Chapter 29 Antiinflammatory Drugs
The Pathophysiology of Inflammation
Inflammation can occur in any vascularized tissue. Initiated as a protective mechanism intended to remove the underlying cause, be it chemical, physical, or biological, the acute inflammatory process facilitates return of the inflamed tissue to normal function.1,2 With time, however, its unfettered actions can lead to chronic inflammation, which can contribute to harm to the patient. The sequelae of inflammation are manifested as five cardinal signs: redness, heat, swelling or edema, pain, and loss of function. The initial response to vascular damage is vasoconstriction of small vessels in the area of injury, which serves to control hemorrhage within 5 to 10 minutes; however, vasodilation and increased vascular permeability of small venules are evident. Leukocytes, platelets, and erythrocytes in the injured vessels become “sticky,” adhering to the endothelium. Leakage of cells and plasma-derived protein-rich fluid is followed by platelet aggregation and fibrin formation. Initially, the predominant cell type infiltrating damaged tissues is the polymorphonuclear leukocyte (PMN), in part because it predominates in circulation. These cells provide a protective response by removing chemicals or other materials that may have initiated the inflammatory response. As the short-lived PMNs die, macrophages become the predominant cell type. The migration and concentration of PMNs to the site of injury is facilitated by chemical mediators that act as chemotactic agents. As PMNs die, the contents of the lysed cells accumulate to form the component of inflammatory exudate commonly referred to as pus.
The Role of Chemical Mediators in the Inflammatory Response
Released mediators perpetuate the inflammatory response (Table 29-1 and Figures 29-1 and 29-2) and are responsible for the clinical signs associated with inflammation, including pain and fever.3 Mediators (see Table 29-1) are derived from both the cells (both preformed and formed in situ) and fluid that reach the site of tissue damage by way of the bloodstream. Although quantitative differences between species and tissue concentrations of the mediators vary, the effect on and role in the pathophysiology of inflammation that each mediator has are predominantly the same. Leukocytes are a rich source of a variety of chemical mediators of inflammation. These cells, as well as cells of the injured tissues (either at the time of damage or after subsequent damage), perpetuate the inflammatory response and become potential targets of antiinflammatory drugs. Preformed mediators include those located in granules (e.g., histamine and serotonin) and lysosomes and other enzymes. Their release occurs in the earliest stages of inflammation, to be followed by the release of mediators formed in situ. This latter group includes products of arachidonic acid (AA) metabolism, including eicosanoids (prostaglandins [PGs], leukotrienes, and related compounds), and platelet-activating factor, as well as oxygen radicals, and cytokines. The role each of these mediators varies. Although most mediators are proinflammatory in action, antiinflammatory mediators also are formed. Examples include lipoxins, epoxyeicosatrienoic acids (EETs), hydroxyeicosatetranoic acids (HETEs), and epoxyoctadecenoic acids (EpOMEs), selected cytokines, and possibly cyclooxygenase-2 (COX-2)–generated PGs formed in later stages of inflammation (see Table 29-1).1,2 The induction of genes responsible for the generation of proinflammatory mediators is an emerging target of interest for antiinflammatory drugs. Among the inducible transcription factors that control inflammatory gene expression, nuclear factor NFκB is of particular interest because of its ability to coordinate soluble proinflammatory mediators such as cytokines and chemokines as well as leukocyte adhesion molecules. Normally, this factor is trapped in the cytosol by an inhibitory protein that hides the signal necessary for nuclear localization. However, in the face of proinflammatory cytokines (e.g., tumor necrosis factor-alpha [TNF-alpha], interleukin-1 [IL-1]), the inhibitory protein is phosphorylated and degraded, thus allowing movement of NFκβ to the nucleus.4 The role of matrix metalloproteinases (MMPs) in the inflammatory process is being increasingly recognized.5 The extraceullar matrix has long been recognized as a substrate for these proteolytic tissue-remodeling enzymes. However, other molecules serve as substrates, including cytokines and chemokines. As such, MMPs may act to control or perpetuate inflammation, and the sequelae of their inhibition may lead to adverse reactions as well as therapeutic benefits.
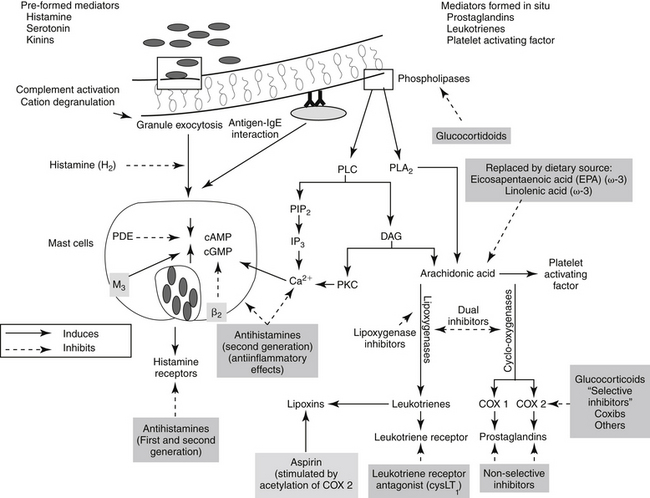
Figure 29-1 Leukocytes are an important source of inflammatory mediators that perpetuate the inflammatory response. Cellular mediators of inflammation include those preformed in granules or lysosomes, such as histamine and serotonin, and those formed in situ from arachidonic acid released by phospholipases in the cell membrane. Muscarinic receptors (M3) stimulate, and β-adrenergic receptors (2) inhibit, inflammatory mediator release. Although the phagocytic cell is intimately involved in the inflammatory reaction, it is not the only cell type capable of generating mediators of inflammation. The mediators of cellular origin interact with plasma-derived mediators, further compounding the response. Drugs used to control inflammation generally target specific mediators (see boxes) (see Table 29-1). cAMP, Cyclic adenosine monophosphate; cGMP, cyclic guanosine monophosphate; DAG, diacylglycerol; IP3, inositol triphosphate; PAF, platelet-activating factor; PDE, phosphodiesterase; PIP2, phosphatidylinositol; PLA2, phospholipase A2; PLC, phospholipase C.
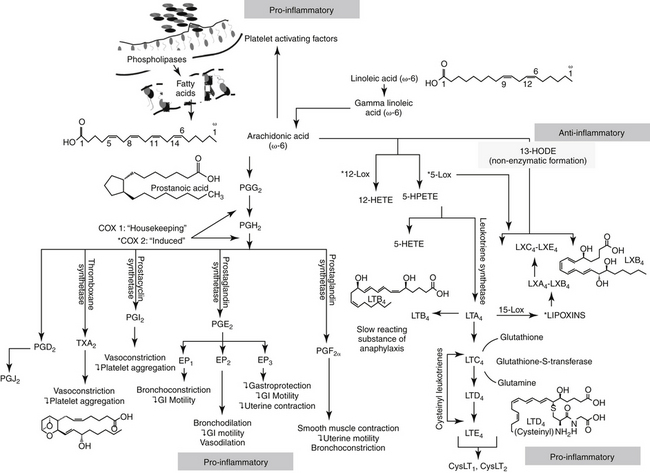
Figure 29-2 The release of arachidonic acid from the phospholipids in cell membranes leads to a cascade of events resulting in the formation of inflammatory mediators. The family of cyclooxygenases (COX) results in the formation of constitutive prostaglandins (COX-1) and inducible prostaglandins (COX-2). The inducible prostaglandins contribute to all cardinal signs of inflammation. Selectivity of nonsteroiodal antiinflammatory drugs (NSAIDs) for COX-2 ultimately may result in marked safety of the NSAIDs. Lipoxygenases result in the formation of leukotrienes, which, along with their precursors, are potent inflammagens in a number of tissues. However, among these products are antiinflammatory lipoxins. The cysteinyl leukotrienes play a major role in type I hypersensitivity and potentially in chronic inflammatory diseases as well (see Chapter 19). Platelet-activating factor also is a potent inflammagen, although some of its actions may be mediated through leukotriene activity.
Acute inflammation can cause severe organ or life-threatening damage. Monocytes, which follow neutrophils to the site of inflammation several hours later, release collagenases and elastases, softening local tissues. Interleukin release draws fibroblasts to the area, which in turn deposit collagen at the site. The collagen is gradually remodeled, and new blood vessels continue to form until oxygen tension is normal. If the cause of inflammation is removed, healing is complete. Failure to remove the inciting cause leads to persistent, chronic inflammation. Pharmacologic control of inflammation is oriented toward preventing the release of various chemical or plasma mediators, inhibiting their actions and treating pathophysiologic responses to them. Drugs useful for modulating the activity of chemical mediators derived from cells, plasma, or both are summarized in Table 29-1. Among the most important and frequently used drugs are the nonsteroidal antiinflammatory drugs (NSAIDs), all of which control fever; pain; and, to varying degrees, inflammation. A number of drugs and novel ingredients target inflammation particularly associated with specific conditions including osteoarthritis and septic shock; these are discussed separately. Glucocorticoids engage such a substantial role in the contribution, prevention, and treatment of inflammation that their discussion warrants a separate chapter.
Shared Pharmacology of Nonsteroidal Antiinflammatory Drugs
Chemistry
Although NSAIDs have been variably defined, the name is used to describe compounds that are not steroidal and that suppress inflammation. Generally, the classification is restricted to those drugs that inhibit one or more steps in the metabolism of AA, generally at the COX side of the cascade.6 The NSAIDs vary in their ability to influence inflammation. The mechanism of action of some of these drugs is not limited to inhibition of AA metabolism.7
Aspirin, one of the earliest components of herbal therapy, is the progenitor NSAID, and terms such as aspirinlike and aspirin and related drugs have been used to refer to NSAIDs,6 although this older terminology may become obsolete with approval of newer NSAIDs. Structurally, NSAIDs can be broadly classified as either the salicylate or carboxylic acid derivatives, including the indoles (indomethacin), propionic acids (carprofen, ibuprofen, and naproxen), fenamates (mefenamic acid), oxicams (piroxicam), and pyrazolones or enolic acids (phenylbutazone and dipyrone)(Figures 29-3 and 29-4).6 Functionally, NSAIDs increasingly are being categorized by the enzymes they target: conventional (COX-1 and COX-2); newer COX-2–selective (COX-1 protective), which includes the coxibs as well as similarly acting drugs; more appropriate and dual inhibitors (tepoxalin) (see Figure 29-2). The coxibs include the sulfones and the sulfonamides. However, the term coxib refers to a chemical structure (see Figure 29-4) and not pharmacologic action and should not be used to refer to all drugs that might preferentially target COX-2 compared with COX-1. The coxibs are not arylamine sulfonamides (see Chapter 4), and therefore allergic reactions typical of sulfonamides antibiotics (see Chapter 7) may not occur. Nonetheless, several of these drugs are associated with keratitis sicca or suppression of thyroid gland activity.
Mechanism of Action
Prostaglandins
Prostaglandin Formation
Eicosanoids, such as PGs and leukotrienes, are 20-carbon chain derivatives of cell membranes. Eicosanoids are potent mediators of inflammation and are particularly important in the later stages.3,8 These compounds are synthesized when oxygen reacts with the polyunsaturated fatty acids of cell membrane phospholipids (see Figures 29-1 and 29-2). The most important of these fatty acids is AA, an omega-6 fatty acid, which is released into the cell from phospholipids of the damaged cell membranes. Release reflects activation of phospholipase A2 (dependent on calcium and calmodulin) located in the cell membrane.9 Once inside the cell, AA serves as a substrate for enzymes that generate intermediate and ultimately the final eicosanoid end product.8,10 The formation of lipoxygenases and their subsequent inhibition is discussed under “Miscellaneous Antiinflammtories.” COX PG synthase or prostaglandin H (PGH) synthase, located in all cells except mature red blood cells, add oxygen to AA, generating unstable PG endoperoxides (PGG2). Subsequent peroxidase reactions convert PGG2 to PGH2, the precursor of all PGs and thromboxane. The final PG end product depends on the presence of specific isomerase reductase or synthetase enzymes, some of which may be inducible.8,9
The direct effects of PGs are mediated through cell membrane–spanning G protein receptors located on target tissues and cells. At least nine subtypes have been identified, corresponding to each of the COX metabolites: DP1-2 (PGD2), EP1-4 (PGE2), FP A, B (PGF2α), IP (PGI2), and TP α, β (TXA2) (G&G);11 another four have been characterized for PGE2, opening the potential for selective drug activity.9 In addition to their receptor interactions, PGs also act indirectly by enhancing other mediators such as histamine and bradykinin.
The role of PGs in normal physiology as well as in disease might best be understood by considering them as protective in nature, even those associated with inflammation. Their formation is mediated by one of at least two isoforms of cyclooxygenases (see Figures 29-1 and 29-2), located on different genes in humans.12–14 COX-1, the “housekeeping” isoform,14 mediates the formation of constitutive PGs produced by many tissues, including gastrointestinal cells, platelets, endothelials cells, and renal cells. PGs generated from COX-1 are constantly present, providing homeostasis through a variety of normal physiologic effects. These include protection of the gastrointestinal mucosa, hemostasis, and the kidney when subjected to hypotensive insults. COX-2 is the product of an “immediate-early” gene that is rapidly inducible and tightly regulated.14 Regulation of COX expression is complex, with more sites present on the COX-2 compared with the COX-1 gene. Its expression is tightly restricted (but not absent) under basal conditions, but it is dramatically upregulated in the presence of inflammation or other diseases.9 Diseases associated with increases include (in humans) rheumatoid arthritis, seizures, ischemia, and (posttranslational) cancer.9 Proinflammatory cytokines such as TNFα and the interleukins stimulate the expression of COX-2 in many cell types, such as synovial cells, endothelial cells, chondrocytes, osteoblasts, and monocytes and macrophages.14 COX-2 catalyzes the formation of inducible PGs, which are needed only intermittently or under specific situations.12,13,15,16 A third COX isoform has been suggested, but it appears to be a variant of COX-1. Originally identified in high concentrations in canine cerebral cortex (hence the term used by some investigators, “canine COX-3”),17 it is inhibited by acetaminophen (and other NSAIDs). Its inhibition may influence central analgesic effects of NSAIDs.1,2 Differential or selective inhibition of COX-2 clearly offers potential some safety benefits by avoiding loss of homeostatic PGs; however, loss of COX-2 activity is also associated with adversities.14 It is important to note that COX-2 is ofttimes constitutively expressed: in the kidney and brain it mediates a cytoprotective effect in damaged or inflamed gastrointestinal mucosa.
Cyclooxygenases in health and disease
An appreciation of both efficacy and safety of COX inhibition (e.g., NSAIDs) is facilitated by an understanding of the role of COX in healthy and diseased tissues. COX has been described as the most common target of drug therapy with NSAIDs. In general, inhibition of COX-2 is responsible for efficacy, whereas inhibition of COX-1 is responsible for side effects.9 However, strict adherence to this simplistic approach will lead to therapeutic failure and increased morbidity with NSAID use. Although COX-1 does indeed appear to be the predominant constitutive enzyme responsible for housekeeping, both COX-1 and COX-2 are constitutively expressed in many tissues. Further, although COX-2 clearly is more active in the promotion of inflammation, COX-1 does appear to have some role.
Inflammation
PGs, and primarily PGE2, induce vasodilation, capillary permeability, and chemotaxis. As such, PGs cause the cardinal and clinical signs of inflammation, including pain and fever.8 PGE also modifies both T-cell and B-cell function, in part by inhibition of IL-2 secretion.8 Other inflammatory effects of PGE include its regulation IL-6, macrophage colony-stimulating factor, and vascular endothelial growth factor. In general, research consistently indicates that it is COX-2 that predominantly mediates formation of PGE associated with inflammation, pain and fever. For example, whereas COX-1 is largely absent in normal synovial cells, COX-2 is induced in most types of arthritis, including inflammatory arthritis in animals and rheumatoid arthritis in humans. In cartilage COX-2 is associated with IL-1 degradation of proteoglycan and apoptosis of synovial cells.18 However, not all of the inflammatory actions of COX-2 are undesirable in that COX-2 induction in response to inflammation contributes to tissue healing. For example, COX-2 is associated with healing in ligaments, bone, the gastrointestinal tract, and other tissues. The importance of COX-2 to dermal healing is not yet known.9
Central Nervous System
Both COX-1 and COX-2 are consitutively expressed in the brain and spinal cord. Constitutive COX is very responsive to ischemia, immunomodulation, cytokines, toxins, brain damage, and maturation processes.17 However, although both isoforms are present, COX-2 predominates. PGs of the central nervous system (CNS) play a major role in pain; howevever increasingly they are recognized for roles in other disorders. Among them is the role of COX in the pathogenesis of Alzheimer’s disease (AD). Extracellular deposition of fibrillar amyloid β (Aβ), intracellular accmumulation of abnormally phosphorylated tau protein, and subsequent formation of Aβ plaques mediating neurodegeneration and dementia in AD are associated with inflammation and COX-2.19 Mediators of inflammation are present throughout all stages of the disease, whereas COX-2 is absent in normal astrocytes or microglial cells. Further, COX-2 is upregulated in acute brain injury and in animal models of AD.9,19 Finally, the connection between AD and COX is supported by a lower incidence of AD in patients with rheumatoid arthritis, presumably because of the use of NSAIDs for its treatment.19 The protective effects of NSAIDs in the AD patient may reflect the antiplatelet properties of NSAIDs; aspirin in particular may decrease the risk of ischemic damage induced by blocked capillaries of the brain. Decreased formation of amyloid β protein also has been proposed,9 suggesting a possible role in other diseases associated with amyloid β protein deposition. Finally, COX-2 appears to be involved in the loss of glutamate-induced apoptotic cell death.19 Because N-methyl-d-aspartate (NMDA) receptors stimulate the arachidonic acid cascade, NSAIDs mute the role of NMDA receptors in pain. NSAIDs may also inhibit NMDA–mediated neuronal cell death by preventing increased extraceullar glutamate.19a
Pain
PGs have been implicated in causing increased pain perception (allodynia) in damaged compared with normal tissues.1,2 Induced COX-2 PGE as been associated with hyperalgesia (exaggerated response to pain) at the level of the spinal cord (primary hyperalgesia) or nociceptors (secondary hyperalgesia).17 Induction of COX-2 in the dorsal horn has been associated with central sensitization, manifested as a change in excitability threshold.9,17,18 Currently being descriped is the ability of PGs to contribute indirectly to neuropathic pain through influence on chemical mediators (e.g., histamine, bradykinin, substance P, nitric oxide [NO]), neurotransmitters (e.g., glycine inhibition or glutamate stimulation), or modulation of other receptors (e.g., NMDA).
Gastrointestinal and Other Healing
Both COX-1 and COX-2 are constitutively expressed in the gastrointestinal tract. However, constitutive expression of COX-1 has a predominant role in the protection of the gastrointestinal tract. COX-1 PGs decrease hydrochloric acid secretion, increase mucosal bicarbonate and mucus production, and increase epithelial cell proliferation and mucosal blood flow (Figure 29-5). The latter effect facilitates delivery of oxygen and nutrients to proliferating cells as well as rapid removal of damaging hydrogen ions that make their way into the mucosa. Drugs that express preferential potency for COX-2 PGs generally are associated with fewer gastrointestinal side effects compared to those targeting both COX isoforms, although the relative protection varies with the drugs. Protection appears to be more important in the presence of high drug concentrations (e.g., high doses).20 COX-2 is critically important to the gastrointestinal tract as well, being important for healing of gastrointestinal damage: COX-2 appears within 1 hour of gastrointestinal damage. Newer coxib PGs will inhibit gastrointestinal healing as has been demonstrated in dogs receiving firocoxib (see the discussion of tepoxalin).21 Interestingly, in the pancreas, constitutive COX-2 expression dominates, although the clinical relevance of this is not yet known.20 The impact of NSAIDS on bone healing is an emerging concern. COX influences osteoblast and osteoclast; experimental studies have demonstrated impaired bone healing.20a Their use in controlling pain and limiting ectopic bone growth must be balanced with the increased risk of impaired fracture healing; conventional NSAIDs might be preferred compared to newer NSAIDs. The impact of NSAID on soft tissue healing (or ligaments) remains to be confirmed; whereas short term use may have minimal negative (and potentially a positive) impact, long term used might be approached cautiously. A recent meta-analysis in humans found no advantage in terms of return to function for coxibs, but recommended caution with long term use.20b A strong association has been reported between NSAIDs and severe necrotizing soft tissue infections.20c
Cardiovascular Disease
The role of PGs in the cardiovascular system is largely beneficial, and the relationship between COX-1 and COX-2 and their respective PG end products exemplifies the complex “ying–yang” balance that characterizes them (Figure 29-6). Platelets contain thromboxane, a synthase, which catalyzes the formation of thromboxane from AA. Thrombosis reflects platelet aggregation and vasoconstriction. The formation of a thrombus is kept in check by the presence of prostacyclin synthase in vascular endothelial cells. This enzyme catalyzes metabolism of AA to prostacyclin (PGI2), a vasodilatory and platelet-inhibiting PG end product. However, whereas thromboxane A2 (TXA2) is associated with COX-2, prostacyclin synthase co-localizes with COX-1. Thus, whereas drugs that target both COX isoforms will potentially allow the balance to be maintained, drugs that preferentially target only one isoform risk disruption of the balance. Such may be the case with COX-2 selective NSAIDs. Their preferential inhibition of COX-2 may allow thrombus formation to go unchecked, increasing the risk of thromboembolic disorders. Indeed, in a meta-analysis of human clinical trials, two coxib drugs (rofecoxib and valdecoxib) were associated with an increased risk of stroke (see “Adverse Events”).20d
Kidney
In the kidney both COX-1 and -2 are constitutively expressed. Both are formed in the macula densa of humans and animals, but COX-2 may have a more important role than COX-1 (Figure 29-7). In animals, inhibition of COX-2 causes sodium and potassium retention in salt-depleted, but not normal, animals. However, in humans COX-2 appears to influence renal vasculature and podocytes. The role of COX in the kidney requires further elucidation before safety can be assumed for any NSAID; sparing COX-1 and targeting COX-2 can be expected to alter renal function. For example, kidneys do not develop in the embryos of COX-2–null knockout mice.22 The role of COX differs among tissues and species; their impact on adverse reactions are addressed under “Adverse Reactions.”9
Reproductive tract
Both COX-1 and -2 play a significant role in the normal reproductive tract. Induction of COX-2 is associated with ovulation, fertilization, implantation, and decidualization, as well as induction of labor.22 Female knockout mice devoid of COX-2 are largely infertile.22
Cancer
In the 1990s a reduced risk of colon cancer was associated with consistent aspirin use. Subsequent studies demonstrated a marked increase in COX-2 in a variety of soft-tissue tumors in humans and in transitional cell carcinoma in dogs.23 These studies suggest that benefits of NSAIDs in cancer may reflect inhibited COX-2.9 Mechanisms by which COX-2 may facilitate cancer growth or spread include impaired apoptosis, transactivation of epidermal growth factors or receptors and promotion of angiogenesis.9,17 Depending on the model, inhibition of COX-2 by NSAIDS reduces cell proliferation, increases apoptosis, and reduces metastasis. COX-2 inhibition may also enhance antitumor effects of radiation, although host toxicity also will be increased. Not surprisingly, gastrointestinal toxicity of anticancer drugs is also increased, presumably reflecting a combined toxic effect on the gastrointestinal tract.9 COX-1 may also have a role in cancer as is suggested by a decrease in colon cancer in COX-1 knockout mice.24
Cycloloxygenase and nonsteroidal antiinflammatory drugs
NSAIDs (Table 29-2) act to block PG synthesis by binding to and inhibiting cyclooxygenase (see Figures 29-1 and 29-2).8 This action is both dose and drug dependent. The planar form that characterizes these drugs is thought to facilitate their binding to COX.6,25 Several investigators have shown that some drugs (e.g., phenylbutazone and flunixin meglumine) also reduce later steps of the formation of PGE2 in inflammatory exudate at therapeutic doses.26 However, both the major therapeutic and toxic effects of NSAIDs have been correlated extensively with their ability to inhibit PG synthesis, with anti-inflammatory potency related to potency of impaired PG synthesis.8
The differential effect of NSAIDs on the isoforms of COX presumably contribute to clinical differences in efficacy and safety, .17,27 Whereas as a class, NSAIDs appear to inhibit both COX-1 and COX-2, the ratio of the concentration of a NSAID necessary to inhibit the same level of COX-1 vesrus COX-2 activity (e.g., inhibition of 50% or 80% activivity [IC50 or IC80]) compares the potency each drug toward each isoform, and as such, provides a basis for screening predicted relative safety and efficacy of each. Thus, a COX-1 to COX-2 ratio greater than 1 (or a COX-2 to COX-1 ratio of less than 1) indicates greater potency for COX-2 which is desirable in that formation of inflammatory PGS should be inhibited preferentially to the housekeeping PGs.12,13,15,16 Inhibition of platelet activity is commonly used to measure in vitro COX-1 inhibition whereas inhibition of PGs released from macrophages stimulated with endotoxin is used to measure COX-2. A number of drugs associated with in vitro COX-2 selectivity in humans, including rofecoxib (Vioxx) and celecoxib (Celebrex); others include etodolac, meloxicam, and nimesulide; the newest drugs are valdecoxib, etoricoxib, and (soon to be approved) lumiracoxib.9 Drugs approved for use in animals include carprofen (the first) followed by etogesic; deracoxib; meloxicam; and, most recently, firocoxib.
Although the gene for COX-1 is about 3 times as large as that for COX-2, the two COX proteins, which are membrane bound, share about 60% homology.9 Differences in NSAID selectivity for these two enzymes reflects smaller amino acids at two positions in COX-2 compared with larger amino acids at the same site in COX-1. The result is a larger and more flexible “pocket” into which the newer drugs insert and inhibit the COX-2 isoform.9 For example, ”coxib” drugs contain a sulfonamide group that interacts through hydrogen bonding to arginine of COX-2 but not histidine in the same position in COX-1.28 Despite the usefulness of the COX-1:COX-2 ratio in screening drugs for safety or efficacy, its applicability to clinical use is questionable. Further, comparing results among studies is difficult. For example, whereas some studies focus on the IC50, others determine the IC80, the latter probably being more clinically relevant. Assay methods contribute to variability in ratios among investigators for the same drug in the same species (Table 29-3). In vitro assasys based on cell culture or recombinant enzymes are easier to perform, but it is the ex vivo whole blood assays (as opposed to in vitro cell culture assays) that generally are recognized as the most representative of the clinical patient.1 However, even whole blood assays do not take into account different distribution patterns to different tissues.18,22 Extrapolation among species should be avoided.29 For example, wherease the COX-1 to COX-2 ratio of etodolac is much better than that for carprofen in humans,20,30 the opposite is true in dogs. Indeed, Wilson and coworkers31 have demonstrated that, wherease critical residues in the active sites of canine COXs are identical with human homologs, amino acids differ (up to 54) at in active sites and may contribute to differences in activity that preclude extrapolation among species (see Table 29-3). Further complicating extrapolation among and within species is the fact that NSAIDs generally exist and are largely marketed as enantiomers (see Chapter 1), each with a possible different ratios. For example, Ricketts and coworkers32 found the COX-1:COX-2 ratio of carprofen to be 129 for the racemic mixture but 181 for the S isomer and only 4.19 for the R isomer. Little information is available regarding COX selectivity in cats.33,34 Much of the information that is available is based on manufacturer-sponsored studies and as such might potentially reflect reporting bias. For example, in Table 29-3, drugs that generally performed best in each study tended to be the drug manufactured by the sponsor of the study. As such, COX ratios might be considered a screening procedure1,2
Table 29-3 COX-1 to COX-2 Ratios for Selected Nonsteroidal Antiinflammatory Drugs in the Dog by Different Investigators
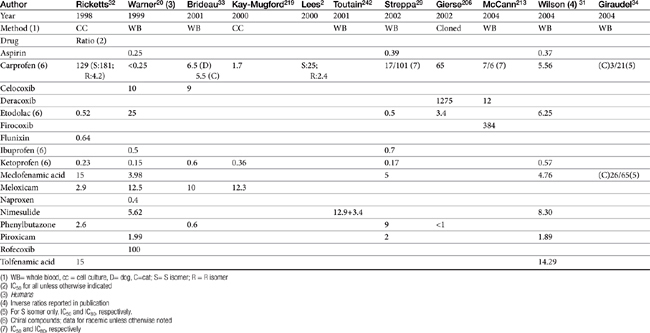
Other Mechanisms of Action
Inhibition of eicosanoid formation is not the sole antiinflammatory mechanism of action of NSAIDs. The NSAIDs also appear to alter cellular and humoral immune responses and suppress inflammatory mediators other than PGs.7 As a group, all NSAIDs are planar and anionic, thus able to partition into lipid environments, including neutrophil cell membranes. As a result, cell membrane viscosity is altered, even at low concentrations.10 At higher concentrations, NSAIDs appear to uncouple protein–protein interactions within the plasma membrane and thus interfere with a variety of cell membrane processes. Examples include oxidative phosphorylation and cellular adhesion.10 Response of inflammatory cells to extracellular signals is impaired by affecting signal transduction proteins (G proteins).10 Neutrophil adherence and activation is inhibited, as is subsequent release of inflammatory cellular enzymes, including collagenase, elastase, hyaluronidase, and others.7 The extent of these effects varies with the drug. Whereas piroxicam inhibits superoxide ions and lysosomal enzymes release, ibuprofen does neither.10 All NSAIDs appear to inhibit neutrophil adhesion. Some NSAIDS directly block PG receptors. Inhibition of nuclear transcription factor NF- B, is responsible for expression of many genes linked to inflammation. Modulation of peroxisome proliferator-activated receptors (PPARs), heat shock proteins (HSPs) and mitogen-activated protein kinases (MAPK cascades), and impaired induction of nitric oxide synthase (iNOS) are among the COX-independent effects of the class of NSAIDs.2,28,35 Selected drugs (e.g., celecoxib but not rofecoxib) directly inhibit signaling needed to promote angiogensis, invasiveness, and proliferation of neoplasias.28 Interestingly, sulfone coxibs (see Figure 29-4) are pro-oxidant, whereas sulfonamide coxibs are not.28 Connective tissue metabolism may also be affected.7
NSAIDs are immunomodulators by virtue of their effect on several PGs and LTs as immunomodulators.8 Nonsteroidal antiinflammatory cells indirectly influence lymphocyte activity through altered PG formation.7 Selected NSAIDs appear to enhance cellular immunity by inhibiting PGE2, a mediator that dampens the immune response.7 This effect appears to be more important in the immunosuppressed animal.
Pharmacokinetics
Shared Pharmacokinetic Properties
Each drug will be discussed in depth; however, the NSAIDs share a number of pharmacokinetic properties as a class. Package insert data for dog and cat contains selected information for some drugs, although the information often is not comprehensive, with content tending to vary, in part based on the requirements implemented by the FDA at the time of drug approval. The United States Pharmacopeia has provided a series of monographs providing pharmacokinetic information regarding the use of NSAIDS in species; information will be addressed for individual drugs. As weak acids, the NSAIDs tend to be well absorbed after oral administration. Bioavailability can vary between animals but has not been established for many drugs because of the lack of intravenous preparations.36 Solutions of selected injectable preparations tend to be alkaline and can cause necrosis or pain if perivascular leakage occurs Food can impair the oral absorption of some NSAIDs or contribute to drug interactions.37,38 The drugs are lipid soluble. Whereas the volume of distribution tends to be small (approximating 10%) when based on total drug as a result of ≥ 90% binding to serum albumin39 the volume of distribution of unbound drug is consistent with distribution to extracellular fluid (Table 29-4). Only a small portion of pharmacologically active drug reaches peripheral tissues. Displacement from albumin (e.g., due to competition with other substrates for binding sites or from decreased serum albumin concentrations) may result in higher than expected concentrations of pharmacologically active drug and thus predispose the patient to drug-induced adverse effects. Although this increase is likely to be transient as the elimination of of unbound drug increases,36,40 adversities might yet emerge particularly in patients at risk (e.g, decreased hepatic or renal function). Clearance of unbound drug is likely to be reduced in geriatric patients. The volume of distribution of unbound drug in pediatric animals is twice that in adults, which will contribute potentially to longer half-life.36 For this and other reasons, (see metabolism), NSAIDs should not be used in the pediatric patient.
Most NSAIDs are eliminated primarily by both phase I and II hepatic drug-metabolizing enzymes. Clearance differs in both rate and extent among drugs and species. Differences in clearance are largely responsible for differences in drug half-life among animals.36 Failing to anticipate these differences may contribute to adverse reactions. Conjugated parent drug or metabolites are eliminated through the bile or urine, depending on the drug. Stereoselective metabolism plays a profound role in species, gender and age differences. Saturation of drug metabolizing enzymes may occur at doses higher than recommended clinically for some drugs as has been demonstrated for phenylbutazone and derocoxib (package insert). Genetic polymorphism has been described in dogs for at least one NSAID (celecoxib)41 and is likely to occur for others. Several drugs, or their enantiomers, undergo extensive enterohepatic circulation, again with differences among species (e.g., naproxen, meclofenamic acid, and the S enantiomer of carprofen in dogs), increasing the risk of gastrointestinal toxicity.42 Renal active tubular secretion occurs for some parent drugs. Naproxen, carprofen, ketoprofen, and celecoxib are among the drugs which exemplify pharmacodynamics and pharmacokinetics differences in safety and efficacy among species.36 Whereas allometric scaling generally can accurately predict systemic clearance and volume of distribution (based on body weight) of NSAIDs, allometric scaling generally cannot predict differences in pharmacodynamic indices (e.g., inhibition of TXB2 and PGE2 synthesis).43
Integration of Pharmacokinetics and Pharmacodynamics
Pharmacologic Effects
The pharmacologic effects of this class of drugs include analgesia, antipyresis, and control of inflammation. Antithrombosis also occurs, but this effect is variable, being the greatest for aspirin and the least to absent for newer COX-2 selective drugs. The effects are dose and drug dependent and include antithrombosis (when present), which occurs at the lowest concentrations (relatively lower for aspirin compared to all others); followed by antipyresis, analgesia and antiinflammation, with the latter occurring at the highest concentrations, although an exception appears to occur again for aspirin.10,43d Studies that compared efficacy and safety traditional or newer NSAIDs are generally are based on in vitro or ex vivo methods, with clinical trials limited. Yet, differences should be anticipated even among the newer NSAIDs. The mechanisms by which NSAIDs inhibit (interact with) COX are responsible, in part, for the variable antiinflammatory effects that characterize these drugs. Although several NSAIDs are characterized by a short plasma elimination half-life, the clinical response may last for over 24 hours after a single dose or up to 72 hours after multiple doses. These differential durations reflect, in part, drug-receptor interactions,with irreversible binding to COX most likely contribute to a longer biologic response.44 Lees and co-workers have reviewed the relationship of NSAID effect and dose, emphasizing the advantages of PK-PD modeling as a basis of dose design.44a Inhibition of COX-1 has been described as simple competitive inhibition, whereas that for COX-2 has been described as a slowly reversible, contributing to a longer biologic versus plasma half-life.45 Aspirin binds reversibly to the COX activity site on PGH synthase and then irreversibly inactivates the enzyme by acetylating a serine residue.10 Both thromboxane and prostacycline synthase are impacted. However, platelets cannot produce additional thromboxane synthase; as such, platelet activity depends on new platelet production in the absence of aspirin. In contrast, endothelial cells are able to synthesize more prostacyclin synthetase and are less susceptible to the inhibitory effects of low dose aspirin.10 In contrast to irreversible binders of COX, ibuprofen binds reversibly with COX and thus competes with AA for binding. In laboratory animals and humans, a relative potency (not to be confused with efficacy) has been established for selected conventional NSAIDs and COX: meclofenamic acid >indomethacin >naproxen >phenylbutazone >aspirin.44 However, differential potency for COX-1 versus COX-2 varies among the NSAIDs and their enantiomers. In addition to drug-receptor interaction, prolonged elimination of NSAIDs from inflammatory exudate compared with plasma may also result in differential effects among the drugs and species.37,44
Along with inhibition of PGs, disruption of cellular signaling is responsible for all three pharmacologic effects (antiypresis, analgesia, anti-inflammation) that characterize all NSAIDs.10 The antipyretic effect of NSAIDs may or may not be of benefit. Indeed, in human medicine little scientific support exists for the use of antipyretics for relief of discomfort or reduction of morbidity and mortality associated with fever.47 Fever may have a beneficial effect on the outcome of bacterial or viral infections and often inversely correlates with the severity of lesions or the time to resolution. Thus the antipyretic effects of NSAID may be of most benefit when increased metabolism induced by fever might prove detrimental, such as with septicemia.47 Central analgesic effects have been suggested for some but not all NSAIDs because NSAIDs can provide analgesia at very low intrathecal doses.15,16,46
The impact of COX on disease has led to a number of new applications for NSAIDs. The antiendotoxic effect of selected NSAIDs has been known (see the discussion of flunixin), but newer mechanisms are being examined. For example, several NSAIDs (carprofen, flunixin meglumine, and phenylbutazone) may specifically inhibit activation of the proinflammatory transcription factor nuclear factor kappa B (NF-κB) and on lipopolysaccharide (LPS) induction of iNOS. Using a mouse macrophages line, carprofen and flunixin, but not phenylbutazone, inhibited iNOS, whereas carprofen and, to a lesser degree, flunixin, inhibited NF-kappaB activation.35
Because NSAIDs are commonly first-choice treatments for arthritis, a focus on the effects of NSAIDs on cartilage is warranted. NSAIDs have been shown to inhibit proteoglycan synthesis in vitro, and for the salicylates this is supported by in vivo studies.48 This effect has been attributed to inhibition of uridine diphosphate glucose dehydrogenase, an enzyme important in proteoglycan synthesis.48 Hyaluronic acid synthesis, also dependent on this enzyme, does not appear to be as affected. The clinical effects of NSAIDs on cartilage are controversial. Some NSAIDs may, in fact, favorably modify the metabolism of proteoglycans, collagen, and matrix and may decrease the release of proteases or toxic oxygen metabolites.48 This may reflect, in part, control of inflammation. Thus, whereas several NSAIDs have documented adverse effects on normal cartilage, ranging from decreased proteoglycan synthesis (e.g., aspirin) to chondrocyte death (phenylbutazone), others (e.g., naproxen, piroxicam, ketoprofen, and possibly carprofen) are recognized for their chondroprotective effects. Dual inhibitors have been noted for their potential efficacy to slow the progression of arthritis.48b
The use of NSAIDs in the control of acute pain is evolving. Preemptive use (just before and immediately after surgery) has been demonstrated in humans to be more effective than either placebo or control,17 and similar findings have been reported in the clinical use of COX-1–sparing drugs in animals. Drugs that target COX-2 (NSAIDs) offer a morphine-sparing effect for control of postoperative pain.17 Their use in multimodal analgesia is increasing.
NSAIDs are currently being investigated for their potential antitumor effects. Knapp and coworkers50 found piroxicam to be clinically useful in reducing tumor size and increasing survival time in dogs with transitional cell tumors of the urinary bladder. The result does not appear to reflect direct cytotoxic effects.49–51 NSAIDs may be beneficial when combined with other anticancer drugs.52 The effects of drugs inhibiting COX-2 cancerogenesis may be dose dependent: At least for aspirin, the maximum anticancer effect occurs at a dose less than that associated with control of inflammation but similar to that associated with antiplatelet effects.9 Mechanisms other than COX inhibition contribute to NSAID antitumor effects.51a In addition to the inhibition of cancer growth, the use of COX-1–sparing drugs may improve gastrointestinal tolerance of anticancer drugs.
Drug Interactions
The NSAIDs can be involved in a variety of drug interactions during any phase of drug disposition (see the discussion of individual drugs). Trepanier53 has reviewed some of these interactions. Displacement of only a small percentage of bound drug from albumin can increased the concentration of pharmacologically active drug in tissues. Few, if any, adverse reactions resulting from drug displacement have been reported, in part because of the failure to recognize the combination as problematic. In addition, the increase in pharmacologically active drug is only transient: Clearance of the unbound drug by both the liver and kidneys will increase.36,40 However, the impact of changes in protein binding in patients with altered liver function may be problematic; accordingly, attention to the possibility that protein-binding drug interactions may worsen risks associated with liver disease might be prudent. Several NSAIDs can induce or inhibit drug-metabolizing enzymes and thus the clearance and half-life of other drugs cleared by the liver.36 Phenylbutazone can both increase and inhibit selected drug-metabolizing enzymes depending on the second drug, whereas salicylates increase metabolism.36 Renal competition with other organic acids for active renal tubular secretion in the proximal tubule has been documented for aspirin and other drugs, although the clinical relevance of this is not clear.
Drug interactions also occur at the level of pharmacodynamics and may increase the risk of adversities. Most notable is the combinations of NSAIDs or glucocorticoids, which increase the risk of gastrointestinal toxicity (discussed later). Also notable are those drugs that, like NSAIDs, alter renal blood flow, and potentially renal autoregulation, thus increasing the risk of nephrotoxicity (e.g., aminoglycosides, angiotensin-converting enzyme inhibitors, amphotericin B). NSAIDs, in turn, may blunt response to diuretics and hypertensive drugs. NSAIDs may also block endogenous responses to hypertensive drugs (e.g., alpha adrenergics such as phenylpropanolamine), thus increasing the risk of hypertension, although this is more likely a risk only in animals for which primary hypertension is a concern.54 The impact of combining NSAIDs with other drugs that affect platelet function or coagulation proteins is complicated by the differences in mechanisms of action between nonselective and preferentially selective drugs. Nonselective drugs would be expected to potentiate platelet function defects or deficiencies, whereas preferentially selective drugs would not. Prudence dictates that NSAID use ideally be avoided in patients with metabolic, hematologic, cardiovascular, or other disorders that put the patient at risk. NSAIDs are more likely to cause CNS effects if combined with fluorinated quinolones with unsubstituted piperazinyl rings (ciprofloxacin) at position 7.55 It is not clear if the interaction occurs at the level of CNS (at the level of the gamma-aminobutyric acid receptor) or as a result of altered clearance.56
Selective serotonin reuptake inhibitors increase the risk of upper gastrointestinal hemorrhage based on a meta-analysis of the human-medicine literature.57 The risk is greater in elderly patients; those drugs most selective for reuptake are associated with a higher risk. Among the proposed mechanisms is depletion of platelet serotonin and loss of platelet aggregation.
Adverse Reactions
All NSAIDs induce undesirable and potentially life-threatening side effects. In general, side effects tend to be predicted by toxicity studies implemented during the approval process,58 although the small number of animals tends to limit their prediction to only the most common side effects. The role of drug interactions in causing adverse events was previously described. Accidental poisoning has been described for several NSAIDs, with the most common among the conventional drugs being ibuprofen, acetaminophen, aspirin, and indomethacin;59 decreased use of these drugs as newer NSAIDs are used decreases relevancy. The most common clinical signs of toxicosis were vomiting and diarrhea followed by CNS depression, and circulatory manifestations.59 Most adverse reactions reflect the inhibitory effects of NSAIDs on PG activity. Acute intoxication by selected drugs can be fatal; the more common adverse drug events are discussed along with their prevention and treatment below.
The adverse event reporting site of the Food and Drug Administration (FDA) is publically acceptable and can be reviewed for adverse drug events associated with NSAIDs in animals.59a However, effective epidemiologic assessment is limited and cause and effect between drug and adverse drug event may not exist. Further, the FDA is not provided information regarding the number of units sold precludes standardizing incidence of adverse drug events among drugs. Among the greatest contributing limitations to the adverse event reporting site may be failure of veterinarians or clients to report adverse events.
Transitioning from one NSAID to another in an attempt to reduced toxicity (or improve efficacy) should be based on elimination half-life (plasma or biological, whichever is longer). For those that reversibly impair COX, 3 to 5 elimination half-lives of the current NSAID should elapse prior to initiating the second (see Table 29-4). Prudence dictates that prophylactic measures be implemented during transition for at-risk patients.
Toxicity as a result of overdosing should be treated as with other overdoses (i.e., removal of ingested drug, supportive therapy). Treatment generally should continue for at least 3 to 5 half-lives of the ingested NSAID; longer may be necessary, particularly if the intoxicating amount is sufficient to saturate drug metabolizing enzymes. The risk of saturation kinetics is greater in age extremes, for selected drugs (e.g., phenylbutazone, deracoxib), and in the presences of liver disease. For NSAIDs characterized by enterohepatic circulation, administration of cholestyramine should be considered. The drug binds to at least several NSAIDs, thus preventing enterohepatic circulation, decreasing half-life as well as gastrointestinal exposure.60 Treatment of other specific disorders is addressed with the adverse drug events.
Gastrointestinal
Mechanism of Gastrointestinal Adverse Events
Gastrointestinal damage is the most common and serious side effect of the NSAIDs. Dogs are described as being “exquisitively sensitive” (see package insert for meclofenamic acid, Fort Dodge) to NSAID-induced gastrointestinal ulceration.The incidence of gastrointestinal side effects associated with NSAID use in dogs (or cats) is not known. However, essentially every NSAIDs (conventional and new) used in the dog has been cited literature, at the FDA adverse event reporting site, in manufacturing package inserts, or other sources as causing gastrointestinal adverse drug events.61–66 Gastrointestinal ulceration should be anticipated in dogs receiving these drugs, and clients should be counseled regarding the side effects and potential treatments for ulcerative injury. The incidence of side effects associated with new NSAIDs approved for use in animals has led the inclusion of client information sheets (as part of package inserts) to be distributed to clients when NSAIDs are dispense to animals for at home therapy.
The mechanism of gastrointestinal damage is not completely understood. A review of the FDA-CVM adverse event website reveals gastrointestinal adversities to be the most common for most, if not all, NSAIDs for which adversities are reported. In the cat, gastrointestinal adversities are the first, or more commonly second, adverse event reported for most NSAIDs. It is likely that several mechanisms act in concert to cause adversities; the mechanisms and risks as they are perceived in human medicine have been reviewed.67–69 Several mechanisms offer a target for prevention and treatment. Gastroduodenal erosion and ulceration reflect, in part, inhibition of COX-1–stimulated PGE2-mediated bicarbonate and mucous secretion, epithelialization, and increased blood flow.70–71 Breakdown of small blood vessels resulting from a deficiency of mucus may be the initiating lesion.72 Enhanced LT synthesis from AA shunted from the COX pathway to the lipoxygenase pathway exacerbates damage: due to vasoconstriction-induced mucosal damage as well as platelet aggregation and neutrophil activation. Dual inhibitors which target LTs as well as PGs may be less ulcerogenic. Direct irritation contributes to gastrointestinal mucosal damage for acidic drugs: 70 ion trapping in the mucosa precipitates hydrogen ion diffusion from the lumen of the stomach to the mucosa.70,73 The lack of a sensitive indicator of gastrointestinal damage complicates assessment of gastrointestinal adverse drug events caused by NSAIDs. Boston and coworkers74 as others could not find a relationship appears between the presence of gastric lesions and positive fecal occult blood. A method based on sucrose absorption in the gastrointestinal tract may be successful for detecting NSAID-induced gastrointestinal damage.75 Some investigators have gone so far to suggest that endoscopic presence of erosive lesions is not necessarily indicative of ulcerogenic effects of NSAIDs.76 The lack of well-designed clinical trials assessing detection, risk, prevention or treatment is problematic. Some studies continue to inappropriately ascribe the lack of statistical differences among treatment groups as evidence of no treatment (diagnostic) effect. Yet, failure to detect a significant difference (type II statistical error) often reflects the small sample size characterizing these clinical trial and caution is recommended to not overinterpret “lack of significant difference” as “sample populations are similar”.
Prevention and Treatment of Gastrointestinal Adverse Events
Prevention of gastrointestinal toxicity is based on identifying those patients at greatest risk; avoiding NSAIDs drugs, if possible, in these patients; and if this is not possible, selecting the safest drug (i.e., COX-1–sparing drugs) and using it in a dosing regimen that results in the lowest exposure necessary for efficacy. Prevention also includes co-treatment with prophylactic drugs, including those that prevent gastric acid secretion or those that replace PGs.73 The use of other therapies that might decrease the need for NSAIDs (e.g., combination analgesic therapy, cartilage-supportive disease-modifying agents) also is indicated.
Risk factors for NSAID-induced gastrointestinal adverse events
Several risk factors for NSAID-induced gastrointestinal adverse events identified in humans are applicable to animals. No chemical characteristic predicts the likelihood of gastrointestinal toxicity by a particular conventional NSAID.70,72 Drugs that undergo enterohepatic circulation (e.g., naproxen, carprofen, etodolac) may be associated with a greater incidence of gastrointestinal upset. Other risk factors include advanced age (altered disposition coupled with decreased ability to protect damaged mucosa),77 concurrent use of glucocorticoids (increasing the risk 4.4-fold in humans) or other NSAIDs (the exception might be low-dose aspirin, as discussed later), or anticoagulants. Comorbidity (renal, cardiovascular, or liver disase) is also a risk factor.73
The combination of steroids and NSAIDs causes worse lesions than either drug alone, as has been demonstrated for flunixin meglumine or dexamethasone.74,78,79 Further, the FDA adverse drug events site indicates that the risk of gastrointestinal toxicity is greater when drugs are given with glucocorticoids. In a restrospective study of gastrointestinal perforation associated with deracoxib, a risk factor was combination with glucocorticoids (or other NSAIDs) within the past 24 hours.80 Other NSAIDs appear to shift use of AA from the COX pathway to the production of cysteinyl LTs and LTB4, eicosonoids that promote leukocyte migration, break down the mucosal barrier, and stimulate gastric acid secretion. Inhibition of COX-2 may preclude angiogenesis critical to the healing ulcer.81 Persons with previous history of gastric ulcer disease also are predisposed to NSAID-induced gastrointestinal adverse drug events. Although peptic ulcer disease is unusual in animals, prudence dictates that evidence of any gastrointestinal disease associated with mucosal damage (e.g., inflammatory bowel disease) that might require COX-2–mediated healing be considered a potential risk factor.
Dose-dependent toxicity has been demonstrated during the approval process essentially for all NSAIDs as is indicated by product package inserts (Table 29-5). The single most common cause of NSAID-induced toxicity found by a review of NSAID-induced adverse drug event reports by the FDA is overdosing, in particular failure to adhere to the recommended dosing regimen. Overdosing was associated with an increased risk of NSAID-induced gastrointestinal perforation in dogs.80 Drugs for which low-concentration preparations are not available increases the risk of toxicity because of inaccurate dosing. Use of compounded preparations (for which oral bioavailability is not known) should be implemented with extreme cautions; increasing bioavailability will result in a proportional increase in drug concentrations. The risk for overdosage is greater for selected drugs for which higher doses may result in zero-order elimination (e.g., deracoxib, phenylbutazone). For such drugs half-life is no longer germane, and the risk of drug accumulation dramatically increases.
Table 29-5 Safety Data for Selected NSAIDs in Dogs and Cat Based on Target Safety Studies and Clinical Trial Field Studies
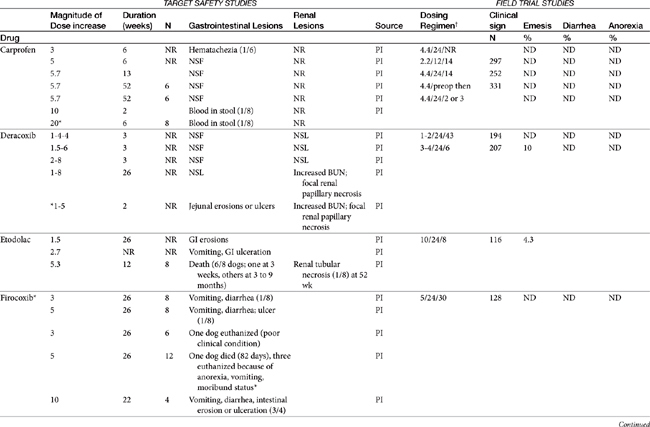
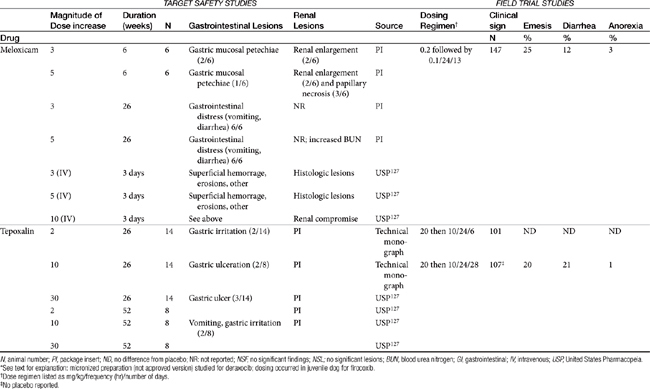
The relationship between dose and risk of toxicity remains complex, even for NSAIDs, as is exemplified by aspirin. At low doses (concentrations), aspirin blocks COX activity and thus shunts AA into the lipoxygenase pathway. Although gastrotoxic LTs are produced (cysteinyl LTs and LTB4), aspirin also simultaneously triggers lipoxin (aspirin-triggered lipoxin [ATL]) formed by lipoxygenase-15. Lipoxin is antiinflammatory and thus inhibits the gastrotoxic leukocyte recruitment and activation caused by cysteinyl LTs, as well as mediates the antiadhesive platelet effects of aspirin. As such, low-dose aspirin tends to be both cardioprotective as well as safe to the gastrointestinal tract. However, at higher doses production of LTs is sufficient that the protective effects of lipoxin are masked and gastrotoxicity emerges. Interestingly, COX-1–sparing (COX-2 preferential drugs) inhibit the formation of lipoxin, contributing to the toxicity of the newer drugs when combined with low-dose aspirin.81 Theoretically, whereas COX-inhibiting drugs should not be combined with low-dose aspirin, dual-acting NSAIDs, which do not target lipoxygenase-15 (see the discussion of dual-acting NSAIDs), should not inhibit lipoxin and thus should maintain the low-dose aspirin gastroprotective and cardioprotective effects.81
In general, conventional NSAIDs are associated with a greater risk of gastrointestinal adverse drug events in humans, but exceptions may occur. For example, in humans (but not dogs) ibuprofen, which is essentially equivalent in its COX-1 versus -2 selectivity, is ranked as low risk, along with the COX-1–sparing drugs celecoxib, rofecoxib, meloxicam, and etodolac.73 Comparison in dogs of gastrointestinal safety among newer NSAIDs, including both COX-1–sparing drugs and dual-acting NSAIDs, might be based on toxicity data generated during the approval process and available on package inserts. This latter source includes both targeted safety studies (the dose is multiplied by 3, 5, or 10) and field studies (clinical trials at recommended doses under conditions of anticipated use) (see Table 29-5).
Comparison of clinical safety among NSAIDs ideally should reflect nonmanufacturer-sponsored, well-designed clinical trials involving a sufficient number of animals such that the power is sufficient to detect a significant difference. Placebo controls are imperative; positive responses have been reported in 40% or more of placebo-treated animals.82 However, such studies in veterinary medicine are few and far between. Data that do exist thus far support the safety of the newer drugs compared with conventional NSAIDs. For example, using a placebo-controlled, parallel, randomly assigned design in dogs (n = 6/group), Reimer and coworkers83 found that buffered aspirin caused endoscopic gastric lesions within 5 days of starting therapy compared with no or minimal lesions in animals treated with carprofen or etodolac (sample size may have limited the ability to discern a difference between the two groups); all drugs were administered at mid-recommended doses. Endoscopic lesions followed the same relative pattern among drugs throughout the 28-day study period, indicating that aspirin is more commonly associated with lesions in the canine gastrointestinal tract compared with the COX-1–sparing drugs. A separate study found no differences in gastrointestinal lesions in animals receiving ketoprofen (nonselective), carprofen, meloxicam, or placebo. However, the power of this latter study to detect a significant difference was not reported.
Pharmacologic prevention and treatment
Future pharmaceutical manipulations designed to deliver NSAIDs by alternative routes may decrease risk of gastrointestinal adverse drug effects, although their application to veterinary patients should not necessarily be assumed. For example, formation of nitroso derivatives of conventional NSAIDs may improve the safety margin of these drugs as a result of in vivo release of NO that provides gastroprotection while improving antiinflammatory and analgesic potency.2 However, NO also has been associated with the pathogenesis of osteoarthritis, thus exemplifying the continuously complicated nature of designing safe NSAIDs (see later discussion).22 In contrast to current drugs that only slowly dissociate with COX-1, newer NSAIDs appear to be weak and rapidly reversible binders of COX-1, thus enhancing safety.17,45
Topical (including transdermal) NSAID administration is appealing because it avoids direct contact between the drug and target tissue. Indeed, a meta-analysis of clinical trials comparing topical NSAIDs to placebos and oral NSAIDs in humans found this route to be effective, with no difference in response compared with oral. Topical administration was safe, although not necessarily safer than oral. Of the NSAIDs reviewed, ketoprofen was described as the best.84 Thus far, topical NSAID administration has not proved to be a vital means of avoiding gastrointestinal adverse drug events in small animals, primarily because of failed drug delivery. Because the amount of drug delivered cannot be predicted, administration of NSAID in novel drug delivery systems offered by compounding pharmacists is not recommended unless the amount of drug delivered by that system has been scientifically demonstrated, as would occur for an approved drug. Enteric coatings, combination drugs (e.g., with gastroprotectants), and other approaches may be of some benefit for some drugs (e.g., aspirin) and, if currently available, are discussed with the individual drugs.
Both the prevention and treatment for gastrointestinal toxicity focus on, in order of priority, control of gastric acid secretion, replacement of the missing PGs, and (for treatment) protection of the damaged mucosa.64,85 The two major categories included antisecretory drugs and the PG analog misoprostol; cytoprotectants, such as sucralfate, also play a role in treatment.
Among the antisecretory drugs, proton pump inhibitors (PPIs) generally have proved more effective than H2 receptor blockers for prevention and treatment of NSAID-induced gastrointestinal adverse drug events. An exception has been demonstrated in humans for famotidine, but only when it is administered at higher than recommended doses (40 mg twice rather than once daily).73 Boulay and coworkers86 demonstrated that cimetidine did not protect the gastric mucosa from developing lesions in dogs receiving nonbuffered aspirin (35 mg/kg every 8 hours). PPIs appeared to be more effective, as well as better tolerated, compared with misoprostol. In one human study, close to 30% of persons reciving misoprostol could not tolerate the full dose.76 Omeprazole generally is the PPI of choice, although lansoprazole may also be a reasonable choice. Although lansoprazole was not found to be superior to misoprostol for prevention of gastrointestinal adverse drug events in humans, improved compliance and better tolerance made it the preferred PPI drug in humans. However, the use of PPI will not prevent ulcers in all patients, as has been demonstrated in humans. Symptoms of GI side effects still occur in 20% of human patients receiving omeprazole.73 Although routine use of PPI in nonrisk animals receiving NSAIDs is not indicated, strong consideration should be given to their use in at-risk animals. As a class, the PPIs are inhibitors of drug-metabolizing enzymes, and care should be taken to review drug interactions before their use. Among them, esomeprazole may be the least likely to impact metabolizing enzymes.86a However, PPI also have been shown to induce selected CYP45O enzymes (P45OIA1 and A2) but this impact appears to be most clinically relevant to (human) poor metabolizers that are deficient in enzymes.86b
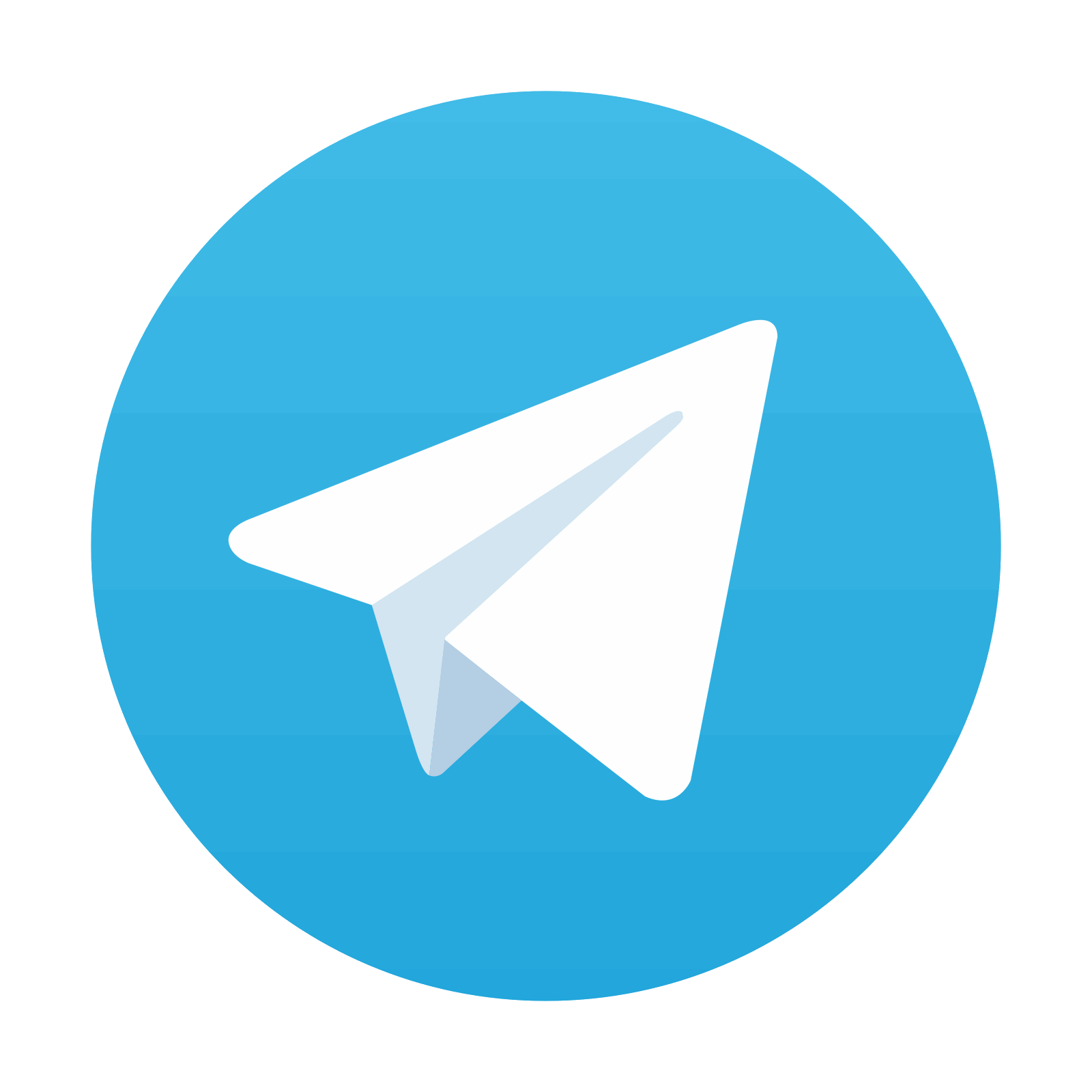
Stay updated, free articles. Join our Telegram channel

Full access? Get Clinical Tree
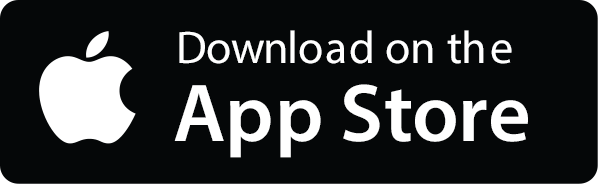
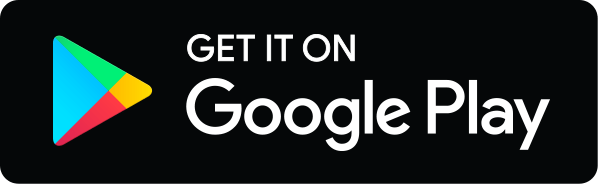
