Rebecca C. Reader MSPCA/Angell Animal Medical Center, Boston, Massachusetts, USA The ability to monitor anesthetic gases is incorporated into many multiparameter physiologic monitors. Respiratory gases of interest to the anesthesiologist include oxygen, carbon dioxide, and the volatile inhaled anesthetics. Information obtained from respiratory gas monitoring can be used as a teaching tool, to aid in the determination of minimum alveolar concentrations (MAC) in research settings, and to assist in monitoring patient anesthetic depth and safety. This chapter will discuss principles and technologies associated with the monitoring of volatile anesthetic agents; information pertaining to the monitoring of carbon dioxide and oxygen gas is discussed in Chapters 14 and 15, respectively. Respiratory gas monitors may report data as partial pressure, which is an absolute term, or volume percent (vol %), which is a ratio, and understanding the difference between these two values is important. Respired gases consist of molecules in constant motion. These molecules strike the walls of the respiratory tract or anesthesia delivery system and exert pressure against those walls. At a given temperature, the pressure exerted is proportional to the number of molecules present; that is, the greater the number of molecules present, the greater the pressure exerted [1,2]. Dalton’s law of partial pressure states that the total pressure exerted by a mixture of gases is equal to the arithmetic sum of the partial pressures of each gas in the mixture [1]. At sea level, the total sum of all gases in an anesthesia system is equal to 760 mmHg (i.e., the pressure is equivalent to the surrounding atmospheric pressure) [2]. With this information, it is possible to calculate the partial pressures of each gas in the mixture. For example, for a patient administered 2% isoflurane in 100% oxygen, the partial pressure of each respiratory gas is as follows: Thus, partial pressure quantifies the number of molecules of gas that are present in absolute terms, such as millimeters of mercury (mmHg). This method is used in infrared (IR) analysis of respiratory gases [2]. As described in detail below, gas molecules with two or more dissimilar atoms (carbon dioxide and inhaled anesthetics) absorb distinct wavelengths of IR radiation. In accordance with the Beer–Lambert law, the greater the number of molecules of gas present, the more radiation of that wavelength that will be absorbed [1]. Because the total amount of radiation of a specific wavelength that is absorbed is determined by the number of molecules present, and each molecule contributes to the total pressure of the system, IR analyzers can measure and report the partial pressure of the gas in question [2]. Volume percent (vol %) separates the molecular components of a gas mixture into individual portions, and is expressed as a ratio, or proportion of the gases in the mixture [2]. Using the previous example, two molecules of isoflurane would be present in a sample containing 100 molecules, and isoflurane would represent 2% of the gas sample. This would be expressed as 2 vol %, or as a fractional concentration (0.02). This type of analysis is used with mass spectrometry. If the absolute pressure reading of the system is known, such as 760 mmHg at sea level, it is possible to convert the proportions to readings in mmHg: Monitors equipped to analyze respiratory gases must place the gas to be analyzed within proximity of the analyzer. This can be accomplished by bringing the sampled gas to the analyzer or by placing the analyzer in the path of the gas. Monitors that place the analyzer in the path of the gas are called mainstream or non‐diverting monitors, as no gas is removed from the circuit for analysis. Monitors that sample gas from the airway and bring it to the analyzer are called sidestream or diverting monitors. Anesthesia providers must be able to rapidly respond to changes in respiratory gas composition. As a result, it is important to understand the total response time of each sampling system. The total response time is typically sigmoid in shape (Fig. 16.1) and is made up of the system’s transit time and rise time [2]. The transit time (or lag time) accounts for the delay that occurs as the gas sample travels to the analyzer. Transit time applies only to sidestream monitors, as there is no delay for the sample to reach the analyzer in mainstream monitors [2]. The response time of sidestream systems depends heavily on the inner diameter and length of the sampling line as well as the gas sampling rate. Rise time refers to the time it takes an analyzer to react to a change in gas concentration, and is generally defined as the time it takes the analyzer to move from 10% to 90% of a sudden change in concentration [2]. For example, if an analyzer detected a change in carbon dioxide concentration from 0 mmHg to 50 mmHg, the rise time would be the time it took the monitor to report a change from 5 mmHg (10% of a 50 mmHg increase) to 45 mmHg (90% of the total increase). Figure 16.1 Total response time for a sidestream respiratory gas sampling system. The transit (lag) time reflects the delay that occurs as the gas sample travels to the analyzer and is generally defined as the time required to achieve 10% of the final gas concentration. Rise time refers to the time it takes an analyzer to react to a change in gas concentration and is typically defined as the time required to move from 10% to 90% after a sudden change in concentration. Source: Reproduced from Jaffe et al. [2], with permission of Elsevier. Until recently, the only respiratory gases that could be analyzed using mainstream systems were carbon dioxide (using IR technology) and oxygen (using a fuel cell). However, mainstream multigas analysis is now available for carbon dioxide, oxygen, and potent volatile inhalant anesthetics [2,3]. Mainstream multigas analyzers require a special airway adapter that uses a miniaturized spinning filter wheel placed in the breathing system near the patient’s airway. The adapters are designed to be lightweight and add only a small amount of deadspace to the patient circuit. Because the analyzer is located within the circuit, data is displayed in real time with minimal delay due to transit time for gas to reach the analyzer. Despite the lightweight design, when used with small patients, traditional mainstream adapters can be bulky, adding weight to the end of the endotracheal tube and contributing to mechanical deadspace. Some of the newer mainstream analyzers address this issue using “solid‐state” design, which relies on electronic components instead of a spinning filter wheel, making the adapters more compact and less prone to breaking. Mainstream multigas monitors are also subject to interference from water vapor, secretions, and blood [2,4]. Sidestream monitors continuously aspirate gas from the breathing circuit and pump it through tubing to an analyzer within the main unit of the monitor [4]
16
Anesthetic Gas Monitoring
Introduction to respiratory gas monitoring
Units of measurement
Partial pressure
Volume percent
Monitors
Gas sampling systems
Stay updated, free articles. Join our Telegram channel

Full access? Get Clinical Tree
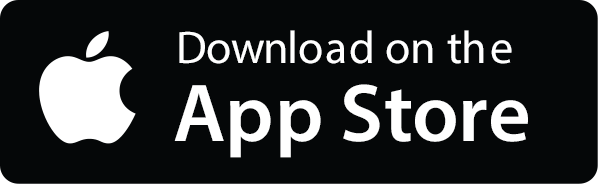
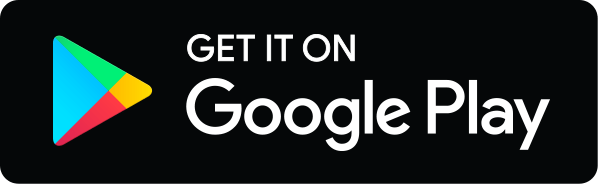