9 Applied Andrology in Chickens and Turkeys Julie A. Long* Modern commercial poultry operations rely heavily on assisted reproduction to maintain the high level of genetic selection that is the backbone of the industry. The growth rate of meat birds and egg production in laying birds are, respectively, the two most economically important traits. Because these traits have negative genotypic and phenotypic relationships, poultry companies either specialize in breeding meat-type birds or have two separate programmes for meat- and egg-producing strains. Primary breeders maintain 50 or more pure lines with relatively small numbers of pedigreed birds. After specific line crosses, the successive generations are multiplied, or produced in greater numbers, until sufficient bird numbers for the final commercial lines are achieved. For the layer and broiler chicken industries, artificial insemination (AI) is used mainly at the primary breeder level. For the turkey industry, all phases of production utilize AI. In this chapter, pertinent aspects of male reproductive biology, semen evaluation and semen storage are reviewed with an emphasis on unique attributes of avian reproductive biology. The reproductive organs of the male bird are completely internalized, unlike the situation in mammals where the testes are externally located. In contrast with the female bird, which has a single functional ovary, avian testes are paired organs anterior to the cranial pole of the kidneys. The male avian reproductive tract consists of the paired testes, epididymal regions and ductus deferens. Avian testes are organized into branching seminiferous tubules containing Sertoli cells, and a relatively small amount of interstitial tissue containing the androgen-secreting Leydig cells. The networks of seminiferous tubules coalesce into a series of ducts, known as the epididymal region, which empties into the ductus deferens. The avian reproductive tract does not contain the characteristically subdivided epididymis found in mammals, in which spermatozoa mature and are stored before ejaculation. Instead, avian spermatozoa mature and are stored in the ductus deferens. Accessory glands found in the mammalian reproductive tract (the seminal vesicle, prostate, etc.) are not present in birds. Males of most avian species do not have a truly intromittent copulatory organ, but rather a phallus on the wall of the cloaca. Mature turkey and chicken spermatozoa exhibit similar morphological features (see extensive review by Jamieson, 2007). The spermatozoa are long and narrow with a vermiform appearance, being 0–5–0.8 mm at the widest point. The acrosome of the turkey spermatozoon is 1.8 mm long, reaching 2.5 mm for the rooster acrosome (Marquez and Ogasawara, 1975). A fibrous, actin-containing, rod-like structure, known as the perforatorium or the acrosomal spine (Etches, 1996), is located between the acrosome and the anterior pole of the nucleus in the spermatozoa of non-passerine birds; no corresponding structure is evident in the subacrosomal space of passerine birds (Pudney, 1995) or mammalian spermatozoa, except in rodents (Baccetti et al., 1980). At 1.0 μm in length, the perforatorium of the turkey and rooster is appreciably shorter than that of the guinea fowl (1.9 μm). The role of this structure in bird spermatozoa is suggested to be skeletal in nature, acting as an inner conical support for the acrosome (Baccetti et al., 1980). The sperm nucleus consists of dense chromatin, forming a concave implantation fossa where it joins the midpiece of the tail. The turkey sperm nucleus is shorter (7–9 μm) than that of guinea fowl or chicken (10–14 μm), and the junction of the nucleus with the midpiece at the neck region is not as conspicuous as in guinea fowl spermatozoa (Thurston and Hess, 1987). For turkey and chicken spermatozoa, the neck region of the midpiece consists of a proximal and an elongated distal centriole, while guinea fowl spermatozoa contain only a single elongated centriole and associated pericentriolar projections (Thurston and Hess, 1987). Cross sections of the centrioles have the typical ‘pinwheel’ arrangement of nine triplet microtubules embedded in a cylindrical, dense wall (Jamieson, 2007). Enveloping the distal centriole and extending to the annulus are 25–30 helically arranged mitochondria (Thurston and Hess, 1987). In contrast to the guinea fowl, the cristae of turkey and rooster sperm mitochondria are parallel to the outer membrane (Jamieson, 2007). The absolute size of the midpiece region and the proportion of the midpiece to the head are greater in turkey than in chicken spermatozoa (Lake and Wishart, 1984). The flagellum ultrastructure consists of the typical 9 + 2 microtubular axonemal complex, but outer dense fibres are absent (Thurston and Hess, 1987). Surrounding the outer doublets is an amorphous sheath (Lake et al., 1968), which defines the principal piece. The portion of the flagellum where the cell membrane is in juxtaposition to the doublet microtubules, without the amorphous sheath, is known as the endpiece (Jamieson, 2007). The flagellum comprises most of the length of the poultry spermatozoon. Turkey and guinea fowl sperm flagellae are usually 60–65 mm long (Marquez and Ogasawara, 1975; Thurston and Hess, 1987), and the flagellum approaches 70 μm in length in rooster spermatozoa (Jamieson, 2007). Thus, the overall length of turkey and guinea fowl spermatozoa (75–80 μm) is also less than that of rooster spermatozoa (90 μm). In all three species, the spermatozoa increase in width from the acrosome to a maximum of 0.5–0.7 μm at the junction of the nucleus with the midpiece. The width then decreases to 0.1–0.2 μm at the end of the flagellum (Thurston and Hess, 1987). Abnormalities in the morphology of poultry spermatozoa have been widely reported; however, there is no uniform classification system for the different types of sperm defects. For example, the term ‘bent sperm’ can refer to a bend in the midpiece or the tail. For this reason, the predominance of one type of defect over others is difficult to discern from the literature. Poultry sperm abnormalities can be broadly categorized according to the anatomical region of the defect (e.g. acrosome, head, midpiece, flagellum). Alkan et al. (2002) published comprehensive drawings of sperm defects in the Bronze turkey (Fig. 9.1), in which 20 sperm defects are illustrated. In general, the presence of 15% or fewer abnormal spermatozoa in a given semen sample does not adversely affect fertility (Clark et al., 1984), although the percentage of abnormal spermatozoa in semen can be as high as 24.1% in turkeys (Nestor and Brown, 1971), 46–48.3% in layer chickens (Siudzińska and Łukaszewicz, 2008; Jarinkovićová et al., 2012) and 42.1% in broiler chickens (Edens and Sefton, 2009), illustrating the need for a common classification system for abnormal poultry sperm morphology. The surface of mammalian spermatozoa comprises a dense coating of carbohydrates forming a 20–60 nm thick glycocalyx (Schröter et al., 1999) that arises from the lipids and proteins forming the plasma membrane. Recently, Long and colleagues have demonstrated that poultry spermatozoa are also covered by carbohydrate residues (Peláez and Long, 2007). The glycocalyx of poultry spermatozoa is extensively sialylated, with sialic acid residues distributed along the entire sperm surface (Plate 12a); it also contains residues of α-mannose/a-glucose, a- and β-galactose, α-fucose, a- and β-N-acetylgalactosamine and N-acetyl-lactosamine, as well as monomers and dimers of N-acetylglucosamine. Non-sialic acid carbohydrate residues are segregated among specific morphological zones. For example, α-mannose and α-glucose were detected only in the plasma membrane overlying the head region, whereas, N-acetyl-glucosamine residues were distributed mainly along the acrosome region. One notable difference between turkey and chicken spermatozoa was the absence of α-galactose residues on the head region of chicken sperm (Plates 12b and c). N-acetylglucosamine dimers and N-acetyl-lactosamine residues were more prevalent in chicken spermatozoa, but N-acetyl-galactosamine residues were more prevalent in turkey spermatozoa. A major role of glycosylation is the formation of specific molecular surfaces that permit specific intermolecular recognition (Ivell, 1999). The sperm glycocalyx is modified extensively during sperm transport and maturation, and represents the primary interface between the male gamete and its environment. While the function of these glycoconjugates is still largely unknown, in the hen, sialic acid has been implicated for both sperm passage through the vagina (Steele and Wishart, 1996) and sperm sequestration in the sperm storage tubules (Froman and Thursam, 1994), and N-acetyl-D-glucosamine is necessary for sperm–egg interaction (Robertson et al., 2000). Long and colleagues have demonstrated that hypothermic storage of turkey semen (4°C) and chicken semen (–196°C) affects the composition of the sperm glycocalyx (Peláez and Long, 2008; Peláez et al., 2011). In particular, terminal sialic acid residues are less abundant after hypothermic storage for both species. Sialic acids act as masking agents on antigens, receptors and other recognition sites of the cell surface, and it appears that the amount of sialic acid in sperm cells is considerably higher than that in somatic cells (Diekman, 2003). Lipids are a major component and integral part of sperm membranes, and are involved with the series of biochemical and functional changes required for fertilization, such as sperm maturation and the acrosome reaction (Bréque et al., 2003). Phospholipids represent the main lipid type found in avian sperm membranes, with phosphatidylcholine comprising ~40% of the total phospholipid content. Poultry spermatozoa also contain a high proportion of polyunsaturated fatty acids in the plasma membrane (Surai et al., 1998). Polyunsaturated fatty acids are classified as n–3 or n–6 based on the location of the last double bond relative to the terminal methyl end of the molecule. In contrast to the plasma membranes of mammalian spermatozoa, which contain mainly n-3 polyunsaturated fatty acids such as docosahexaenoic acid (22:6n-3; DHA), the phospholipids in avian spermatozoa are enriched mainly with n-6 polyunsaturated fatty acids, including arachidonic (20:4n-6; AA) and docosatetraenoic (22:4n-6; DTA) acids. It has been proposed that the functional significance of this n-6/n-3 dichotomy in the fatty acid profiles of avian and mammalian spermatozoa may represent an adaptation to temperature (Kelso et al., 1997). The higher body temperature of birds (41°C) compared with that of mammals (37°C), in tandem with the fact that avian testes are internalized, requires avian spermatozoa to develop and function in a considerably warmer environment. The major poly-unsaturated fatty acid (PUFA) of avian spermatozoa, DTA, displays the same chain length but fewer double bonds than the mammalian PUFA counterpart DHA; this difference in the degree of polyunsaturation provides a means for maintaining the appropriate biophysical properties of sperm membranes at the different body temperatures. An interesting feature of the poultry sperm membrane is that neither chicken nor turkey spermatozoa appear to require capacitation (Howarth, 1970; Howarth and Palmer, 1972; Bednarczyk, 1990). Intramagnal insemination of spermatozoa originating from the testis, epididymis and vas deferens resulted in fertility levels of 85–90%; in contrast, vaginal insemination of testicular spermatozoa resulted in a total absence of fertile eggs (Howarth, 1983). In intramagnal insemination of the female bird, spermatozoa are deposited near the site of fertilization, thus bypassing the vagina and the uterovaginal junction, where sperm motility is essential for transport. In mammalian spermatozoa, physiological capacitation occurs in the female reproductive tract and can be induced in vitro using species-specific conditions. In either circumstance, capacitation results in membrane destabilization, and this facilitates the acrosome reaction. In contrast, the acrosome reaction of chicken spermatozoa may be induced very rapidly in vitro in the presence of the inner perivitelline layer of the egg, or of N-linked glycans (derived from the inner perivitelline layer) and extracellular Ca2+ (Horrocks et al., 2000). More recently, it was shown that the acrosome reaction occurred within the first minute of incubation with the inner perivitelline layer and Ca2+, with the highest percentages of acrosome reacted spermatozoa occurring after 4–10 min of incubation (Lemoine et al., 2008). The absence of a capacitationlike process before the initiation of the acrosome reaction makes sense in the context of the prolonged stay of poultry spermatozoa in the sperm storage tubules of the hen’s reproductive tract, as discussed later in the chapter. Although the gross morphology of turkey and chicken spermatozoa is similar, ultrastructural differences in the midpiece region appear to be indicative of the different biochemical features of spermatozoa from these two species. The main distinction in energy production is that turkey spermatozoa require oxygen to produce ATP, but chicken spermatozoa can metabolize using either aerobic or anaerobic respiration (Sexton, 1974). Both turkey and chicken spermatozoa use the Embden–Meyerhof–Parnas pathway of glycolysis and the Krebs cycle for oxidative metabolism (Kraft et al., 1978). Turkey spermatozoa have a low glycolytic capacity and require the presence of oxygen for optimal ATP production (Lake and Wishart, 1984). Under anaerobic conditions, chicken spermatozoa form lactate from glucose 13 times faster than turkey spermatozoa (Sexton, 1974; Wishart, 1982) owing, in part, to the 20-fold higher activity of lactate dehydrogenase in chicken spermatozoa (McIndoe and Lake, 1973). Profiles of glycolytic enzymes are similar for spermatozoa from both species; however, chicken sperm enzyme activities are twofold to fourfold higher than those of turkey sperm, with glycerophosphate mutase and lactate dehydrogenase activities 9.5 and 41 times greater, respectively (Wishart and Carver, 1984). Glutamate and glycine are not used by either species as oxidizable substrates, while acetate and succinate increase the rate of oxygen consumption in chicken spermatozoa only (Sexton, 1974). At the physiological temperature of 40°C, chicken spermatozoa maintain high ATP levels and fertilizing potential for up to 3 h by the aerobic or anaerobic metabolism of glucose (Wishart, 1982). When chicken and turkey spermatozoa were incubated aerobically without glucose, the rate of ATP hydrolysis (a measure of energy utilization) and the rate of oxygen consumption (a measure of the rate of energy production) decreased by 75–80% as the temperature was lowered from 40 to 5°C (Table 9.1). At each temperature, decreases in the rates of oxygen consumption and ATP hydrolysis were equivalent, suggesting a coupling of ATP production to oxygen metabolism at all temperatures, as the sperm ATP levels remained constant (Wishart, 1984). This was not the case with turkey spermatozoa, which, under conditions of anaerobic glycolysis at temperatures between 5 and 40°C, contained <5% of the ATP concentrations exhibited under aerobic conditions (Table 9.1). After storing chicken semen at 5°C under anaerobic conditions for 48 h, no differences in the activity of the glycolytic enzymes aldolase or lactate dehydrogenase were evident, whereas the activities of the two Krebs cycle enzymes fumerase and aconitase were lower in stored than in fresh spermatozoa (Buckland, 1971). It has been reported that semen ejaculated from the broiler rooster is in an anaerobic state, as there was no free oxygen available in undiluted semen (Parker and McDaniel, 2006). Spermatozoa recovered from different anatomical regions of the male layer rooster reproductive tract had markedly different degrees of sperm motility that corresponded to the degree of sperm maturation: testicular spermatozoa demonstrated the lowest motility, followed by a gradual increase in sperm motility from the epididymal region to the distal ductus deferens (Ahammad et al., 2011). There was no difference in sperm motility between spermatozoa obtained from the distal ductus deferens and ejaculated spermatozoa. Spermatozoa from some avian species exhibit a unique sperm motility phenomenon of reversible temperature-dependent immobilization (Ashizawa et al., 2010) in which, in simple salt solutions, spermatozoa become immotile at body temperature (40–41°C) but regain motility when the temperature is lowered to 30°C or by the addition of Ca2+ at 40°C (Munro, 1938; Wishart and Ashizawa, 1987; Ashizawa et al., 1989; Thomson and Wishart, 1991; Ashizawa et al., 1994). This pattern of temperature-dependent motility occurs in chicken, duck and copper pheasant spermatozoa, but occurs only partially in turkey and not at all in quail or Houbara bustard spermatozoa (Wishart and Wilson, 1999; Ashizawa et al., 2010). Turkey sperm motility was only partially inhibited at 40°C, while quail and bustard spermatozoa showed no reduction in the percentage of motile spermatozoa at 40°C (Wishart and Wilson, 1999). Ca2+ restored the motility of chicken and duck spermatozoa at 40°C and also released the partial inhibition of turkey sperm motility; however, Ca2+ had no apparent effect on the proportion of motile bustard spermatozoa and completely inhibited quail sperm motility at 40°C (Wishart and Wilson, 1999). The axoneme and/or accessory cytoskeletal components appear to be directly involved in the temperature-dependent regulatory system, because the motility of de-membranated spermatozoa is similar to that of intact spermatozoa, being negligible at 40°C and restored at 30°C (Ashizawa et al., 2000). This temperaturedependent motility has been shown to be a function of intracellular Ca2+ content; intracellular levels of Ca2+ were reduced at the higher temperatures, where spermatozoa were immotile, and increased as the temperature was lowered from 40 to 30°C (Thomson and Wishart, 1991). Further, the temperaturedependent retention of Ca2+ was a function of the rate of Ca2+ efflux rather than influx, and involved a Ca2+ ATPase that was relatively inactive at 30°C, but active at 40°C (Thomson and Wishart, 1991). Chicken sperm motility was inhibited by loading with an intracellular Ca2+ chelator, and subsequently reactivated by the addition of excess Ca2+ to the medium (Ashizawa et al., 1994). Not all avian species demonstrate this temperature-dependent sperm motility phenomenon. In the chicken and turkey, a sperm motion attribute known as sperm mobility has been positively correlated with fertility in poultry (Donoghue et al., 1999; Froman et al., 1999; King et al., 2000b). Sperm mobility is defined as the net movement of a sperm cell population against resistance at body temperature (Froman and Kirby, 2005), and is assayed by measuring the absorbance of a solution of Accudenz (a cell separation medium) following sperm ‘swim down’ from an overlaid sperm suspension (Froman and Feltmann, 1998). Absorbance is proportional to the number of spermatozoa within the sample that have a straight-line velocity of, or greater than, 30 mm/s (Froman and Feltmann, 2000). Simply put, all mobile sperm are necessarily motile, whereas motile sperm are not necessarily mobile (Froman and Kirby, 2005). When the sperm mobility assay was applied to populations of randomly bred broiler roosters, the phenotype varied among males (Froman et al., 1997), was independent of age (Froman et al., 1999) and determined male fecundity (Froman and Feltmann, 1998). Subsequently, the heritability of this quantitative trait was estimated (Froman et al., 2002), and it was shown that mitochondrial dysfunction accounted for the phenotypic variation (Froman and Kirby, 2005; Froman et al., 2006). In the turkey, the high sperm mobility phenotype was characterized by a straightline velocity of 45.5 μm/s and an average path velocity of 60.3 μm/s, compared with 28.8 and 45.1 μm/s, respectively, in males exhibiting the low sperm mobility phenotype (Donoghue et al., 1998). Like the sperm mobility phenotype established for the chicken, that in turkeys also varies characteristically among males (King et al., 2000a), is independent of age (Holsberger et al., 1998) and influences paternity (Donoghue et al., 1999). The turkey sperm mobility phenotype does not, however, predict the outcome of sperm function after 24 h of storage at 4°C, as sperm mobility values decline to similar levels irrespective of the male phenotype (Long and Kramer, 2003). Finally, male turkeys characterized as either high or low sperm mobility phenotype exhibit striking differences in the content of carbohydrates in the glycocalyx, including mannose/glucose, N-acetyl-glucosamine and N-acetylgalactosamine (Peláez and Long, 2008). The components of poultry seminal plasma are thought to be derived from the extragonadal duct system within the epididymis, primarily the proximal efferent duct, which appears to be where spermatozoa are mixed with secretions as they are concentrated (Hess et al., 1976; Aire, 1979; al-Aghbari et al., 1992). During natural mating in chickens, a lymphlike, transparent fluid is mixed with the ejaculate. This transparent fluid, derived from the paracloacal vascular bodies (Fujihara, 1992), is chemically distinct from deferent duct fluid (Lake, 1966, 1984). In turkeys, a frothy fluid is secreted from the triangular fold of the cloaca and mixed with the ejaculate during copulation (Fujihara et al., 1985). The chemistry of the frothy fluid resembles that of blood plasma, rather than that of seminal plasma (Fujihara and Nishiyama, 1984). As outlined below, the biochemistry of seminal plasma differs markedly from that of blood plasma. Thus it is not surprising that prolonged exposure of turkey spermatozoa to this frothy fluid adversely affects sperm morphology (Fujihara et al., 1987). Compared with mammalian livestock, data on the content of the principal inorganic ions (Na+, K+, Cl–, Ca2+, Mg2+) in the seminal plasma of chicken and turkey semen are sparse, and most reports date from the 1960s (Table 9.2). As in mammals, a reciprocal relationship exists between the amounts of Na+ and K+ in avian spermatozoa and seminal plasma, with seminal plasma containing higher Na+ and lower K+ concentrations than spermatozoa and, conversely, spermatozoa containing lower Na+ and higher K+ than seminal plasma. Chicken seminal plasma, though, contains more Na+ and less K+ than has been reported for ram, bull or human seminal plasma (Quinn et al., 1965; Mann and Lutwak-Mann, 1981). Newer results reported by Karaca et al. (2002) for inorganic ion concentrations in chicken seminal plasma fell within similar ranges to those in older reports (Hammond et al., 1965; Quinn et al., 1965; El Jack and Lake, 1969; Lake, 1971; Lake and Wishart, 1984), although a recent paper where semen from aged broilers was evaluated after an induced moult reported markedly lower concentrations of Na+, K+ and Ca2+ in seminal plasma (Khan et al., 2012) than did previous reports (Table 9.2). Fewer data are available for turkey seminal plasma ion concentrations than for those of chickens, but all reports indicate appreciably higher concentrations of K+ and lower concentrations of Cl– in turkey seminal plasma (Brown et al., 1959; Cherms, 1967; Graham, et al., 1971; Lake and Wishart, 1984) than in chicken seminal plasma (Table 9.2). Glutamic acid is the principal amino acid in poultry seminal plasma, and is thought to serve as the main anion in place of Cl– (El Jack and Lake, 1969). Lake and McIndoe (1959) reported that glutamic acid comprises 90% of the free amino acids found in chicken seminal plasma; similarly, a high proportion of glutamic acid is found in turkey seminal plasma (Ahluwalia and Graham, 1966b; Graham et al., 1971). The concentration of glutamic acid in poultry seminal plasma is ten times the total amino acid concentration in bull semen (Lake and McIndoe, 1959), and several 100 times greater than that found in blood plasma (Lake and Wishart, 1984). The functional significance of glutamate, other than in establishing osmolality, is unclear, as poultry spermatozoa lack adequate metabolic capabilities to use glutamate as an energy source (Etches, 1996). Of the 20 recognized amino acids (excluding glutamic acid), arginine, asparagine, threonine and glycine are the most prevalent in chicken seminal plasma, whereas arginine, asparagine, aspartic acid and serine are the most prevalent in turkey seminal plasma (Ahluwalia and Graham, 1966b). Graham et al. (1971) determined that the semen collection frequency in turkeys influenced the amount of free amino acids in seminal plasma; the levels of most amino acids (except for glutamic acid) were higher when semen was collected at 2 day intervals than at 4 or 7 day intervals. It appears that the seminal plasma amino acid profile also varies among genotypes. Froman and Bernier (1987) identified a heritable reproductive disorder in the male rooster in which spermatozoa degenerate prematurely. The inheritance of this trait was attributed to a single dominant gene (Froman et al., 1990), and mutant roosters were characterized by a reduced surface-to-volume ratio within their proximal efferent ducts (Kirby et al., 1990) and a reduced level of glutamic acid in seminal plasma compared with normal roosters (al-Aghbari et al., 1992). Carnitine plays a vital role in transporting long chain fatty acids across the inner mitochondrial membrane to produce energy through β-oxidation (Bremer, 1983), and may also function as an antioxidant to scavenge free radicals (Agarwal and Said, 2004). Both carnitine and acetyl carnitine are present in poultry seminal plasma (Golan et al., 1982; Lake and Wishart, 1984). While the levels of carnitine (3.2 mmol) and acetyl carnitine (2.1 mmol) are comparable to those reported for mammalian semen (Mann and Lutwak-Mann, 1981), turkey seminal plasma contains about half (1.7 and 0.64 mmol, respectively) of the levels found in chicken seminal plasma (Lake and Wishart, 1984). Fructose is the principal unbound sugar of seminal plasma for a number of mammalian species (Mann and Lutwak-Mann, 1981), but only trace amounts of fructose are found in poultry seminal plasma (Kamar and Rizik, 1972; Lake and Wishart, 1984; Garner and Hafez, 1993). A few older reports document glucose levels of 80–88 mg/dl in chicken seminal plasma (Mann, 1964; Hammond et al., 1965), though only 3.1 mg/dl was detected by Ahluwalia and Graham (1966a); rather, the primary carbohydrate isolated from chicken seminal plasma in that report was inositol (20.4 mg/dl), along with low amounts of glycerol (2.8 mg/dl). Wishart (1982) concluded that chicken and turkey semen did not contain glycolytic substrates, as no lactate was produced when semen was diluted in buffer without glucose and incubated under anaerobic conditions. A more recent report documented the presence of free oligosaccharides in human seminal plasma – the first report for any species (Chalabi et al., 2002); however, the function of these oligosaccharides remains unknown. The lipid composition of poultry seminal plasma has been investigated much more recently than the free carbohydrate or amino acid contents, and differs considerably from the lipid content of poultry spermatozoa. The total lipid content of poultry seminal plasma generally has a lower proportion of phospholipids than are found in spermatozoa and, within the phospholipid class, a lower proportion of phosphatidylcholine (Douard et al., 2000; Zaniboni and Cerolini, 2009). In contrast to spermatozoa, there are significant amounts of cholesterol esters and triglycerides in seminal plasma (Douard et al., 2000). There appear to be species-specific differences in seminal plasma lipids among turkeys, layer chickens and broiler chickens (Table 9.3), a finding reinforced by the results from one laboratory that compared the seminal plasma lipid profiles from all three poultry groups (Cerolini et al., 1997); in this study, the mean total lipid content of turkey, layer chicken and broiler chicken seminal plasma at peak semen production was 1.3, 1.5 and 0.3 mg/ml, respectively. Age of the male may influence the lipid content of seminal plasma, as the total lipid content increased from 0.14 to 1.1 mg/ml in broiler males aged 25 and 60 weeks, respectively (Kelso et al., 1996). Two of the six non-cyclic phosphodiesters are found in semen: glycerol phosphorylcholine in mammalian seminal plasma and serine ethanolamine phosphodiester in avian seminal plasma (Burt and Ribolow, 1994). In chicken seminal plasma, serine ethanolamine phosphodiester was shown to be 23–26% of the total observable phosphate in three males, but in two other males, it was only 7–9% (Burt and Chalovich, 1978). Further, turkey semen collected in the spring showed ‘substantial levels’ of serine ethanolamine phosphodiester (Burt and Ribolow, 1994), which decreased as males began to stop semen production in late summer (Ribolow and Burt, 1987). Phosphodiesters are a class of compounds that may function as lysophospholipase inhibitors in semen, a role that would decrease the rate of membrane phospholipid turnover and lead to a net sparing of phospholipids (Burt and Ribolow, 1994). Chicken seminal plasma contains 52–77% protein (Blesbois and Hermier, 1990) or 2.0–2.4 g/dl (Harris and Sweeney, 1971; Amen and Al-Daraji, 2011), while turkey seminal plasma averages 1.8 g protein/dl (Thurston et al., 1982). Many proteins in poultry seminal plasma have been identified as enzymes. Autoproteolytic activity has been detected in the seminal plasma of the domestic chicken (Droba, 1986), and serine proteinase inhibitors are present in chicken seminal plasma, with their proposed function being the inactivation of acrosin that is released from dead or damaged spermatozoa (Lessley and Brown, 1978). Turkey seminal plasma contains a unique serine proteinase not found in the chicken or guinea fowl, and the amidase activity of turkey seminal plasma is 23–28 times greater than for chicken or guinea fowl (Thurston et al., 1993). The turkey seminal plasma serine proteases were found to be distinct from the sperm enzyme acrosin (Thurston et al., 1993). The biological roles of these enzymes in birds are unknown as, unlike in mammals, turkey seminal plasma serine proteases cannot participate in semen liquefaction or coagulation because these processes do not occur in birds (Holsberger et al., 2002). More recent research has shown that turkey seminal plasma contains at least three trypsin inhibitors, providing further evidence for increased activities of serine proteinases as a unique characteristic of turkey seminal plasma (Kotłowska et al., 2005). A number of glycosidases are present in poultry seminal plasma, including β-N-acetylglucosaminidase, the α and β forms of mannosidase, galactosidase and glucosidase, and β-glucuronidase (Kannan, 1974; McIndoe and Lake, 1974; Droba and Droba, 1987; Droba and Dzugan, 1993), which hydrolyse a variety of compounds containing terminal, non-reducing carbohydrate residues. Among the glycolytic enzymes present in whole semen, β-N-acetylglucosaminidase was the most active, with α-mannosidase ranking next in activity, whereas the activities of β-mannosidase, both forms of galactosidase and glucosidase, and β-glucuronidase, were almost negligible (Kannan, 1974). The activity of acid phosphatases, which catalyse the hydrolysis of phosphate esters, is distinctively high in human and poultry seminal plasma (Wilcox, 1961; Bell and Lake, 1962; Mclndoe and Lake, 1974; Hess and Thurston, 1984; Dumitru and Dinischiotu, 1994). The presence of several phospholipases have been confirmed in turkey seminal plasma, including phospholipase A2, phospholipase A1 and lysophospholipase; these have been linked with sperm membrane phospholipid loss during hypothermic semen storage (Douard et al., 2004). Several antioxidant enzymes are found in poultry seminal plasma, including glutathione peroxidase, superoxide dismutase, paraoxonase, arylesterase and ceruloplasmin (Surai et al., 1998; Khan et al., 2012). The relative activity of superoxide dismutase in the seminal plasma of five different avian species has been ranked as guinea fowl > chicken > goose > duck > turkey; conversely, glutathione peroxidase activity is the highest in the turkey and lowest in the duck and goose (Surai et al., 1998). A potent phospholipid mediator, platelet activating factor (PAF), has been detected in mammalian spermatozoa from several species (Kumar et al., 1988; Kuzan et al., 1990; Minhas et al., 1991). Platelet activating factor acetylhydrolase, which inactivates PAF, may represent a mechanism for regulating sperm-derived PAF and has been detected in chicken seminal plasma, although the acetylhydrolase activity was lower in rooster seminal plasma than in that of the bull, stallion and rabbit (Hough and Parks, 1994). Transport proteins, such as albumin, comprise the majority of the protein content of poultry seminal plasma (Blesbois and Caffin, 1992). For example, over 60% of the protein spots from 2D SDS-PAGE gels for chicken and turkey seminal plasma have been identified as serum albumin precursor, ovalbumin or ovotransferrin (J.A. Long, unpublished data). Ovotransferrin is mainly known as an iron-binding glycoprotein found in the egg white (Kurokawa et al., 1999), but it has recently been shown to be a multifunctional protein with a major role in avian natural immunity (Giansanti et al., 2012). Ovalbumin also has been traditionally associated with the egg white and development of fertilized eggs (Smith and Back, 1962; Sugimoto et al., 1999). Serum albumin is the main protein of blood plasma, in which a high binding capacity for water, Ca2+, Na+, K+ and fatty acids contributes to the main function of regulating the colloidal osmotic pressure of blood. It seems highly likely that serum albumin precursor has a similar role in seminal plasma. High density lipoproteins (HDLs) have been detected in chicken seminal plasma; however, the low concentration and the lack of larger lipoproteins suggest that seminal HDLs originate from blood plasma and pass through the blood–testis barrier (Blesbois and Hermier, 1990). As cholesterol carriers, HDLs allow cellular cholesterol efflux (Eisenberg, 1984), and in large amounts could impair the stability of the poultry sperm membrane. Triglyceride-rich lipoproteins (very low, intermediate and low density lipoproteins) were not detected in chicken seminal plasma (Blesbois and Hermier, 1990), but Long and colleagues (unpublished) have identified apovitellenin-1, a low density lipoprotein (LDL), in chicken seminal plasma (Table 9.4). Additionally, these workers identified nine other binding/transport proteins in chicken and/or turkey seminal plasma; vitamin D-binding protein precursor, beta-2-glycoprotein 1, alpha-1-acid glycoprotein 2-like, phosphatidylethanolamine-binding protein 1, nuclear transport factor 2-like protein, calmodulin, retinol binding protein, transthyretin and gamma-glutamyltranspeptidase 1 (Table 9.4). To date, they have identified 29 proteins in the seminal plasma of these two species, with five proteins in common (Table 9.4). One protein common to both chicken and turkey seminal plasma is PIT54 protein precursor; PIT54 is a soluble member of the family of scavenger receptor cysteine-rich proteins whose gene exists only in birds (Wicher and Fries, 2006), and may have an antioxidant role in seminal plasma.
Beltsville Agricultural Research Center, US Department of Agriculture
Agricultural Research Service, Beltsville, Maryland, USA
Introduction
Male Reproductive Biology
Sperm Morphology and Physiology
Morphology
Sperm membrane carbohydrates and lipids
Sperm metabolism and motility
Seminal Plasma
Electrolytes
Amino acids and peptides
Carbohydrates and polyols
Lipids and phosphodiesters
Enzymes and other proteins
Semen Collection and Evaluation
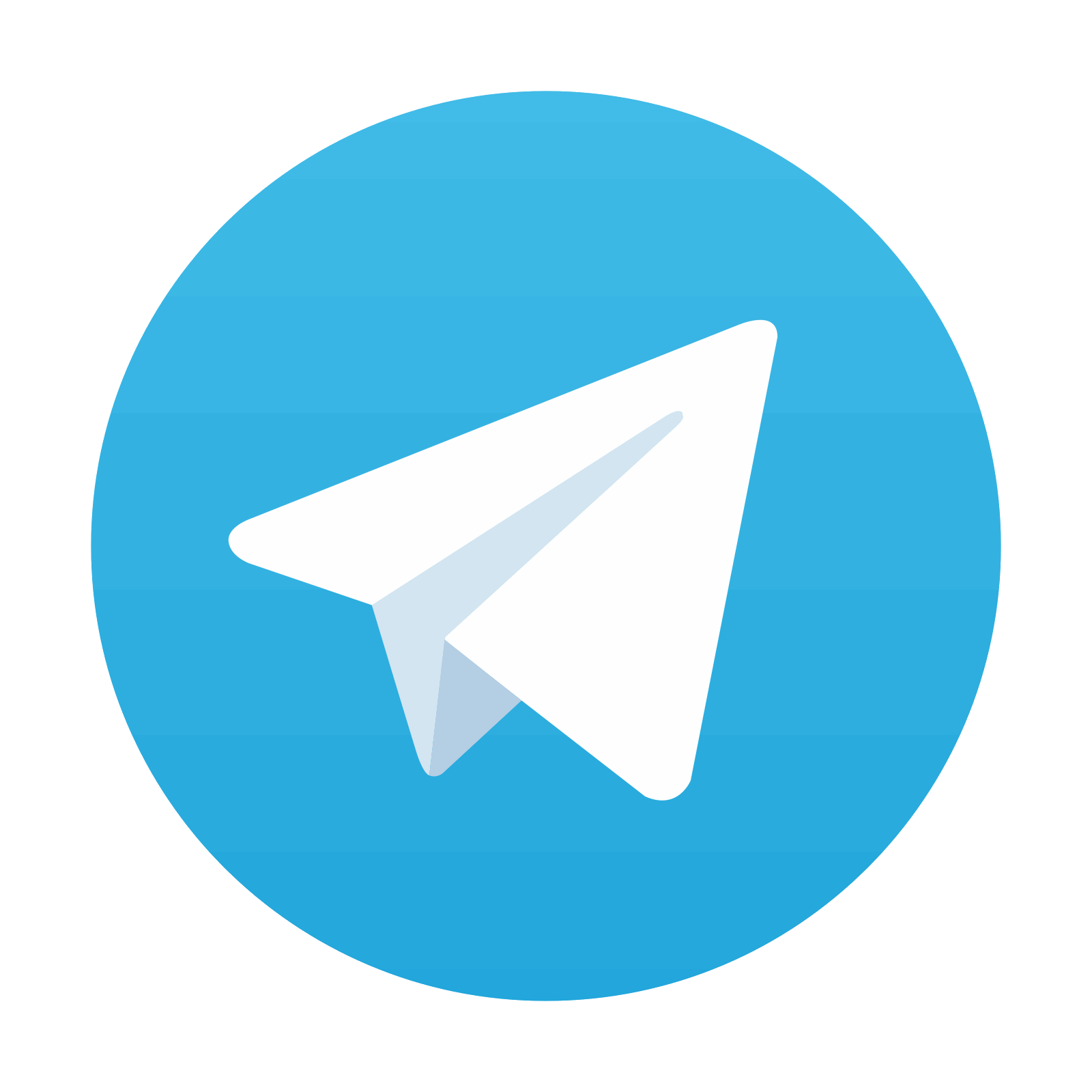
Stay updated, free articles. Join our Telegram channel

Full access? Get Clinical Tree
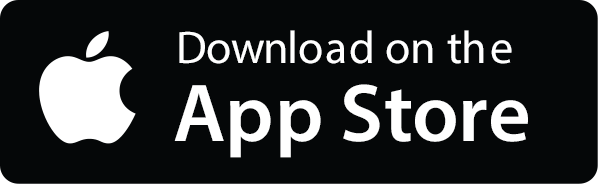
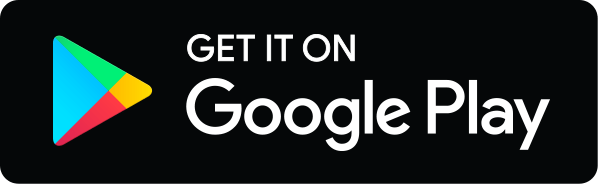