Fig. 1.
A comparative overview of spinal cord blood vessels often targeted when modeling ischemic spinal cord injury caused by aortic cross clamping. In humans, the anterior spinal artery and two smaller posterior spinal arteries are the main vessels supplying the spinal cord. These latter spinal arteries receive radicular branches from the intercostal arteries in the thoracic region, while the arteria radicularis magna (ARM) is the primary arterial blood supply to the lower two-thirds of the spinal cord (usually between T11–L3). The cauda equina is supplied by lower lumbar, iliolumbar and lateral sacral arteries.Differences between spinal cord blood supply in humans and other species: Mice: similar to human (2); Rat: hetero-segmental aorta, ARM origin at T8–L2 (3–5); Rabbit: Uniform homosegmental blood supply; main blood supply to spinal cord is from segmental branches of the abdominal aorta (6); Dogs: ARM arises from the fifth lumbar artery (7–10); Pigs: Internal thoracic and subscapular arteries are larger than in humans – providing extensive collateral flow to the spinal cord. Segmental thoracic and lumbar arteries are larger than in humans (11); Sheep: No details about the spinal cord blood supply in sheep; Primates: Virtually identical to that in humans. The anterior spinal artery is continuous in man and baboon, which was not true in rabbits, dogs or pigs (12, 13).
The number, size, and location of the radicular arteries varies. At each level of the spinal column, a radicular artery enters the intervertebral foramen to supply the spinal cord. At the thoracic and lumbar regions of the cord, segmental spinal arteries originate from the posterior branches of the intercostal and lumbar arteries, respectively. However, the origin of these vessels in the cervical and sacral regions is variable. In the cervical region they arise from the vertebral artery or the subclavian artery (via the ascending cervical branch of the thyrocervical trunk or the deep cervical branch of the costocervical region). The sacral segmental spinal arteries most frequently arise from the lateral sacral artery, but can originate from the iliolumbar, middle sacral, fifth lumbar or obturator artery (14).
The Artery of Adamkiewicz (also known as the arteria radicularis magna or ARM) is the largest of the radicular arteries and is the principle supply of arterial blood to the thoracoabdominal region of the spinal cord (Fig. 1; usually found between T11–L3). The Artery of Adamkiewicz is of great clinical importance, especially in regard to aortic aneurysm surgery. Preoperative localization of the Adamkiewicz artery and its segmental supplier before thoracic and thoracoabdominal aortic aneurysm repair can help to prevent postoperative paraplegia (15).
3 Animals Models of ISCI
3.1 Mouse ISCI Models
Generally, the blood supply of the mouse spinal cord is similar to that of humans. The ASA originates from the vertebral arteries and <10 anterior radicular arteries join it. Two posterior spinal arteries are supplied by several radicular arteries, which form large anastomoses around the spinal cord (2).
To date, four different models of mouse ISCI have been published (Table 1); three used an open surgical approach with different locations of AXC (Fig. 2), and the fourth induced ischemia via photochemical thrombosis of the dorsal spinal cord blood supply (16). The Lang-Lazdunski et al. and Wang et al. models cause immediate paralysis, while the Awad et al. model causes delayed paralysis with reproducible spinal cord pathology. The application of systemic hypothermia is thought to be responsible for promoting survival for months after ischemia/reperfusion (18). This low mortality and long-term survival allows scientists the opportunity to study the consequences of ischemia/reperfusion for many weeks or months after the insult. Mouse models of ISCI also offer an additional advantage, i.e., the ability to manipulate genetics to understand specific mechanisms that cause or contribute to ischemic neuronal injury.
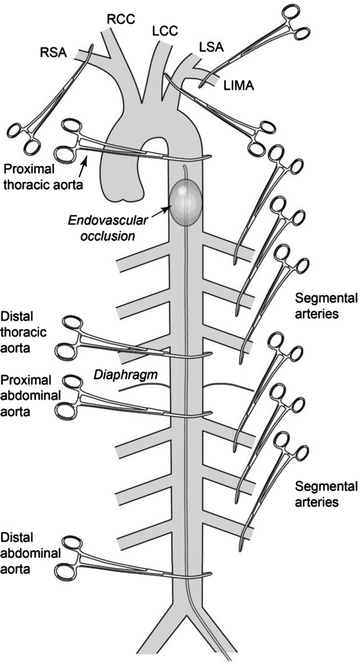
Table 1
Mouse models of ischemic spinal cord injury
References | Anatomical location of induced ischemia | Technique and outcome of induced ischemia |
---|---|---|
Lang-Lazdunski et al. 2000 (2) | Left internal mammary artery, the aortic arch between the left common carotid artery and the left subclavian artery (LSA) and the LSA at its origin | •Approach: Cross clamping the three vessels through a ventral midline cervico-thoracic incision. •Ischemia time: 9 or 11 min followed by perfusion for 24 or 48 h. •Temperature: 36 °C. •Clinical outcome: All animals developed immediate paralysis. Recovery from paralysis was dependent on the duration of ischemia. Mortality was 21% after 11 min of ischemia, while no mortality was reported after 9 min of ischemia. |
Gaviria et al. 2006 (16) | T8 vertebrae | •Approach: Photochemical spinal cord ischemia by intravenous injection of Rose Bengal dye with 8 min irradiation of exposed spinal cord. •Post-ischemic changes were recorded by ex vivo magnetic resonance imaging and histological analysis. •Clinical outcome: After 4 h of injury, alterations of the spinal cord dorsal and lateral white matter were reflected by a progressive loss of white/grey matter contrasted with further ventral extension by 24 h. |
Wang et al. 2010 (17) | Descending aorta at T8 | •Approach: Aortic cross clamping (AXC) through left thoracotomy along the eighth rib. •Ischemia time: 0, 8, 10, 12 min. •Clinical outcome: All mice had acute weakness of the hind limb at 1 h post-ischemia and 100% motor deficit within 24 h with 30–50% recovery of normal motor functions by 7 days. Eighty percent of mice survived 28 days and all of had severe motor deficits immediately after injury. |
Awad et al. 2010 (18) | Descending aorta 1 mm distal to LSA origin | •Approach: AXC through left thoracotomy between second and third ribs. •Ischemia time: 3–11 min @ 33 °C, 35 °C, or 36 °C. •Clinical outcome: The onset of paralysis was delayed for 24–36 h after reperfusion and 70% of mice developed severe hind limb paralysis. More than 95% of mice survived up to 9 weeks after surgery. |
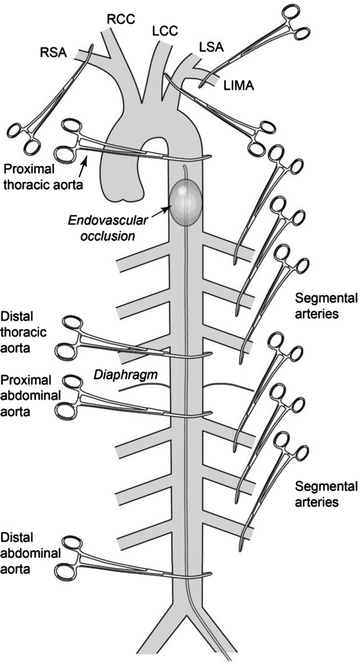
Fig. 2.
Anatomical location(s) of aortic occlusion in animal models of spinal cord ischemia. (RSA, right subclavian artery; RCC, right common carotid artery; LCC, left common carotid artery; LSA, left subclavian artery; LIMA, left internal mammary artery). Different approaches used to induce spinal cord ischemia in experimental studies – species (ref): LIMA + LSA + proximal thoracic aorta: Mouse (2), Proximal thoracic aorta: Mouse (18); Rat (19, 20); Dog (21, 22); Sheep (23), Proximal and distal thoracic aorta: Rat (24); Dog (25); Primates (26, 27), Proximal abdominal aorta: Rat (28); Rabbit (29); Dog (30), Proximal and distal abdominal aorta: Rat (31), Segmental arteries: Rabbit (32); Dog (33); Pig (34), Endovascular occlusion: Rat (35, 36); Rabbit (37); Dog (38).
3.2 Rat ISCI Models
Tveten compared the arrangement of spinal cord blood vessels in humans and rats, and noted some similarities, but also several differences (3, 4). For example, blood supplied by radicular arteries to the lower cervical and upper thoracic segments is increased in rat models compared to humans. Tventen also concluded that rats possess an ARM; however, its location was less variable, located slightly rostral to where it is found in humans. Also, with few exceptions, anastomoses between the ventral and the dorsal spinal arteries did not occur in rats. In contrast, Schievink et al. concluded that a human equivalent of the ARM does not exist in rats (5). Although current models were developed assuming that rats possess an ARM, further comparative studies are needed to fully characterize rat spinal cord vasculature. Differences in collateral blood flow and/or the location of cross-clamping relative to these poorly defined collateral systems may have influenced the variability that existed in the different reported rat ISCI models (see below and Table 2).
Table 2
Rat models of ischemic spinal cord injury
Study | Anatomical location of induced ischemia | Technique and outcome of induced ischemia |
---|---|---|
Matsushita and Smith 1970 (19) | Thoracic aorta | •Approach: Aorta was exposed through left thoracotomy between the third and fourth ribs and occlusion was done by clip application. •Ischemia time: 15–60 min. •Clinical outcome: With occlusion time <20 min, n = 1/5 rats exhibited extensor rigidity. The remaining n = 4 regained normal motor activity within 24 h after the operation. Occlusion of the aorta for >30 min caused death within 24 h in most animals. |
Coston et al. 1983 (35) | Descending thoracic aorta | •Approach: Aortic occlusion with 2F Fogarty catheter introduced through the femoral artery. •Ischemia time: 13–20 min. •Clinical outcome: Permanent hind limb motor deficits in 75% of operated animals. |
Le May et al. 1987 (20) | Aortic arch between the left common carotid and left subclavian artery (LSA) | •Approach: A snare was placed around the aorta through a longitudinal incision in the sternal region. •Ischemia time: 10, 15, 20 min. •Clinical outcome: 10 min occlusion caused mild neurological deficits with almost complete recovery after 24 h. Occlusion for 15 min caused intermediate but persistent deficits with some improvement by 24 h. The 20 min occlusion caused severe paralysis with minimal improvement over 24 h. |
Marsala and Yaksh 1994 (36) | Descending thoracic aorta at the level of LSA | •Approach: Aortic occlusion by insertion and inflation of 2F Fogarty catheter inserted through the tail artery. •Ischemia time: 10, 15, 20, 30 min. •Clinical outcome: 30 min of ischemia caused hindlimb flaccidity at 1 h, spasticity at 4 h, and flaccidity at 8 h. After 20 min of ischemia, initial flaccidity was followed by hind limb spasticity that persisted for 8 h. Ischemia for shorter intervals had minimal effects on motor function. |
Lopez et al. 1995 (24) | Descending thoracic aorta at the level of the third and the tenth intercostal spaces | •Approach: Two left thoracotomies at the third and tenth intercostal spaces. The thoracic aorta was occluded by 2-0 silk snare. •Ischemia time: Different time intervals. •Clinical outcome: When the aorta was cross-clamped for 15 min or less, all animals recovered completely. Beyond 15 min, the incidence of paraplegia increased proportionately with the duration of aortic occlusion. When the aorta was double cross-clamped for 40 min, none of the rats survived. Aortic occlusion for 30 min was used in follow-up studies in which addition of HCO3 (20 mM) to the hypothermic (15 °C) solution was found to be neuroprotective. |
Usul et al. 2004 (28) | Abdominal aorta just distal to the left renal artery | •Approach: An aneurysm clip was applied to the abdominal aorta through a retroperitoneal approach. •Ischemia time: 45 min. •Clinical outcome: Significant neurological deficits in rats after 45 min of aortic occlusion. |
Pashin and Viktorov 2009 (39) | Thoracic aorta | •Approach: Photochemical spinal cord ischemia by intravenous (jugular) injection of Bengal pink dye with irradiation of exposed spinal cord. •Ischemia time: Duration of radiation. •Clinical outcome: Histology revealed the necrosis at location of focal ischemia 1 day after surgery. |
Guler et al. 2010 (31) | The abdominal aorta just distal to the left renal artery and proximal to the aortic bifurcation | •Approach: Double cross clamping of the aorta via vertical midline incision. •Ischemia time: 45 min. •Clinical outcome: Significant neurological impairment with histological evidence of ischemic necrosis. |
3.2.1 Thoracic Aortic Occlusion in Rat Model of ISCI Using Open or Endovascular Approach
Matsushita and Smith developed a rat model to study the effect of AXC on hind limb spasticity (19). In 1987, Le May et al. developed a rat model of ISCI (20) to replace the rabbit model. At that time, this rabbit model was widely used as a nonspecific representation of neuronal ischemic injury (29, 40–42). Soon after, Le May et al. made additional modifications to the rat model (43). They used the same incision to explore the thoracic aorta, but instead occluded the right and left subclavian arteries and the thoracic aorta for 10 min. Neurological deficits were recorded at different time intervals.
Coston et al. developed another rat model of ISCI using an endovascular approach (35). Specifically, a 2F Fogarty catheter was inserted through the femoral artery into the aorta and was inflated. Fifteen minutes of aortic occlusion caused permanent hind limb motor deficits in 75% of the operated rats. Marsala and Yaksh modified the Coston et al. model by inserting the 2F Fogarty catheter through the tail artery into the thoracic aorta until the catheter tip reached the level of the left subclavian artery (35).
3.2.2 Thoracic Aortic Occlusion in Rat Model of ISCI with Hypovolemia
3.2.3 Abdominal AXC in Rat Model of ISCI
Models in which cross clamping of the abdominal aorta has been used to cause spinal cord ischemia have produced variable results. Usul et al. and Guler et al. reported significant neurological deficits in rats after 45 min of aortic occlusion (28, 31). In contrast, Carrillo et al. reported that sub-diaphragmatic AXC is not suitable for causing spinal cord ischemia in rats because this method does not increase blood and tissue concentrations of pyruvate, glucose, or lactate relative to a non-ischemic control population (45). Nouri et al. also concluded that abdominal AXC is not a reliable method to induce spinal cord ischemia in rats (46) because the clamp is placed distal to the origin of the artery of Adamkiewicz (ARM). In rats, the origin of the artery of Adamkiewicz lies slightly more rostral to segments of the thoracic spinal cord (relative to human). Consequently, blood supply to the lumbosacral spinal cord is largely unaffected (46).
3.3 Rabbit ISCI Models
Recently, Mazensky and colleagues found that contrary to other laboratory animals, the blood supply to the spinal cord of rabbits is homo-segmental; each spinal cord segment is supplied with one corresponding radicular artery with minimal or no collateral bloodstream (6). They reported high variability of segmental arteries supplying blood to the ASA inside the spinal canal. Also, when studying the ARM which supplies the caudal half of the spinal cord, a bigger feeding artery arising from the spinal branch of the sixth lumbar artery was observed. Concordantly, there was no collateral blood supply coming from the thoracic spinal cord. These findings suggest the rabbit model is more susceptible to ischemia after abdominal AXC. Table 3 provides a summary of publications detailing rabbit ISCI models.
Table 3
Rabbit models of ischemic spinal cord injury
Study | Anatomical location of induced ischemia | Technique of induced ischemia |
---|---|---|
Zivin and DeGirolami 1980 (29) | The abdominal aorta just caudal to the left renal artery | •Approach: Exposure of the aorta through abdominal incision. Occlusion was done by a clip. •Ischemia time: 1 h. •Clinical outcome: All animals (n = 40) were paraplegic, incontinent and unresponsive to noxious stimuli in the hindlimbs. |
Cheng et al. 1984 (37) | Abdominal aorta just distal to the left renal artery | •Approach: Abdominal aorta was occluded by a balloon catheter that was introduced through the femoral artery. •Ischemia time: 15–60 min. •Clinical outcome: Frequency of paraplegia varied as a function of ischemia duration: 0% after 15 min, 30% after 17 min, 33% after 20 min, 38% after 25 min, and 100% after 60 min of ischemia. |
Maeda et al. 2003 (32) | Five lumbar arteries (L3–L7) | •Approach: Selective occlusion of the five lumbar arteries through a flank incision. •Ischemic time: Variable. •Clinical outcome: Occlusion of the lumbar arteries for 10 and 20 min induced paraplegia within 24 h after ischemia in 2/6 and 4/6 rabbits, respectively. Delayed paraplegia was observed in one rabbit after 10 min ischemia and another one after 20 min ischemia. |
3.3.1 Abdominal Aortic Occlusion in Rabbit Model of ISCI with Open or Endovascular Approach
Zivin and DeGirolami developed the first reproducible rabbit model of ISCI in 1980 (29). Their intention was to develop a central nervous system ischemia model to avoid variability and clinical complications associated with modeling infarction in the cerebral arteries. They attributed the reproducibility of their model to the uniformity of the spinal cord vasculature in rabbit models, i.e., the main source of blood in the caudal spinal cord is segmental from the abdominal aorta and can be interrupted by a single AXC (29). They later modified their technique of AXC by placing a snare ligature around the abdominal aorta, with the free end of the snare accessible outside the rabbit (40). In related studies, a subcostal incision was performed to expose the infra-renal aorta through a retroperitoneal approach, instead of a midline laparotomy. The use of an externalized snare ligature enabled investigators to cause repeated bouts of ischemia in the conscious animal (47–51).
Cheng et al. used a less invasive approach (52). A balloon catheter was introduced through the femoral artery and advanced into the abdominal aorta until it was just distal to the left renal artery. Occlusion of the aortic lumen was accomplished by inflation of the balloon. This approach has been applied in diverse rabbit models of SCI because it is simple, fast, and less invasive than ligating the aorta through a laparotomy (53–59).
3.3.2 Rabbit Model of ISCI Using Selective Occlusion of Lumbar Arteries
Maeda et al. developed a new rabbit model of SCI (32). Instead of the classical technique of infra-renal AXC, they selectively occluded the five lumbar arteries (L3–L7), utilizing a flank incision. Their goal was to avoid the hemodynamic changes associated with AXC, which include proximal hypertension with subsequent increase in CSF pressure and distal hypotension with subsequent ischemia of the bowel and lower extremities. By selective occlusion of the lumbar arteries, blood flow in the aorta was maintained, but the blood supply to the lumbar spinal cord was still interrupted (Table 3).
The rabbit model with infra-renal AXC is widely used as a model of ISCI. Although the rabbit model is reproducible, owing this quality in part to the simplicity of the surgical approach and the low incidence of adverse reactions associated with the procedure, it does not mimic the consequence of AXC in humans. Indeed, infra-renal AXC in humans undergoing abdominal aortic aneurysm repair rarely causes paraplegia (60, 61).
3.4 Dog ISCI Models
The blood supply to the canine spinal cord is similar to that of humans (Fig. 1). Each spinal segment is supplied by intercostal or lumbar arteries branching from the aorta (7), and like the human spinal cord, rich anastomotic vascular networks exist, especially at the level of the conus medullaris (8). In most dogs, the origin of the ARM lies caudal to the origin in humans; arising from the fifth lumbar artery after following a course similar to the human ARM (8–10). Because dogs have extensive collateral pathways from the aorta to the cord, their motor neurons at any spinal level may survive irreversible ischemic damage when the aorta is cross-clamped, even at high thoracic levels (22).
The idea of using dogs to model ISCI was described long ago. In 1667, Niels Stensen described a procedure he performed on dogs as such: “. . . . on making a ligature in the descending aorta, without previous cutting, that voluntary movement of all the parts below ceased in proportion to the tightness of the band, while it was restored similarly as the knot was loosened” (29). Since then, multiple techniques have been used to cause ISCI in dogs (Table 4).
Table 4
Dog models of ischemic spinal cord injury
Study | Anatomical location of induced ischemia | Technique of induced ischemia |
---|---|---|
Gelfan and Tarlov 1959 (62) | Thoracic aorta | •Approach: Adopted from Tureen’s technique for cats (1963) (63). •Ischemia time: 40–50 min. •Clinical outcome: More than 60% of 120 dogs subjected to 40–50 min of aortic occlusion survived the stress. Sixty-two percent of the survivors exhibited permanent rigidity of the hind limbs. The rest exhibited either permanent flaccid paralysis or temporary weakness or were normal. |
Nylander et al. 1982 (30) | The abdominal aorta caudal to the renal artery | •Approach: Permanent occlusion of the abdominal aorta. •Clinical outcome: Paraplegia incidence was 90% in the ischemic group of animals within 24 h post ischemia. High mortality was reported in the ischemic group after 48 h; only 1 animal survived out of 10. |
Coles et al. 1982 (25) | The left subclavian artery (LSA), the thoracic aorta just distal to the LSA and the distal thoracic aorta at the level of the diaphragmatic hiatus | •Approach: Left thoracotomy through the fourth and the eighth intercostal spaces. •Ischemia time: Different time intervals. •Clinical outcome: Twelve animals subjected to aortic occlusion demonstrated a characteristic time-related deterioration of the somatosensory evoked potentials (SEPs). |
Laschinger 1984 (21) | The thoracic aorta distal to the LSA | •Approach: Single aortic cross clamping (AXC) just distal to the origin of the LSA via a small thoracotomy. •Ischemia time: Different time intervals guided by loss of SEPs. Clinical outcome: Animals with AXC for 5 min after loss of SEPs recovered without neurological deficit. Animals underwent AXC for 10 min after loss of SEPs sustained a 67% incidence of permanent spastic paraplegia. |
Kaplan et al. 1987 (38) | The thoracic aorta and positioned 1 cm distal to the origin of LSA | •Approach: A balloon catheter was introduced through the carotid artery into the thoracic aorta and ischemia of the spinal cord was induced by inflation of the balloon. •Clinical outcome: Acute occlusion of the thoracic aorta (distal pressure 15–25 mmHg) was associated with a change in the SEP. Aortic occlusion for 30 min beyond an evoked potential change resulted in a moderate to severe motor deficit in all cases. Acute occlusion of the thoracic aorta (ten animals, distal pressure 15–25 mmHg) was associated with a change in the SEP. |
Kai et al. 1994 (33) | Lumbar arteries between the aortic hiatus and the bifurcation in addition to the middle sacral artery | •Approach: Baseline sciatic motor evoked potentials (MEPs) and SEPs were obtained, lumbar arteries were ligated and evoked potentials were recorded continuously. If sciatic MEPs were not lost, intercostal arteries were ligated, and potentials and clinical status were reassessed. •Clinical outcome: Following ligation of the lumbar and intercostal arteries, no change in the evoked potentials was recorded from the mid-spine; however, ischemia of the lumbar spine was recognized in all cases. |
Sugawara et al. 2005 (22) | Proximal descending aorta | •Approach: In addition to AXC, they induced hypovolemia by draining blood from the LSA. A catheter was introduced through the axillary artery to the LSA to drain the blood. •Ischemia time: 60 min with AXC only and 40 min in animals with AXC and hypovolemia. •Clinical outcome: All animals that underwent the procedure had paraplegia after 40 min of ischemia, while one-third of animals with thoracic aortic occlusion alone demonstrated no spinal cord injury, even with a longer ischemic time. |
3.4.1 Abdominal Aortic Occlusion in Dogs
Nylander et al. developed a canine model in which permanent occlusion of the infra-renal aorta was used to cause ISCI (30). The location of aortic ligation was chosen to avoid bowel infarction and kidney damage, i.e., complications that occur with occlusion of the aorta above the celiac artery or the superior mesenteric artery (64, 65).
3.4.2 Thoracic Aortic Occlusion in Dog Model of SCI
Coles et al. described a temporary thoracic aorta occlusion model of dog ISCI (25). By clamping the proximal and distal thoracic aorta, as well as the origin of the left subclavian artery (LSA), it was discovered that collateral blood flow to the spinal cord also was interrupted, thereby increasing the reproducibility of ISCI and paraplegia (63). Coles used this model to evaluate the neuroprotective effects of regional spinal cord cooling and systemic injection of a putative free radical scavenger (63, 66). Subsequent experimental studies by other groups have shown that double aortic cross clamping is also a suitable approach (with minor variations) for causing ISCI in dogs (67–75). Other researchers used single AXC (via a small thoracotomy) just distal to the origin of the LSA, to induce spinal cord ischemia in dogs (21, 76–84). Similarly, Grossi et al. (85), Sugiyama et al. (86) and Shokoku et al. (87) determined the effects of ligating the LSA, right subclavian, and/or double AXC in their models. Kumagai et al. described a different model of canine ISCI (88). This research group was able to simulate TAAA surgery through extensive clamping of the aorta and every other visceral branch. Sugawara et al. (22) added proximal hypovolemia to their model. Specifically, a significant reduction in proximal aortic blood pressure (reduced to ∼80 mmHg) was created by withdrawing blood into a reservoir while simultaneously cross clamping the descending aorta. This technique caused substantial ischemia and loss of motor function.
3.4.3 ISCI in Dogs by Selective Occlusion of Segmental Arteries
Because of the extensive collateralization of blood supply to the canine aorta, it is difficult to cause prolonged and consistent ischemia. To overcome this innate property of the canine blood supply, a number of researchers evaluated the effects of ligating lumbar and intercostal segmental arteries. By interrupting the segmental blood supply to 3–5 consecutive segments of the spinal cord (without AXC), Kato et al. (9), Fujimaki et al. (10), and Zhang et al. (89) created reproducible ISCI models. Awad et al. used AXC in addition to permanent interruption of the segmental blood supply to produce irreversible paralysis (90).
3.4.4 Dog Models of ISCI Using an Endovascular Approach
Endovascular occlusion techniques have been used to induce ISCI in dogs. Both Kaplan et al. and Ishizaki et al. used an endo-aortic occlusion approach to produce spinal ischemia in dogs (38, 91). In both cases, a balloon catheter was introduced through the left common carotid artery into the thoracic aorta, then advanced caudally to the lower abdominal aorta. The celiac and superior mesenteric arteries were ligated to eliminate collateral blood flow to the spinal cord. Graded ISCI was caused by stepwise alternation of the level of aortic occlusion via drawing the inflated balloon in the cephalic direction (91).
Overall, because dogs have extensive collateral blood supply to the spinal cord, their spinal cord neurons may escape ischemic injury after single level AXC. Additional ligation of segmental arteries (90), with or without proximal hypovolemia (22), may be required to consistently develop robust spinal cord ischemia in dogs.
3.5 Pig ISCI Models
The availability and cost-effectiveness of pigs compared to other large animals makes the swine model of ISCI a particularly attractive pre-clinical model (92). A comparative anatomical study of pig and human spinal cord vascular supplies revealed some similarities, but also several significant differences. First, pigs have larger internal thoracic and subscapular arteries than humans. This provides extensive collateral flow to the lower body, including the spinal cord. The pig also has a fine caliber vascular plexus that provides blood to the neck area, from which blood flows to reach the spinal cord and the base of the brain. Second, the pig’s spinal cord vascular anatomy is plurisegmental, but is paucisegmental (few) in humans. This feature of the pig spinal cord vasculature may render it less susceptible to ischemic injury after ligation of the segmental arteries (34, 93). The segmental thoracic and lumbar arteries are relatively small in pigs, and almost all of them originate as a single branch from the aorta then divide after 3–4 mm. The segmental vessels show a clear diminution after 2–3 cm at the level of the vertebral bodies. The median sacral artery in pigs has an isolated single dorsal branch leading to the spinal cord, and is a large caliber vessel, comparable in size to the common iliac artery (11). See Table 5 for a summary of pig ISCI models.
Table 5
Pig models of ischemic spinal cord injury
Study | Anatomical location of induced ischemia | Technique of induced ischemia |
---|---|---|
Wadouh et al. 1984 (94) | Thoracic aorta just distal to the left subclavian artery (LSA) Lumbar arteries or arteria radicularis magna (ARM) | •Two approaches: Endovascular occlusion by a balloon catheter that was inserted through the left femoral artery. Lumbar arteries or ARM were ligated through left retroperitoneal approach. •Ischemia time: Occlusion of the descending aorta for 45 min or permanent ARM ligation. •Clinical outcome: The degree of permanent spinal cord damage was 85.7% with thoracic aortic occlusion and 71.4% with permanent ARM ligation. |
Wadouh et al. 1986 (95) | The thoracic aorta at the level of T3 to T4 and T13 The abdominal aorta at the level L1 and S1 with ligation of the anterior organ arteries | •Two approaches: The thoracic aorta was exposed through two thoracotomies in the bed of fourth and twelfth ribs and the abdominal aorta was exposed through a left retroperitoneal approach. •Clinical outcome: Exclusion of the thoracic aorta by double clamping restored the intercostal bed pressure almost to control, whereas exclusion of the abdominal aorta hardly affected the lumbar bed pressure. |
De Haan et al. 1999 (34) | Segmental arteries in a cranial to caudal direction, starting just below the level of the LSA | •Approach: The thoracoabdominal aorta was mobilized, and the intercostal and lumbar arteries were exposed from the LSA to the bifurcation. Using spinal cord motor evoked potentials (MEPs), a group of segmental arteries not critical for spinal cord blood supply was identified. Before, during, and after clamping of the noncritical segmental arteries, SCI was produced by decreasing spinal cord perfusion pressure by means of increasing cerebrospinal fluid pressure. •Clinical outcome: Clamping of originally noncritical segmental arteries significantly reduced the tolerance of the spinal cord to a decrease in spinal cord perfusion pressure. |
Hellberg et al. 2000 (96) | The aorta just distal to the LSA and below the level of L1 | •Approach: The thoracic aorta was exposed through median sternotomy with extended midline incision to expose the abdominal aorta. They used a shunt between the LSA and the left iliac artery to improve the hemodynamics during spinal cord ischemia. •Ischemia time: 60 min with the shunt and 30 min without the shunt. •Clinical outcome: The shunt between the LSA and the left iliac artery improved the hemodynamics during spinal cord ischemia and obviated the need for pharmacological manipulation. |
3.5.1 Pig Model of ISCI Using Open and/or Endovascular Approaches
Wadouh et al. used both endovascular and open approaches to induce spinal cord ischemia in the pig. From their research, the anatomical location of the ARM and the efficacy of the collateral circulation were defined as critical determinants of ischemic spinal cord injury during aortic occlusion (94, 97, 98). They also studied hemodynamic changes in the spinal and aortic collateral circulation after single and double AXC (95, 99, 100).
3.5.2 Pig Model of ISCI Using Thoracic Aortic Occlusion
Like smaller species, the relative simplicity of the single AXC approach has been used by many laboratories to induce spinal cord ischemia in pigs (101–107). The proximal thoracic aorta is usually exposed through a small thoracotomy that is cross clamped distal to the LSA. Other researchers used the double cross clamping approach as a more reliable method to induce ischemia in pig models, and to mimic the surgical technique used during aortic aneurysm surgery (108–110). For double AXC, the aorta is exposed through two thoracotomies in the fourth and seventh intercostal spaces, then is cross clamped just distal to the LSA above the diaphragm (109, 110).
< div class='tao-gold-member'>
Only gold members can continue reading. Log In or Register a > to continue
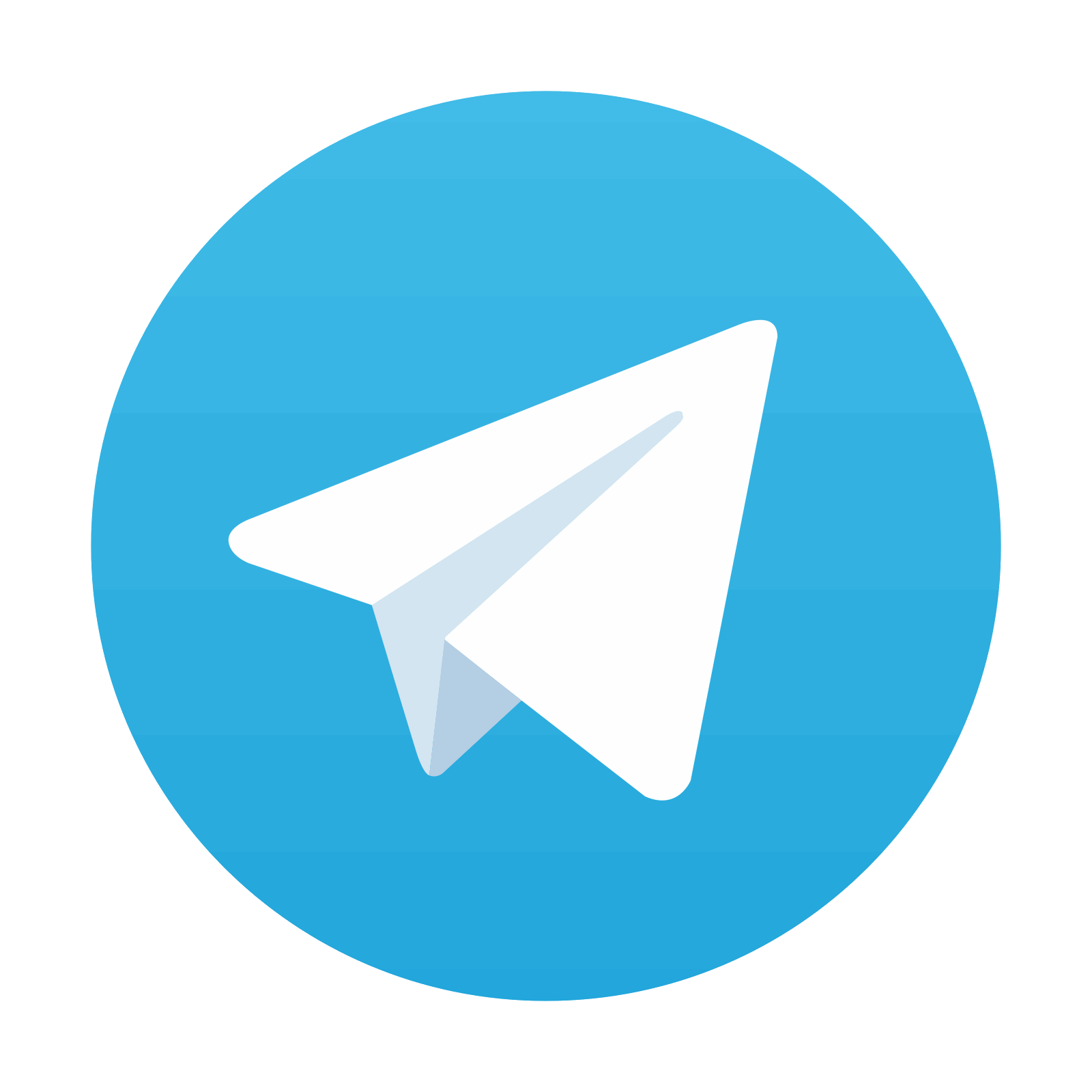
Stay updated, free articles. Join our Telegram channel

Full access? Get Clinical Tree
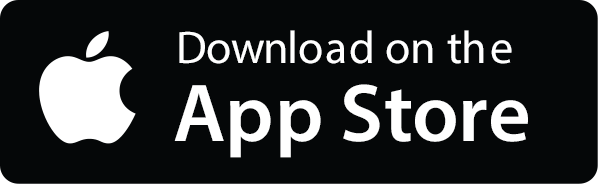
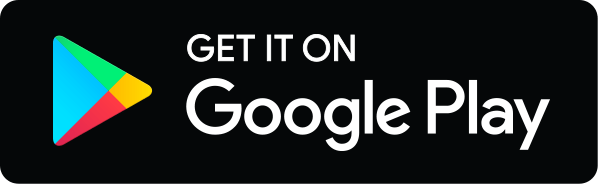