mantegazza, although the potential applications of this technique were not recognized until the mid-20th century. The critical consideration of precise conditions and manipulations of the freezing and thawing processes to a vast number of species across a wide range of taxa has enabled long-term preservation of viable spermatozoa. Of primary concern in the development of cryopreservation methods is the avoidance of cryoinjury during the transition through solutions of altered tonicity and temperature (Mazur, 1963). These transient states include moving from isotonic solution to hypertonic solution to supercooled solution with the greatest concern for cell integrity between the temperatures of –30°C and –40°C, at which point intracellular freezing and ice crystal formation occurs. Vitrification, whereby freezing occurs at an ultra-rapid rate in order to avoid the formation of ice crystals, is a lesser studied avenue of cryopreservation for spermatozoa, but is the preferred method of preservation for organized groups of cells, such as testicular tissues and embryos; this turns specimens into a glass-like formation (Vajta and Nagy, 2006). Frog spermatozoa are able to survive exposure to liquid air at –192°C (Luyet and Hodapp, 1938). Fowl spermatozoa were revived after partial dehydration, freezing to –76°C and storage over several months (Shaffner, 1942). Human spermatozoa have been known to be particularly resistant to the vitrification process (Isachenko et al., 2003, 2012). Vitrification has been successful in recovering motile, viable spermatozoa after thawing in various species, including canids (Sanchez et al., 2011), rhesus macaques (Dong et al., 2009) and rainbow trout (Oncorhynchus mykiss) (Merino et al., 2011). The addition of glycerol was found to be beneficial to sperm cryopreservation in the mid-20th century, and since then the preferred cryopreservation methods have incorporated the use of cryoprotectant agents (CPA) to the present day. The main properties that determine an appropriate CPA include solubility, the ability to form hydrogen bonds with water and the impact on the melting point of ice with a view to reducing ice crystal formation and moderating cryoinjury. Two classes of CPA exist, penetrating and non-penetrating; penetrating substances have a molecular weight of less than 100 daltons and are able to penetrate cells. Examples of CPA routinely used in cryopreservation of spermatozoa include penetrating substances such as glycerol, dimethyl sulfoxide (DMSO) and sugars, as well as non-penetrating substances such as polyvinylpyrrolidone and polyethylene glycol. Sperm viability post-thaw may be considerably compromised owing to species-specific sensitivity to the CPA or concentration of the CPA used. Even within taxonomic families, species and individuals may differ markedly in their response to CPA. For example, in the domestic livestock industry, bull semen is routinely cryopreserved with commercially produced extenders and used for AI. However, using a basic semen extender developed for the domestic bull, such as a Tris-egg yolk-glycerol extender, can damage sperm DNA, plasma membranes and/or acrosomes from a related species, such as the European bison (Bison bonasus) (Pérez-Garnelo et al., 2006) and the buffalo (Bubalus bubalis) (Andrabi, 2009). Other examples of animals with varying and species-specific needs include ferrets (Mustela putorius furo) and black-footed ferrets (Mustela nigripes) (Howard et al., 1991), dogs and wild canids (Farstad, 1998), and cats and wild felids (Pukazhenthi et al., 2006b; Herrick et al., 2010). The ability to collect, cryopreserve and use spermatozoa from free-ranging males of any species can effectively increase the genetic diversity of a closed population, while avoiding the removal of animals from the wild, the potential stress of animal transport and the risk of disease introduction. The disadvantage of this approach is that field conditions often include an absence of electricity for cooling and centrifuging spermatozoa. Spermatozoa are clearly sensitive to high temperatures (England and Ponzio, 1996; Brinsko et al., 2000; Johnston et al., 2000a; Nair et al., 2006; Purdy, 2006), and long-term exposure to seminal plasma has been shown to compromise sperm motility, viability and even DNA integrity (Wildt et al., 1988; Centurion et al., 2003; Love et al., 2005). Portable instant cool packs that can be activated when required are useful in maintaining tolerable temperatures under field conditions. Further, experimentation can be useful in indicating the optimal diluent solutions that will reduce the impact of seminal plasma (Morato et al., 2003). These strategies may prolong sperm viability in transit to laboratories or provide essential steps in a cryopreservation protocol. Ultimately, effective semen collection, cooling and cryopreservation techniques must be tailored to the species and the field conditions in question in order to preserve the viability of spermatozoa (Plate 60). Great advances have been made in the fields of human andrology and reproductive medicine. In comparison, knowledge of non-human primates is poor and has yet to be prioritized despite a rapid decline in many species and populations (Morrell and Hodges, 1998). A diverse range of assisted reproduction protocols has been established in species that serve as models for humans. For example, the use of frozen–thawed spermatozoa has been shown to successfully produce embryos in vitro and result in offspring after embryo transfer in marmosets (Callitrichidae) and gorillas (Gorilla gorilla gorilla) (Pope et al., 1997). Successful production of offspring from intracytoplasmic sperm injection has also been repeated over the past 25 years. Cryopreservation and the recovery of viable spermatozoa post thaw for AI in non-human primates has proved to be more problematic, although success has been reported in the chimpanzee (Pan sp.) (Gould, 1990), rhesus macaque (Wolf et al., 2004) and marmoset (Callithrix jacchus) (Morrell et al., 1998). The varying structure of primate spermatozoa and their susceptibility to cryoinjury, particularly damage to their DNA (Li et al., 2007), is of concern (Rall, 1993). As with many other wildlife species, primates show significant differences in post-thaw sperm survival between individual males, even though pre-freeze parameters are comparable. Thus, optimizing standardized protocols becomes increasingly difficult. Furthermore, a recent study in rhesus macaques found that while the morphological and motility parameters of spermatozoa post thaw were found to be of acceptable quality by visual assessment, when penetration tests through cervical mucus and hyaluronic acid gel were conducted, spermatozoa were found to have a much reduced efficiency (Tollner et al., 2011). Carnivores employ a wide range of reproductive strategies, often influenced by an equally wide range of ecological and social parameters. Whereas some species have received research attention, there is still a great deal of information yet to be gained when the wide diversity of species and their vulnerability to environmental perturbation are considered, as well as the lack of a domestic model that has received adequate research attention. The domestic dog and cat have provided some clues to the reproduction of their non-domestic relatives, but research on even these models has been limited compared with livestock and laboratory species. Ursids, canids and felids are usually seasonal breeders in nature, but semen can be obtained from zoo-based males of some species throughout the year via electroejaculation (EE) (Howard et al., 1986). In contrast, the spermatozoa of mustelids (Sundqvist et al., 1984) or large canids (Koehler et al., 1998) can only be recovered during their breeding season. Across the carnivore families, onset of puberty is variable in terms of years but is approximately proportional to the total lifespan of the species. A reproductive strategy present in males of most canid species is the presence of the bulbus glandis. This ring of erectile tissue at the base of the penis becomes engorged with blood during copulation and results in the penis being locked in the female’s vagina for a prolonged period, presumably to avoid multiple matings (Kleiman, 1967). In felids, the function of the penile spines may be to enhance the stimulation of females in order to induce ovulation (Aronson and Cooper, 1967). The development of these spines is testosterone dependent and should be evaluated as part of any reproductive examination. The collection and cryopreservation of semen from canids can be readily performed using a number of different techniques that provide good initial sperm motility post thaw (Farstad, 1996, 2000), with the exception of select species, including the red wolf (Canis rufus) (Goodrowe et al., 1998), blue fox (Alopex lagopus) (Farstad, 1998) and grey wolf (Canis lupus) (Leibo and Songsasen, 2002). Spermatozoa are readily collected from felid species using EE (Platz et al., 1978), but cryopreservation techniques yield variable success and few species have been cryobanked with the same success as canids (Howard, 1999) – possibly because felid acrosome membranes appear to be particularly cryosensitive (Pukazhenthi et al., 2001a; Leibo and Songsasen, 2002). Techniques developed for felids have been successfully modified and applied to mustelids (Howard et al., 1991). Ursid sperm have been collected via EE (Howard et al., 2006; Chen et al., 2007; Brito et al., 2010) and cryopreserved successfully, including sperm of the giant panda (Spindler et al., 2004; Álvarez et al., 2008). The six orders of ungulates span a wide range of social structures from solitary to highly gregarious. Some species in the bovid, equid and cervid families exhibit seasonal fluctuations in serum testosterone levels and have shown annual cycles in testicular regression, spermatogenesis and/or some seasonal testicular quiescence. However, animals studied in captivity may show attenuated seasonality due to absent or diminished natural cues. ARTs are powerful tools in supporting and conserving many of the still extant species of this group, particularly in the larger, gregarious species where space constraints are often limiting. Of the many advanced ARTs available today, manipulating the male for semen collection, sample manipulation, storage, transport and insemination is often the simplest and easiest applicable set of techniques for ungulate breeding programmes. Generally, semen collection from ungulates is carried out with anaesthetized animals and EE (Watson, 1978; Howard et al., 1986). Collection by artificial vagina (AV) or rectal stimulation has been successful in some species, given appropriate facilities and training opportunities (Holt, 2001). In some wild ungulates, such as the Indian blackbuck antelope (Antelope cervicapra) (Sontakke et al., 2009), scimitar-horned oryx (Oryx damma) (Roth et al., 1999), Mohor gazelle (Gazella dama mhorr) (Holt et al., 1996a) and gerenuk (Litocranius walleri walleri) (Penfold et al., 2005), semen collection and cryopreservation, and AI using frozen semen, have been successful in producing offspring. Some of the most commonly used cryodiluents (extenders) are Berlinger, TEST, Tris, EQ (equine extender) and yolk-citrate buffers. The cryoprotectants of choice still generally contain 4–8% glycerol or 6–8% DMSO (Holt, 2001). Cryopreservation of elephant sperm has been particularly troublesome, and the low success rate has been a significant barrier to genetic management of this globally managed species. Recently, though, due to breakthroughs including the cushioned centrifuge, careful dilution techniques and the advent of directional freezing, sperm viability post thaw has been achieved (Saragusty et al., 2009). In captivity, several species exhibit a gender skew in offspring, usually towards males. Sex ratios are known to be skewed in wild populations, although evaluation of the factors that cause this is still largely unknown (Berger and Gompper, 1999). Gender skews present specific management challenges in captivity, and while research continues on the potential causes, ARTs provide an opportunity to resolve these challenges. Flow cytometric cell sorting allows for the separation, cryopreservation and use of X versus Y populations of spermatozoa (Johnson, 1995; Johnson and Welch, 1999). As with many animals, most ungulates only have 3–4% difference in the emitted fluorescence of X-bearing and Y-bearing spermatozoa after excitation, and specialized equipment is still required to orient the sperm head correctly to allow sorting. So even obtaining a small sample requires considerable time and resources. Increasingly efficient equipment that is capable of sorting cells by their morphological attributes without the use of stains may enable the potential applications of these techniques to develop in the near future. Knowledge of laboratory rodents such as mice, rats, hamsters, gerbils and rabbits has advanced beyond that of most other species in the field of reproductive biology; from basic physiology to endocrinology, genetic markers, proteomics and molecular signalling. Studies in non-domestic or non-laboratory rodents are still sparse, even though they are one of the most successful and widespread of the mammalian orders. Like many wild mammalian species, most rodents respond to seasonal changes and adjust their reproduction according to photoperiod. Semen collection by EE from woodchucks (Marmot monax), has shown seasonally sperm-rich samples (Concannon et al., 1996). Even in the blind mole rat (Spalax ehrenbergi), fluctuations in testosterone levels and production of spermatozoa are mediated by photoperiod (Gottreich et al., 2000). Colonies of the naked mole rats (Heterocephalus glaber) are well known to use social suppression to control female reproduction. Social suppression of males was reported to be less rigorous where both breeding and non-breeding males produced spermatozoa (Faulkes and Abbott, 1991). The success of the order Rodentia is partially attributed to its rapid and highly adaptable breeding mechanisms (even within one genus such as the mole rats) and the wide range of breeding strategies that are governed by a multitude of environmental and physiological cues. Many muroid rodents have evolved a falciform sperm head, where an apical hook is located on the proximal region of the head (Breed, 2004). This apical hook has been implicated in the facilitation of sperm transport in the female reproductive tract and sperm competition (Roldan et al., 1992). Moore et al. (2002) reported interesting observations of spermatozoa from the common wood mouse (Apodemus sylvaticus), where over hundreds and thousands of spermatozoa formed groups or ‘trains’ that significantly increased sperm motility through the oviduct before dispersing for fertilization. In house mice (Mus musculus and M. domesticus), the apical hook is said to assist the formation of motile sperm bundles (Immler et al., 2007). Phylogenetic differences can also be seen in sperm physiology. Sperm chromatin undergoes reorganization during spermatogenesis into a tightly packed nucleus (Oliva, 2006). The ratios of protamine 1 to protamine 2 that enable the stabilization of the reorganized sperm nucleus are species specific. In the rodent, the ratio varies from 50:1 to 1:1.5 from the Rattus genus to the Mus genus, respectively (Corzett et al., 2002). One of the greatest differences in sperm nuclear density occurs between the X and Y populations within the chinchilla (Chinchilla laniger) ejaculate (Johnson et al., 1989). Unlike many other mammalian species, including other rodents, where the X and Y DNA difference is approximately 3–4% separation, chinchilla spermatozoa show a 7.5% separation between the X and Y bearing spermatozoa detected by Hoechst staining (Johnson and Welch, 1999). These seemingly subtle differences, which cannot be observed by the human eye, have enabled much insight into the evolutionary differences between a highly successful order of animals and other taxa. Because of the challenges in obtaining samples from free-swimming wild cetaceans, limited research has examined seasonal changes in circulating hormone concentrations in male cetaceans. Testosterone has been detected in fatty tissues and faecal samples of free-ranging short-beaked common dolphins (Delphinus delphis) (Amaral, 2010) and North Atlantic right whales (Eubalaena glacialis) (Rolland et al., 2005). Seasonal variation in serum testosterone has been quantified for captive beluga (Delphinapterus leucas), orca (Orcinus orca) and bottlenosed dolphin (Tursiops truncatus) (Robeck et al., 2005a; Robeck and Monfort, 2006; O’Brien et al., 2008). Seasonality is observed in migratory cetaceans (e.g. most whale species) as well as in a number of dolphins and porpoises (Pomeroy, 2011). Whales predominately display a seasonal peak in mating during winter, while dolphins and porpoises are the opposite, with peak breeding observed in summer months (Pomeroy, 2011). Reproduction in whales is generally tied to annual migration patterns, with the exception of orcas, which are known to produce sperm and to breed year round (Robeck and Monfort, 2006). Semen has been collected in several captive cetacean species, predominately using operant training and manual stimulation (Robeck et al., 2004, 2009; O’Brien and Robeck, 2006; O’Brien et al., 2008). EE has been used to collect semen from the bottlenosed dolphin (Fleming et al., 1981). Continual spermatogenesis has been confirmed in captive orca and beluga, but beluga exhibit seasonal variations in ejaculate size and sperm concentration (O’Brien et al., 2008). In captive Pacific white-sided dolphin (Lagenorhynchus obliquidens), spermatozoa have been confirmed only in ejaculates collected between July and October, so provide evidence of a more restricted breeding season than that observed for other cetaceans (Robeck et al., 2009). Similarly, ejaculates are unobtainable or aspermic during winter months in captive bottle-nosed dolphins, which corresponds with seasonal changes in serum testosterone (Schroeder and Keller, 1989). Even though studies on sperm cryopreservation are limited by skill base and access to animals, significant progress has been made in some species. This knowledge has been directly applied to population management. For instance, offspring have been produced after AI using cryopreserved spermatozoa in Pacific white-sided dolphin, beluga and orca (Robeck et al., 2004, 2009, 2010; O’Brien et al., 2008). In the bottlenosed dolphin, live births have resulted from AI using liquid-stored, frozen–thawed and sex-sorted spermatozoa (Robeck et al., 2005b; O’Brien and Robeck, 2006). The most primitive amniotes throughout the animal kingdom are the members of the order Reptilia. Unlike fish and amphibians, this order universally uses internal fertilization and produces offspring independent of water. The differences in reptilian gonads are generally minor, even between oviparous and viviparous species (Mulaik, 1946). The reproductive tract resembles that of eutherians; however, the reproductive ducts are generally similar to those of amphibians (Risley, 1940). The primordial gonads have two defined zones – cortex and medulla. Sexual differentiation occurs with the development of one zone and the atrophy of the other under the influence of sex hormones. Relatively long periods of juvenile hermaphroditism, primarily in Chelonians, an inherent bisexual nature and adult sexual hermaphroditism occur naturally in several reptile species (Dodd, 1968). Semen collection and histological examinations document spermiogenesis and allow semen evaluation of spermatozoa of snakes (Esponda and Bedford, 1987; Zacariotti et al., 2007), chelonians (Al-Dokhi et al., 2007), caimans and common lizards (Tropidurus itambere
1Taronga Zoo, Mosman, New South Wales, Australia;2Taronga Western
Plains Zoo, Dubbo, New South Wales, Australia
Introduction
Primary Challenges when Studying Wildlife Andrology
Availability of animals
Animal and researcher health and safety
Genetic diversity
Environmental conditions
Health and nutrition
Ethics
Natural and Assisted Breeding Strategies
Cryopreservation
Cryotoxicity
Field applications for collecting and cryopreserving semen from free-ranging males
Taxa-specific Notes
Non-human primates
Carnivores
Ungulates
Rodents
Cetaceans
Reptiles
Stay updated, free articles. Join our Telegram channel

Full access? Get Clinical Tree
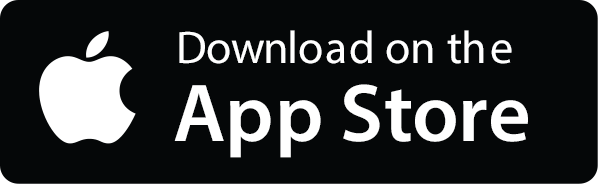
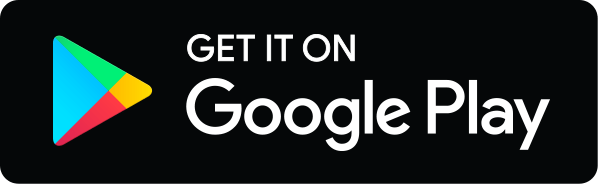