25 Mark G. Papich There are only a small number of drugs used in veterinary medicine to affect the coagulation system. Although important coagulopathies have been identified in animals, and thromboembolism is an important component of several diseases, we lack well-controlled studies to identify the best drug to manage these problems. We often use drugs that are approved for humans to manage thromboembolic diseases but the doses and frequency of administration of these drugs in animals often is undetermined and we extrapolate doses from human medicine, or use anecdotal information to base therapy. For example, there is not a clear understanding for the most effective antiplatelet dose of aspirin to administer to dogs and cats, or whether it is even effective. Likewise, although heparin is an important anticoagulant – including the low-molecular-weight forms – the optimum dose is unclear. The conventional thinking regarding the use of anticoagulant and antiplatelet drugs is that coagulation disorders arise either on the arterial side or the venous side of the circulation. Disorders of high shear force and arterial injury induce platelets to become activated and induce a hypercoagulable state. The resulting clot is platelet rich, with platelets embedded in fibrin (the so-called white thrombi). Coagulation disorders arising on the venous side are often attributed to slow or sluggish blood and blood pooling. These clotting disorders are composed predominantly of trapped red blood cells embedded in fibrin (the so-called red thrombi). These clots can produce pulmonary thromboembolism. Therefore, treatment of clotting disorders on the venous side traditionally employ anticoagulant drugs and clotting disorders on the arterial side employ antiplatelet drugs. Obviously, the clotting disorders cannot so easily be divided into these two categories and for some diseases both components are important. For example, one of the most serious diseases for which thromboembolism plays a role is heart disease in which blood may pool in the atrium and send emboli to the pulmonary circulation, resulting in pulmonary thromboembolism. These occur in the pulmonary arteries and both antiplatelet and anticoagulant drugs have been used to prevent these problems. Dogs with immune-mediated hemolytic anemia (IMHA) have generalized thromboembolic disease of both veins and arteries and pulmonary thromboembolism (PTE) is a common complication (Kidd and Mackman, 2013). Dogs with IMHA may be at more risk because some of the drugs used to treat this disease, such as glucocorticoids and cyclosporine, may increase platelet activity (Thomason et al., 2016; Kidd and Mackman, 2013)., Evidence-based recommendations for other diseases in which thromboembolism plays a role in dogs and cats are found in the review by Lunsford and Mackin (2007). Drugs such as heparin and coumarin inhibit hemostasis by impeding clot formation. Both are used for treatment and prevention of thromboembolic disorders in animals. Newer anticoagulants – factor Xa inhibitors (rivaroxaban, apixaban) and thrombin inhibitors (dabigatran) – are becoming more popular in human medicine but their use in animals is still rare. Antiplatelet drugs in veterinary medicine are limited to aspirin and clopidogrel. We have good evidence for effectiveness of clopidogrel, but the value of aspirin as an antiplatelet agent is being questioned. There are also hemostatic agents – those intended to increase blood clotting, or fibrin formation – but these have little use in clinical veterinary medicine and will be covered only briefly in this chapter. Hemostasis refers to prevention or control of hemorrhage. Physiological control systems operate to ensure fluidity of blood under normal conditions, yet opposing systems promote coagulation when the circulatory system is invaded (Edelberg et al., 2001). Hemostasis is achieved through a series of interdependent mechanisms, including vascular spasm of the injured artery or vein, local aggregation of platelets into a plug, coagulation of the blood into a clot, and subsequent dissolution of the formed clot by fibrinolysis (Figure 25.1 and Figure 25.2). The basic process of hemostasis can be separated into the vascular, platelet, coagulation, and fibrinolysis phases. The phases overlap considerably, and events in one step promote and even cause development of subsequent phases. Figure 25.1 The sequence of events that lead to fibrin formation and clotting in response to vascular injury. Sites of drug action are shown in black ovals. See text for discussion of drugs in each group. Figure 25.2 The sequence of events, simplified here, to show how activated platelets lead to platelet incorporation into fibrin clot. Sites of drug action are shown in black ovals. Note that the source of TXA2 and ADP are from activated platelets, in which they amplify platelet aggregation by secreting platelet-activating substances. See text for discussion of drugs in each group. ADP, adenosine diphosphate; COX, cyclooxygenase; TXA, thromboxane. The vascular and platelet phases are closely allied, with the vascular endothelium having a multitude of both anticoagulant and procoagulant functions. Ordinarily, platelets circulate in an inactive state. Nitric oxide (NO) and prostacyclin (PGI2) released by vascular endothelial cells help to maintain the inactive state of platelets. Reduced levels of NO may contribute to thromboembolic disease in some animals. The endothelial cells also produce ADPase, that helps to degrade adenosine diphosphate (ADP). Adenosine diphosphate is a potent stimulator of platelet activation. Immediately after a blood vessel is injured and the subendothelium exposed, tissue factor (TF) and von Willebrand factor (vWF) are exposed, which attracts platelets to the injured endothelium. Platelets have specialized receptors for vWF and TF that facilitate this attraction to the endothelium. For platelets to properly attach to a traumatized area, vWF must be present because the platelets express a vWF integrin receptor that facilitates adherence. Animals deficient in vWF may have bleeding disorders that may be treated by medications that stimulate vWF. Agents such as desmopressin acetate (discussed in Section Desmopressin Acetate) are used for this role. Activation of platelets, in turn, initiates the coagulation factor cascade (Figure 25.1). Once adhesion has occurred, the platelets undergo a change in shape and release diverse substances that recruit further platelets to the clot and further promote the coagulation cascade. The substances involved in activation include ADP, adenosine triphosphate (ATP), serotonin (5-HT), platelet factor 3 and 4, thromboxane A2 (TXA2), and platelet-derived growth factor. This aggregation yields a rather loosely formed plug or platelet thrombus at the injury site. The smooth muscle of the vascular wall contracts and platelets adhere to the injured site (Figure 25.2). The local vasoconstrictor response, or vascular spasm, mechanically retards the flow of blood escaping from the vessel. Vascular spasm may be partly a local reflex or myogenic response and partly humoral, owing to vasoactive agents released from platelets and nearby endothelial cells. Local vasoconstriction lasts as long as 20–30 minutes, during which the ensuing phases of platelet aggregation and blood coagulation take place. Adenosine diphosphate is a potent chemical activator of platelet aggregation, which in turn activates a phospholipase that acts on membrane phospholipid to yield arachidonic acid. The latter is transformed by a platelet cyclooxygenase into short-lived but potent aggregating compounds called cyclic endoperoxides (prostaglandins [PGs] G2 and H2). (These compounds are also discussed in Chapter 20.) These endoperoxides are converted by cyclooxygenase-1 (COX-1, platelet thromboxane synthase) to the potent aggregating compound called TXA2 (Figure 25.2). Thus platelet clumping initiates formation of chemical agents that promote further platelet aggregation. In contrast, prostacyclin (PGI2) formed by COX-2 is a PG that inhibits platelet aggregation and acts as a counterbalance to thromboxane. Prostacyclin is formed from arachidonic acid and intermediate cyclic endoperoxides. Prostacyclin synthase (COX-2), the enzyme responsible for transformation of PGI2 from the cyclic endoperoxides, is localized in the vascular endothelium rather than in platelets. Once the platelets adhere and aggregate, the contractile proteins in the platelets contract and the platelet plug becomes more tightly bound and organized by incorporation of fibrin strands formed during coagulation (Figures 25.1 and 25.2). The clotting cascade is comprised of a sequence of events primarily involving a series of activation of zymogens. The zymogens are precursor proteins that are converted to an active protease by cleavage of peptide bonds in the precursor molecule. Once activated, these zymogen coagulation factors activate other factors in a sequence until the final protease, thrombin, is generated (Figure 25.1). The clotting factors are designated by Roman numerals (II, VII, VIII, IX, X, etc.; Table 25.1 ) and once activated, are shown with the “a” subscript (IIa, VIIa, Xa, etc.). Calcium also is important in these reactions. Calcium ions (Ca++), referred to as clotting factor IV (Table 25.1 ), are required for all coagulation reactions except the first two steps in the intrinsic pathway. This dependency is used in the laboratory; Ca++-complexing agents such as citrate and EDTA are used as in vitro anticoagulants (discussed in Section In Vitro Anticoagulants and Clotting Tests). Table 25.1 Blood coagulation factors and their common names Calcium chelators (e.g., EDTA used in test tubes). A plasma β globulin called plasminogen is bound to fibrin and incorporated into the clot along with other plasma constituents. Fibrinolysis is initiated when plasminogen is activated by local agents to plasmin. Tissue-type plasminogen activator is produced by endothelial cells and fibroblasts and utilizes fibrin as a cofactor in the conversion of plasminogen to plasmin. Plasmin is a proteolytic enzyme that digests the fibrin chains into soluble polypeptides, thereby preventing further fibrin polymerization. Plasmin also digests other substances in the clot and surrounding blood, for example prothrombin, fibrinogen, and clotting factors V, VIII, and XII. Formation of plasmin results in dissolution of the clot and also in hypocoagulability of the blood because of loss of clotting factors. Thus fibrinolysis represents the physiological converse of the coagulation process. It serves as a defense mechanism against overactivity of the coagulation mechanism. Antithrombin (AT, formerly called antithrombin-III) is an endogenous anticoagulant. It is a globulin with a molecular weight of 65,000 and has the ability to inactivate factors Xa, IXa, XIa, and XIIa, and thrombin. The anticoagulant activity of heparin is due to its interaction with AT. When AT binds to heparin it markedly increases the rate of inactivation of factor Xa and thrombin. Antithrombin also is called the heparin cofactor. This enzyme is an important thrombin antagonist, but it additionally inhibits activated forms of factors IX, X, XI, and XII. Antithrombin combines in a stable manner with the enzymatically active binding sites of these factors, thereby preventing their accessibility to subsequent substrates in the clotting cascade. Two categories of chemicals are used to prevent coagulation of harvested blood: those employed in samples of blood intended for physical or chemical examination and those employed to preserve blood for transfusion. Some of these agents can be used to prevent clotting both in vitro and in vivo (heparin), while others are best used only in vitro because of their toxicity (oxalates). Typically, blood clots in 4–8 minutes when added to a glass tube (red-top tube). To prevent clotting, anticoagulants may be added for blood sample diagnostic tests. The chelator ethylenediaminetetra acetic acid (EDTA) can be added to tubes to chelate calcium and prevent clotting (purple-top tube). Citric acid also can be added to bind calcium. Calcium is an important cofactor for many of the clotting factors. If calcium is then added to a decalcified tube, clotting resumes in 2–3 minutes. If negatively charged phospholipids and a substance such as aluminum silicate (kaolin) is added, the clotting is shorted to less than 1 minute. This is known as the activated partial thromboplastin time (aPTT). Another test of coagulation is the prothrombin time (PT), which is performed by adding calcium to a decalcified blood sample and then adding thromboplastin (tissue factor and phospholipids). These tests may be performed in the laboratory to detect clotting disorders and are discussed more in internal medicine and laboratory medicine textbooks. For monitoring warfarin treatment the international normalization ratio (INR) is recommended. This ratio is derived from a normalization of the PT clotting test. Prothrombin times are reported in seconds and recorded as a ratio of the prothrombin time of the patient to the mean normal prothrombin time of the laboratory and calculated as the international normalized ratio (INR). Because test reagents can differ, the INR is the most reliable way to monitor the prothrombin time (Hirsh, 1991). The PT measurement is converted to the INR using the following formula: where the PT is the prothrombin time of the patient and reference (from pooled normal blood), and ISI is the international sensitivity index, which is supplied by the manufacturer of the test reagent and indicates the degree of sensitivity of the PT. (In most laboratories the value of ISI is 1.0, simplifying the calculation of this ratio.) The “gold standard” test for heparin activity is the measure of the plasma factor Xa. This factor is inhibited by heparin and is used for monitoring and adjusting heparin administration to people (Vandiver and Vondracek, 2012). Based on studies in people the therapeutic range for anti-Xa activity is 0.35–0.70 units anti-Xa/ml (Brooks, 2004). Because the aPTT test can produce variable results, the direct factor Xa assay is considered more reliable for monitoring heparin (Brooks, 2004). Despite these advantages, at this time, the direct factor Xa assay is not routinely available in veterinary laboratories and impractical for clinical veterinary medicine (McLaughlin et al., 2017). Moreover, it is a chromogenic assay and can be influenced by other factors that impart color changes in the sample. More recently, the thromboelastography (TEG) test has been used to diagnose hypo- and hypercoagulable states in animals (Wiinberg et al., 2008). This is a whole-blood assay that tests the viscoelastic properties of clots and provides an assessment of clot forming kinetics and clot strength. The test has become valuable in veterinary medicine to assess hemostasis and is available in many of the large veterinary hospitals. Other anticoagulant agents used in laboratory examination of blood include sodium oxalate in a concentration of 20% at 0.01 ml/ml (2 mg/ml) blood, and sodium citrate in a concentration of 25% at 0.01 ml/ml (2.5 mg/ml) blood. EDTA (mentioned above in this section) can prevent coagulation when used in a concentration of 1 mg/5 ml blood. Heparin also can be used to prevent coagulation and can be added to a collection syringe (washing the syringe with heparin), added to a collection tube (green tube) or by adding 75 units to each 10 ml whole blood. Anticoagulants used for blood and blood component transfusions ideally should maintain the function of the individual components and have preservative effects. Such anticoagulant agents include: Heparin (heparin calcium and heparin sodium) can be used in vivo and in vitro to inhibit clotting. Heparin has both antithrombotic and anticoagulation effects, which are not necessarily dependent on each other. Heparin is prepared from bovine lung tissue or porcine intestinal mucosa. Both calcium and sodium salts of heparin are available for therapeutic use and occur as a white hygroscopic powder that is easily soluble in water. It is composed of polymers of alternating derivatives of α-D-glucosamine (N-sulfated, O-sulfated, or N-acetylated) and uronic acid (α-L-iduronic acid or β-D-glucuronic acid) joined by glycosidic linkages. Heparin used parenterally has a direct and almost instantaneous action on the coagulation process. By contrast, the coumarin derivatives (for oral administration) have an indirect anticoagulant effect by acting as vitamin K antagonists in the hepatic synthesis of select coagulation factors. Subsequently, action by the coumarin derivatives is delayed for several hours. The medical use of heparin consists of the conventional form of heparin, also called unfractionated heparin (UFH), and the more recent use of low-molecular-weight heparin (LMWH), which is discussed in Section Low-Molecular-Weight Heparins. Unfractionated heparin has a molecular weight of 5,000–30,000 (average 15,000). Heparin sodium is the most common form of UFH. Heparin differs from LMWH by the anti-factor Xa/anti-factor IIa ratio. Heparin has a ratio of 1 : 1, but the LMWHs have ratios of 2 : 1 or higher (ratios are given for each product listed in this chapter). The action of heparin is to potentiate the action of antithrombin (AT), discussed in Section Natural Anticoagulants. It produces a conformational change in the AT molecule that significantly enhances its inhibitory effect on various activated coagulation factors. The predominant effect of this binding is inhibition of thrombin (factor IIa), as well as factors IXa and Xa, by forming complexes. The action of heparin accelerates the activity of AT by approximately 1,000-fold. This difference in activity between UFH and LMWH is that conventional heparin (UFH) inactivates both factor Xa and thrombin, but LMWHs predominantly inactivate factor Xa because they are not large enough to bind thrombin and AT concurrently. The additional inactivation of thrombin by UFH increases the anticoagulant activity, but also increases the risk of bleeding. The dose form of heparin is standardized by units rather than mg doses. The standardization is performed by bioassay. In 2009, the standardization underwent changes because of contamination problems in people in 2007–2008 that led to serious adverse events. The contamination problems have been rectified and unitage of heparin is now formulated to an international standard. The United States Pharmacopeia (USP) standard potency is not less than 180 USP heparin units in 1 mg of dried powder. Heparin is administered to prevent and treat hypercoagulability disorders and prevent coagulation disorders such as thromboembolism, venous thrombosis, disseminated intravascular coagulopathy (DIC), and pulmonary thromboembolism. Use in specific situations in animals is primarily anecdotal or derived from the clinical experience in people. There are few outcome-based studies to evaluate efficacy to support specific guidelines for therapy in veterinary medicine. Heparin is usually administered subcutaneously (SC) because intramuscular (IM) administration may create a hematoma. After many years of administering heparin to patients with thromboembolic disorders, veterinarians have still not determined the optimal dose or dose frequency. Clinical doses to prevent thrombosis in canine patients with immune-mediated hemolytic anemia (IMHA) has not been effective (50–300 units/kg, SC, q 6 h) but when doses were adjusted based on anti-Xa activity, they were more effective and doses in individual dogs were as high as 560 mg/kg q 6 h. Unfortunately, the anti-Xa assay used in that study is not routinely available in veterinary laboratories. Heparin is also used in horses to prevent thrombosis in patients at risk, but dosage regimens are based on extrapolation from other animals or people, or clinical opinion. Heparin is definitely not a drug for which one dose fits all. A fixed dose for every patient will not produce consistent and reliable effects. As the study on treatment of IMHA (Helmond et al., 2010) demonstrated, heparin doses may vary tremendously among patients, depending on the severity of the underlying disease and circulating AT levels (Kidd and Mackman, 2013). A prospective study in dogs with IMHA showed that UFH administered at 300 units/kg SC every 6 hours was generally inadequate to attain the anti-Xa activity in a targeted range (Breuhl et al., 2009). Patients with low AT may not respond as well as those with adequate AT levels. Other variables that may affect the efficacy are variable pharmacokinetics among animals and protein binding. Some clinical syndromes result in low AT levels, which is important to the effectiveness of heparin. Ideally, dose adjustments should be tailored to individual patients by monitoring clotting times. The test most often available to veterinarians is the activated partial thromboplastin time (aPTT). Doses have been adjusted to maintain activated aPTT clotting times at 1.5 to 2.5 times normal. A measurement of anti-factor Xa activity is considered the “gold standard” for determining whether therapeutic UFH doses are attained in people. The target range for anti-Xa activity is 0.35–0.70 units/ml (based on recommendations from human medicine). The problem is that the ideal range for measuring activity in dogs, cats, and horses is not established, and availability of the anti-factor Xa test is not available in most veterinary laboratories. The conclusion in a review paper was that adjusting heparin therapy based on aPTT prolongation does not consistently correlate with anti-Xa activity in dogs (Kidd and Mackman, 2013). In healthy dogs a dose of 500 units/kg SC every 6 hours was not reliable and treatment should be monitored in each patient (Mischke et al., 2001). Because it has been difficult to derive scientifically based dosage regimens, the doses in animals are primarily based on anecdotal uses. Heparin sodium is available in 1000- and 10,000-units/ml injection vials. For dogs and cats the most common dose used for low-dose prophylaxis is 70 units/kg q 8–12 h SC. For treatment of on-going coagulation problems the dose for dogs is 100–200 units/kg IV loading dose, followed by 100–300 units/kg q 6–8 h SC. The dose is adjusted by monitoring, which may require an increase to 500–600 units/kg if necessary. In more severe cases and high-risk patients, it is recommended to start with 500 units/kg SC as the initial dose, then administer 500 units/kg q 12 h with follow-up monitoring to adjust the dose. To administer a constant rate infusion, use a loading dose of 100 units/kg IV, followed by CRI 18 units/kg/h. The initial dose for cats is 300 units/kg SC, q 8 h. As with dogs, increase to 500 units/kg if necessary. The typical dose recommended for horses – and used in other large animals – is an initial dose of 150 units/kg SC, followed by 125 units/kg SC q 8–12 h (Moore and Hinchcliff, 1994). These authors discuss the various clinical uses that include jugular vein thrombosis, laminitis, and complications from surgery. The pharmacokinetics in horses are nonlinear and the activity cannot be predicted from plasma heparin pharmacokinetics (McCann et al., 1995). Therefore, monitoring of aPTT is needed to individually adjust the dose. It is important to note that the doses listed above have not been tested in clinical studies; they are extrapolated from human medicine or based on recommendations from veterinary specialists. Adverse effects are caused by excessive inhibition of coagulation and produce bleeding problems in patients. Heparin-induced thrombocytopenia, a problem in people, has not been cited as a problem in animals. If excessive anticoagulation and bleeding occur as a result of an overdose, protamine sulfate should be administered to reverse heparin therapy (discussed in Section Reversing Agent for Heparin: Protamine Sulfate). It should not be used in animals unless there is an ability to monitor in order to prevent life-threatening bleeding. Low-molecular-weight heparins (LMWH) used in veterinary medicine include tinzaparin (Innohep®), enoxaparin (Lovenox®), and dalteparin (Fragmin®). Most of the veterinary experience has been with dalteparin and enoxaparin. As discussed below, these drugs have several advantages in people over the conventional UFH (Weitz, 1997). These advantages include greater safety, as well as more favorable and predictable pharmacokinetics. However, as described in Section Indications and Clinical Uses, these advantages have not been established for the veterinary uses. Dalteparin (Fragmin) is characterized by a molecular weight of approximately 5000, compared to conventional heparin (UFH) with a molecular weight of approximately 15,000. Subsequently, the absorption, clearance, and activity of LMWH differ from UFH. Like the conventional forms of heparin, LMWHs produce their effect by binding to antithrombin (AT) and increasing antithrombin–mediated inhibition of synthesis and activity of coagulation factor Xa. However, LMWH, unlike conventional heparin, produces less inhibition of thrombin (factor IIa). LMWH’s activity is described by the anti-factor Xa/anti-factor IIa ratio. For dalteparin, the ratio is 2.7 : 1 (conventional UFH ratio is 1 : 1). In people, LMWHs have several advantages compared to UFH and include greater anti-Xa/IIa activity, more complete and predictable absorption from injection, longer duration, less frequent administration, reduced risk of bleeding, and a more predictable anticoagulant response. However, in dogs and cats, the half-life of LMWH is much shorter than in humans, reducing some of this advantage. In dogs the half-life of dalteparin is approximately 2 hours; in cats it is estimated to be 1.5 hours, which requires much more frequent administration in either species to maintain anti-Xa activity compared to humans. Cats need higher doses and shorter dose intervals than people. Pharmacokinetic properties in horses were similar to humans (Schwarzwald et al., 2002). Dalteparin, like other LMWHs, is used to treat hypercoagulability disorders and prevent coagulation disorders such as thromboembolism, venous thrombosis, DIC, and pulmonary thromboembolism. Clinical indications are derived from uses of conventional heparin or extrapolated from human medicine. It is not possible to extrapolate doses from a unit-for-unit exchange with other heparins. There have been few clinical studies to examine efficacy of LMWH in animals. Previously published doses extrapolated from humans (100 units/kg q 12 h, SC) have been shown not to produce adequate and consistent anti-Xa activity in dogs and cats (Alwood et al., 2007). When administering LMWH, aPTT and PT clotting times are not reliable indicators of therapy, although prolonged aPTT is a sign of overdosing. In people, anti-Xa activity is considered the preferred laboratory measure of LMWH activity. Peak anti-Xa activity occurs 2 hours after dosing, and the target range for anti-Xa activity should be 0.5–1.0 U/ml for cats and 0.4–0.8 U/ml for dogs. However, the anti-Xa activity assay is not available in most veterinary hospitals, is impractical, and has produced an inconsistent attainment of the targeted range in dogs (Lynch et al., 2014). The formulations of dalteparin available are 2500 units anti-factor Xa (16 mg dalteparin sodium) per 0.2 ml in a single-dose syringe; 5000 units anti-factor Xa (32 mg dalteparin sodium) per 0.2 ml in a single-dose syringe; and 10,000 units anti-factor Xa (64 mg dalteparin sodium) per ml in a 9.5 ml multiple-dose vial. The multiple-dose vial should be used within 2 weeks of initial penetration. Although the doses have not been tested for efficacy, the most commonly recommended dose for dogs is 150–175 U/kg q 8 h SC and, if monitoring is available, doses can be further adjusted. For cats, a dose of 100 units/kg SC every 12 hours failed to produce a sustained anti-factor Xa activity (Alwood et al., 2007) and higher doses of 150 units/kg q 4 h SC, to 180 units/kg q 6 h SC are recommended. There is even less known for horses, but one dose that has been recommended is 50 units/kg/day SC. The dose may be increased in high-risk patients to 100 units/kg/day. In a study comparing dalteparin in horses to UFH, there were fewer adverse effects with dalteparin, and better efficacy for preventing thrombosis in the dalteparin treatment group (Feige et al., 2003). The dose administered (50 units/kg) produced the desired anti-Xa activity recommended for people (Schwarzwald et al., 2002; Feige et al., 2003). Although better tolerated than conventional heparin, bleeding can be a risk. LMWHs are associated with a lower incidence of heparin-induced thrombocytopenia in people, but heparin-induced thrombocytopenia from any form of heparin has not been a clinical problem in animals. If excessive anticoagulation and bleeding occur as a result of an overdose, protamine sulfate should be administered to reverse heparin therapy (discussed in Section Reversing Agent for Heparin: Protamine Sulfate). If dalteparin is administered IM, it may produce a hematoma; therefore, SC administration is preferred. Because LMWHs are excreted by renal clearance in animals, if renal disease is present, the elimination will be prolonged. Rebound hypercoagulability may occur after discontinuation of heparin treatment; therefore, it may be advised to taper the dose slowly when discontinuing treatment. Heparins interact with other drugs and it is not advised to mix these preparations with other injectable drugs. It should be used cautiously in animals that are already receiving other drugs that can interfere with coagulation, such as aspirin, clopidogrel, and warfarin. Although a specific interaction has not been identified, use cautiously in animals that may be receiving certain chondroprotective compounds such as glycosaminoglycans for treatment of arthritis. In people, enoxaparin has largely replaced dalteparin for routine use. Enoxaparin (Lovenox) is characterized by a molecular weight of approximately 5000, compared to conventional heparin (unfractionated) with a molecular weight of approximately 15,000. Like dalteparin, the absorption, clearance, and activity of LMWH differ from UFH, and like dalteparin the mechanism of action is the same. The anti-factor Xa/anti-factor IIa ratio for enoxaparin is between 3.3 and 5.3 : 1 according to the USP standard, which is higher than dalteparin and higher than conventional UFH that has a ratio of 1 : 1. As with dalteparin, there are several advantages in people for enoxaparin over conventional UFH. But, as found with dalteparin, in dogs and cats the half-life of LMWH is much shorter than in humans, reducing some of this advantage. Similar to studies with dalteparin, cats require higher doses and shorter dose intervals than people (Alwood et al., 2007). In dogs the half-life of enoxaparin is approximately 5 hours (Lunsford et al., 2009); in cats it is estimated to be 1.9 hours, which requires much more frequent administration in either species to maintain anti-Xa activity compared to humans. In horses, the pharmacokinetics are similar to humans (Schwarzwald et al., 2002).
Anticoagulant, Antiplatelet, and Hemostatic Drugs
Hemostasis
Vascular and Platelet Phases
Involvement of Prostaglandins
Coagulation Phase
Factor
Common name
Drug inhibitor
Factor I
Fibrinogen
Factor II
Prothrombin
Heparin
Factor III
Tissue thromboplastin
Factor IV
Calcium
Factor V
Proaccelerin
Factor VII
Proconvertin; serum prothrombin conversion accelerator
Warfarin
Factor VIII
Antihemophilic factor
Factor IX
Plasma thromboplastin component; Christmas factor
Warfarin
Factor X
Stuart–Prower factor
Heparin, rivaroxaban
Factor XI
Plasma thromboplastin antecedent
Factor XII
Hageman factor
Factor XIII
Fibrin stabilizing factor
Fibrinolysis Phase
Natural Anticoagulants
In Vitro Anticoagulants and Clotting Tests
Heparin
Indications and Clinical Uses
Adverse Effects
Low-Molecular-Weight Heparins
Dalteparin
Indications and clinical uses
Adverse reactions and precautions
Enoxaparin Sodium
Clinical use
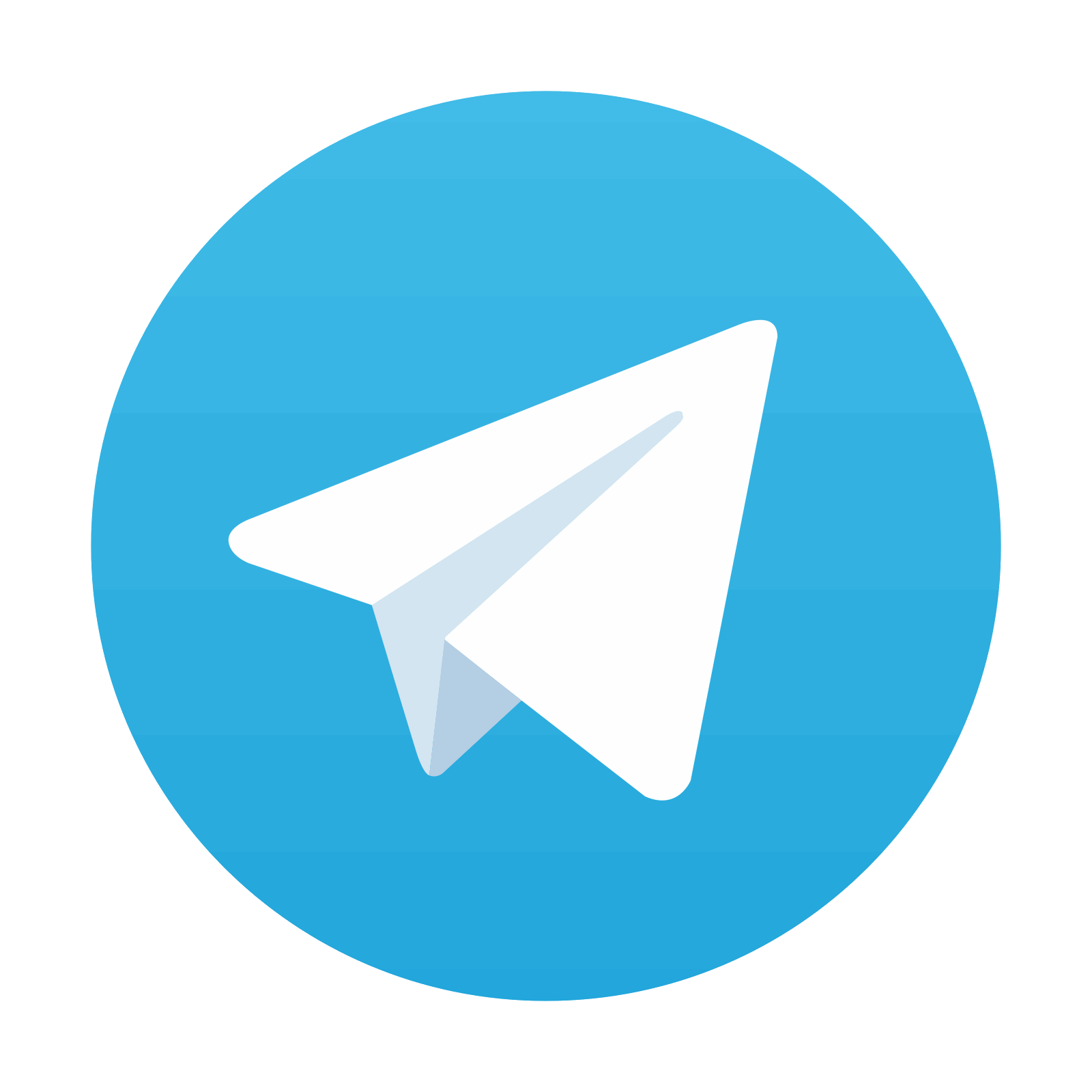
Stay updated, free articles. Join our Telegram channel

Full access? Get Clinical Tree
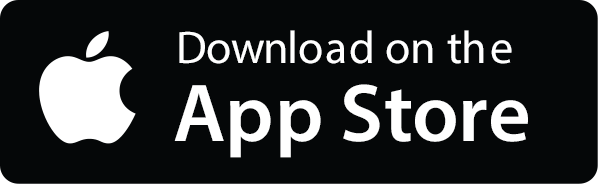
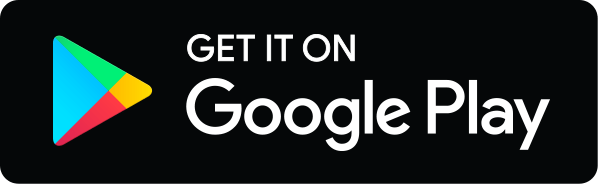