Ocular patients
General considerations
Development of an appropriate anesthetic and pain management plan for any ocular patient requires knowledge of not only the patient’s physical status and the ophthalmic procedure to be performed, but also familiarity with ocular physiology and medications administered for ophthalmic purposes.
Ocular and periocular structures are often neglected during anesthetic induction. Positioning of hands and equipment relative to the eyes should be noted during induction, especially when dealing with severely compromised globes with the potential to rupture. Mask induction may not be an option if the mask rubs or puts pressure on the eye, and patient struggling during induction with a face mask may increase intraocular pressure (IOP) or potentiate eye rupture. Providing analgesia is particularly important in patients with substantial discomfort from ophthalmic disease. It is imperative that the use of analgesic and preanesthetic drugs that are associated with vomiting be avoided in cases where brief increases in IOP (e.g., corneal laceration) may cause rupture of the eye. These patients may also be more inclined to struggle when restrained, which may result in increased IOP and additional damage to the globe.
Protection of the dependent, nonaffected eye should also be considered during patient positioning. In unilateral procedures, corneal protection of the unoperated eye may be provided by application of corneal lubrication with or without a temporary tarsorrhaphy. Positioning of the patient’s head and application of any topical ophthalmic preparations should be coordinated between the anesthesia personnel and ophthalmologist to ensure the best possible surgical outcome when both eyes are to be operated on. Resting the periocular region of the dependent eye on a soft padded eye ring or “doughnut” may help protect the eye from corneal abrasion and external globe compression that may result in hypotony.
Laryngeal stimulation should be minimized at induction and endotracheal intubation accomplished as smoothly as possible to avoid any possible increases in IOP. Lidocaine applied topically to the larynx or administered intravenously (IV) may be helpful in suppressing the cough reflex. The occurrence of increases in IOP during endotracheal intubation has not been clearly established in dogs and cats.
Positioning for the ophthalmic procedure may render ocular patients less accessible for anesthetic monitoring, and maintaining an appropriate level of anesthesia may be difficult. Eye reflexes, jaw tone, and oral mucous membranes cannot be routinely monitored although the ophthalmologist may be able to provide information about eye position and movements. Once the head has been surgically draped the airway also becomes inaccessible. A guarded (i.e., wire-reinforced) endotracheal tube is recommended to prevent unobserved kinking and occlusion of the airway during surgical positioning. Capnography is very useful for detection of an obstructed airway or inadvertent disconnection from the anesthetic delivery system. Similarly, pulse oximetry may help detect desaturation should the endotracheal tube become kinked or the delivery system disconnected. However, the pulse oximeter probe may have to be placed somewhere other than the tongue to prevent movement by the ophthalmologist interfering with its function. Since many ocular patients benefit from or require mechanical ventilation, capnometry is particularly useful to ensure maintenance of normocapnia.
Monitoring heart rate (HR) and arterial blood pressure (ABP) are crucial in ocular patients when opportunities to directly visualize the patient are limited. This is even more important when neuromuscular blocking agent s (NMB s) have been included in the anesthetic protocol, as assessment of anesthetic depth becomes more challenging in this setting. Increased ABP or HR may indicate an inadequate plane of anesthesia or the need for additional analgesics. Conversely, precipitous decreases in HR or ABP may indicate an excessive plane of anesthesia or initiation of the oculocardiac reflex (OCR). Increased respiration rate may also be indicative of inadequate anesthesia but such a response will not be evident in mechanically ventilated patients that may or may not be paralyzed.
Tear production decreases substantially within 30–60 minutes of the onset of general anesthesia, with or without preemptive administration of atropine, and this may persist for up to 24 hours after the anesthetic procedure. Consequently, corneal lubrication every 90 minutes during general anesthesia is recommended.
Transient lens opacification may occur in mice, rats, and hamsters during prolonged sedation or anesthesia. The opacification is believed to be caused by lack of blinking and subsequent evaporation of fluids from the shallow anterior chamber, which then resolves upon awakening.
If intraocular surgery is planned or globe rupture has occurred, application of topical ophthalmic medications including lubricants should be restricted to aqueous-based formulations. Petroleum-based ointments that gain access to intraocular structures may cause severe uveitis and further compromise vision and ocular comfort. Taping the palpebrae closed or performing a partial temporary tarsorrhaphy are additional techniques for protecting the globe and keeping it moist.
A smooth anesthetic recovery including appropriate analgesia and prevention of self-trauma is the primary postoperative management goal. For patients who have undergone intraocular surgery periods of excitement, incoordination, coughing, gagging, or retching are particularly undesirable. Recovery should be in a quiet, dimly lit enclosure where external stimuli will be kept to a minimum. Patients can be kept comfortable and quiet by appropriate analgesia and sedation, although gentle physical restraint or words of reassurance while holding some small patients may be more effective. Elizabethan collars for small patients and padded helmets or protective eyecups for large patients may help protect their eyes but may not be well tolerated by some. Recovery cages should have extraneous structures such as feed-bowl rings removed to prevent eye trauma during recovery.
Ocular physiological considerations
Selection of an anesthetic protocol for intraocular surgery should include consideration of its effects on IOP, pupil size, and globe position. Success of an ophthalmic procedure may depend on control of IOP before, during, and after the procedure. The overall effect of most anesthetics is to decrease IOP. This reduction may be attributable to a combination of factors, including depression of diencephalic centers regulating IOP, increased aqueous outflow, decreased venous and ABPs, and relaxation of extraocular musculature.
Pupil size is controlled by iris smooth muscle that is controlled primarily by the autonomic nervous system. Parasympathetic stimulation of the iris constrictor muscle results in miosis (pupillary constriction), while sympathetic stimulation of the iris dilator muscle results in mydriasis (pupillary dilation). Pupil size is of greatest concern in cataract removal surgery, which requires the pupil to be widely dilated and the eye immobilized. Most anesthetic or sedative agents, with the exception of ketamine, will cause miosis. Opioids have variable effects on pupil size depending on the species. In cats they tend to cause mydriasis, while in dogs they tend to cause miosis. Prostaglandins, histamines, and other mediators of inflammation may also contribute to miosis, and antiprostaglandins and antihistamines are often administered prior to intraocular surgery. Any sympathomimetic, cholinergic, or anticholinergic drug applied topically to the eye also has the potential to affect pupil size. It has been suggested that mydriasis is more difficult to achieve after the onset of sedation or anesthesia, whereas mydriasis achieved prior to anesthetic induction or sedation is usually unaffected by the miotic properties of anesthetics and sedatives.
Globe motion during general anesthesia is not unusual. For corneal or intraocular surgery, any motion of the globe is undesirable. Excessive manual traction to maintain a stable globe position may cause expulsion of intraocular contents or initiation of the OCR. In addition, palpebral eye reflexes that may be evident at lighter planes of anesthesia may also interfere with procedures. Paralysis with NMBs or retrobulbar regional anesthesia during general anesthesia should eliminate ocular reflexes and enable positioning of the globe without excessive manual traction.
Cardiovascular physiological considerations
Many ocular patients are geriatric and the reader is referred to a subsequent section in this chapter for a discussion of anesthesia in geriatric dogs and cats. The depth of anesthesia required for adequate depression of ocular reflexes and globe motion necessary for intraocular surgery often results in pronounced hypotension and may represent additional risk for geriatric patients. This can often be managed intraoperatively by the use of NMBs, controlled ventilation, inotropic drugs, and adequate patient monitoring.
The OCR is a trigeminovagal (cranial nerves V and X) reflex that may be induced by pressure or traction on the eyeball, ocular trauma or pain, or orbital hematoma. Initiation of the reflex manifests as cardiac arrhythmias, which may include bradycardia, nodal rhythms, ectopic beats, ventricular fibrillation, or asystole. Although the OCR occurs most commonly during ocular surgery, it may also occur during nonocular surgery when pressure is placed on the eyeball. It has been suggested that the more acute the onset and the more sustained the pressure or traction, the more likely the OCR is to occur. Hypercapnea may significantly increase the incidence of bradycardia associated with the OCR.
Preemptive intramuscular anticholinergic administration during the preanesthetic period does not necessarily prevent occurrence of the OCR during general anesthesia. Additionally, use of antimuscarinics in patients with glaucoma may exacerbate intraocular hypertension. Treatment of the OCR should begin with discontinuing stimulation. If bradycardia persists, treatment with atropine (0.02 mg/kg IV) or injection of lidocaine into the eye muscles to prevent transmission along the afferent limb of the reflex may be effective. Precautions against initiation of the OCR should include assuring an adequate depth of anesthesia, maintaining normocapnia, and minimizing aggressive surgical manipulations.
Ophthalmic medications
Eye drops and ointments are concentrated medications that may cause systemic side effects especially when administered to very small patients. Systemic effects may be minimized by diluting topical medications and limiting their frequency of application. For a summary of commonly used topical ophthalmic medications that may impact anesthetic management, please refer to Table 18.1.
Anesthetic and sedative agents in ocular patients
Commonly used anesthetic and sedative agents may induce ocular side effects and impact the outcome of ocular procedures. For a summary of commonly used anesthetic and sedative agents and their ocular effects, please refer to Table 18.2.
Analgesic agents in ocular patients
Ocular and periocular structures are richly innervated and highly sensitive. Symptoms of ocular pain include blepharospasm, photophobia, ocular discharge, rubbing of the eyes, and avoidance behavior. A variety of topical and systemic drug therapies are currently available to address pain management adequately in ophthalmic patients. For a summary of commonly used analgesics and their ocular effects, please refer to Table 18.3.
Table 18.1.Ophthalmic medications with potential systemic side effects commonly used in dogs and cats presenting for ocular surgery

a A dministered intravenously.
b A dministered orally.
IOP, intraocular pressure.
Local and regional anesthesia in ocular patients
Local or regional anesthesia may be adequate for less invasive procedures or may be included as part of a balanced general anesthetic protocol. Topical anesthesia for diagnostic and therapeutic procedures in veterinary ocular patients usually requires accompanying sedation to gain cooperation of the patient.
Local anesthetics applied topically are readily absorbed through mucous membranes. Systemic toxicosis is possible, though unlikely, but judicious administration in small patients is recommended. Topical anesthetics can be irritating and cause transient conjunctival hyperemia as well as damage corneal epithelium, delay corneal wound healing, and mask signs of disease or discomfort. It is recommended, therefore, that topical anesthetics be reserved for diagnostic rather than therapeutic purposes. Tear production and the blink reflex will be reduced after topical anesthetic administration, necessitating the application of ocular lubricant to protect the cornea after completion of the diagnostic procedure.
Table 18.2.Ocular effects of anesthetics, sedatives, and anticholinergics
Drug | Class | Comments regarding use in ophthalmic patients |
Isoflurane Sevoflurane | Inhalant | • Dose-dependent ↓ IOP if normocapnia maintained • Use as part of a balanced protocol to ensure smooth recovery and positive ocular outcome • No arrhythmogenic potentiation with topical epinephrine administration |
Nitrous oxide | Inhalant | • Contraindicated with intraocular injection of gas/air into a closed eye (gas expansion may ↑ IOP); discontinue nitrous for > 15–20 minutes prior to air injection |
Thiopental | Injectable anesthetic | • ↓ IOP in normal and glaucomatous eyes |
Propofol | Injectable anesthetic | • ↓ IOP in normal and glaucomatous eyes |
Etomidate | Injectable anesthetic | • ↓ IOP directly but associated myoclonus may lead to ↑ IOP; therefore, coadminister with a benzodiazepine in patients with potential for globe rupture |
Ketamine | Dissociative anesthetic | • May ↑ IOP even when coadministered with benzodiazepines; therefore, avoid in patients with potential for globe rupture • May induce nystagmus → problematic in certain ocular procedures • Palpebrae remain open → predisposes to corneal drying • Pupils dilate and palpebral and corneal reflexes persist • Recoveries may be rough if not modified with other drugs |
Telazol® | Dissociative/benzodiazepine | • Similar to ketamine |
Atropine Glycopyrrolate | Anticholinergic | • May prevent the OCR or counteract bradycardia associated with administration of other vagotonic agents such as opioids • May make cannulation of the parotid duct more challenging in parotid duct transposition surgery • Potential for systemic anticholinergics to induce cycloplegia and mydriasis and ↑ IOP is unclear; glycopyrrolate may be less likely to induce ocular effects compared to atropine due to decreased penetration of end organs |
Dexmedetomidine Medetomidine | Alpha2 agonist | • Topical administration causes mydriasis and ↓ IOP in cats and rabbits • Systemic administration causes miosis and no change in IOP in dogs (miosis not evident with tropicamide pretreatment) • May be useful for postoperative sedation to ensure a smooth recovery |
Diazepam Midazolam | Benzodiazepine | • ↓ IOP • Conflicting evidence regarding ability to counteract ↑ IOP associated with ketamine administration |
Acepromazine | Phenothiazine | • Antiemetic effects may be useful in ocular patients • May be useful for postoperative sedation to ensure a smooth recovery |
IOP, intraocular pressure.
Table 18.3.Ocular effects of selected analgesics
Drug | Class | Comments regarding use in ophthalmic patients |
Morphine Hydromorphone Oxymorphone Fentanyl Butophanol Buprenorphine | Opioid | • Morphine ↓ IOP (other opioids assumed to have similar effects) • May induce emesis, which ↑ IOP; may need to delay administration until the patient is anesthetized if globe rupture is a concern • Induce vagotonic effects that may cause bradycardia and/or contribute to the OCR • Morphine causes miosis in dogs and mydriasis in cats (other opioids assumed to have similar effects) • Topical morphine (1%) reduces corneal pain in dogs with ulcers |
Carprofen Meloxicam Deracoxib Firocoxib Tepoxalin Flunixin | Nonsteroidal anti-inflammatory | • Appear to be effective for pain associated with corneal inflammation, uveitis, and other ocular pathology • Prevent intraoperative miosis • Used systemically and topically (topical application results in systemic absorption through nasal mucosa, therefore must coordinate systemic and topical use to avoid toxicity) • Topical use associated with irritation of conjunctiva, corneal cytopathy, ↓ aqueous outflow |
Lidocaine | Local anesthetic | • IV constant rate infusion provides comparable analgesia to morphine constant rate infusion for intraocular surgery in dogs |
IOP, intraocular pressure.
Topical administration of 1% morphine sulfate solution appears to provide local analgesia in dogs with corneal ulcers. The antinociceptive effect is possibly a result of interaction with mu opioid receptors, which have been identified in small numbers in normal canine corneas, and delta opioid receptors, which have been identified in the corneal epithelium and stroma of dogs. In contrast with the local anesthetics, this local analgesic effect is produced without delaying corneal wound healing or causing any discernible tissue damage.
Neuromuscular blockade for ophthalmic surgery
Paralysis of extraocular muscles relaxes the eye and allows the globe to roll centrally and proptose slightly. These effects greatly facilitate positioning of the globe for ophthalmic surgery, eliminating the need for significant surgical manipulation to obtain proper globe positioning and decreasing the potential for initiating the OCR. Mechanical ventilation is indicated for patients receiving neuromuscular blockers during general anesthesia to maintain normocapnia.
Depolarizing NMBs, such as succinylcholine, tend to increase IOP just prior to paralysis, while nondepolarizing NMBs, such as pancuronim, vecuronium, atracurium, and cisatracurium, do not appear to have this effect.
In glaucoma patients receiving treatment with an indirect-acting cholinergic agent (i.e., anticholinesterase), metabolism of succinylcholine may be delayed and paralysis induced by this NMB may be prolonged. It has been recommended that indirect-acting cholinergic drugs be discontinued 2–4 weeks prior to neuromuscular blockade with succinylcholine, although normal levels of plasma pseudocholinesterase activity may not be totally restored for 4–6 weeks.
While depolarizing NMBs are not reversible, the effects of nondepolarizing NMBs may be reversed with anticholinesterases such as neostigmine and edrophonium. Anticholinesterase administration is not associated with increased IOP. An anticholinergic (atropine or glycopyrrolate) is commonly administered prior to the anticholinesterase to prevent profound bradycardia. Alternatively, the use a nondepolarizing NMB with a shorter duration of action, such as atracurium or cisatracurium, may be indicated to avoid the need for reversal. Reversal of neuromuscular blockade should be complete to prevent hypoventilation, struggling during recovery, self-trauma, and increases in IOP.
Electroretinography and anesthesia
Electroretinography (ERG) is used primarily as a diagnostic test for progressive retinal atrophy or other degenerative retinal disorders, and to assess retinal integrity in dogs where ophthalmoscopic evaluation of the fundus is not possible prior to cataract surgery. Complete ocular akinesia is preferred for diagnostic ERG, which requires general anesthesia and possibly neuromuscular blockade. The ERG requires dark adaptation of the patient and is performed in the dark, which makes anesthetic monitoring a challenge. Inhalant agents depress the ERG in dogs but the results are considered useful as long as the ERG for the patient and the control animal are generated under identical anesthetic conditions. No single anesthetic protocol has been established for performance of ERGs, although propofol induction with isoflurane maintenance has been used successfully in dogs. Sedation with dexmedetomidine and an opioid such as butorphanol or nalbuphine, and a cooperative patient may prove adequate for a semiquantitative ERG as is typically performed for preoperative screening of cataract patients.
General considerations
The ideal anesthetic protocol for cesarean section would provide adequate hypnosis, analgesia, and muscle relaxation for optimal operating conditions without adversely impacting either mother or fetus. Because the physicochemical properties that allow sedative, anesthetic, and analgesic drugs to cross the blood–brain barrier also enable their placental transfer, it is not possible to selectively anesthetize the mother. Agents that affect the maternal central nervous system (CNS) will also produce fetal effects, resulting in fetal depression and potentially decreased viability. In many cases, cesarean section is an emergency procedure where the physical condition of the mother and fetus is less than optimal, making anesthetic management even more challenging.
Selection of an anesthetic plan for cesarean section should take into account the safety of the mother and fetus, provision of adequate patient comfort, and the veterinarian’s familiarity with the anesthetic technique. Factors in decision making include considerations of the physiological alterations induced by pregnancy and labor, the pharmacology of selected drugs and their direct and indirect effects on the fetus/neonate, the benefits and risks of the techniques chosen, and the risk of procedure-related complications associated with anesthetic management. Regardless of the anesthetic technique used, surgical expediency is crucial to decrease maternal recumbency time and fetal drug absorption. With prolonged uterine isolation prior to fetal delivery, placental perfusion decreases, resulting in fetal hypoxemia, acidosis, and distress.
Physiological alterations induced by pregnancy
Metabolic demands of gestation and parturition are met by altered physiological function. Although little work has been done in dogs and cats specifically, it is reasonable to extrapolate from other species. A summary of the major cardiovascular, pulmonary, gastrointestinal, and hepatorenal alterations induced by pregnancy is provided in Table 18.4.
Uterine blood flow during pregnancy
Maintaining stable uteroplacental circulation is important to fetal and maternal homeostasis and neonatal survival. Uterine blood flow is directly proportional to systemic perfusion pressure and inversely proportional to myometrial vascular resistance. Placental blood flow is mainly dependent on uteroplacental perfusion pressure but placental vessels have rudimentary mechanisms for changing vascular resistance. Obstetric anesthesia may decrease uterine and placental blood flow and thereby contribute to reduced fetal viability. In addition, uterine vascular resistance is indirectly increased by uterine contractions and hypertonia (oxytocic response). Decreased placental blood flow may occur with hypovolemia, anesthetic-induced cardiovascular depression, or sympathetic blockade producing reduced uterine perfusion pressure. Uterine vasoconstriction is induced by endogenous sympathetic discharge or by exogenous sympathomimetic drugs having alpha1 adrenergic effects (epinephrine, norepinephrine, methoxamine, phenylephrine, or metaraminol). Every effort should be made to avoid or minimize hypotension induced by anesthetic agents and increased uterine tone induced by ecbolic agents.
Table 18.4.Physiological alterations induced by pregnancy
Cardiovascular | Pulmonary |
↑ HR | ↑ MV |
↑ stroke volume | ↑ oxygen consumption |
↑ CO | o arterial pH |
↓ vascular resistance | o PaO2 |
↑ blood volume | ↓ PaCO2 |
↑ plasma volume | o total lung and vital capacity |
↓ PCV, hemoglobin | ↓ FRC |
↓ plasma protein | ↓ pulmonary resistance |
o ABP | o lung compliance |
o CVP (↑ during labor) | |
Gastrointestinal | Hepatorenal |
↑ gastric emptying | ↑ liver serum enzyme concentrations |
↑ gastric pressure | ↑ sulfobromophthalein sodium retention |
↓ gastric motility | o bilirubin concentration |
↓ pH of gastric secretions | ↓ plasma cholinesterase |
↑ gastric chloride ion and enzyme concentration | ↑ renal blood flow |
↑ glomerular filtration rate |
CVP, central venous pressure; o, no change; PaO2, arterial partial pressure of oxygen; PaCO2, arterial partial pressure of carbon dioxide. See text for definitions of other abbreviations.
Impact of physiological changes on anesthetic management
Parturients are at greater anesthetic risk than are healthy nonparturient patients because of pregnancy-associated physiological alterations and the often emergent nature of the surgery. Cardiac reserve diminishes during pregnancy and high-risk patients can suffer acute cardiac decompensation or failure. The use of ecbolic agents during or after parturition can also adversely affect cardiovascular function. Oxytocin in large or repeated doses induces peripheral vasodilation and hypotension, which can adversely affect both mother and fetus through decreased tissue perfusion.
Because functional residual capacity (FRC) is decreased, anesthetic-induced hypoventilation will induce hypoxemia and hypercapnia more readily in pregnant patients. Hypoxemia is further exacerbated by increased oxygen consumption during labor. Preoxygenation prior to anesthetic induction will increase oxygen reserve and is advisable if the patient is tolerant.
Inhalant induction occurs more rapidly due to increased alveolar ventilation and decreased FRC in the parturient. Additionally, increased progesterone and endorphin levels in the CNS decrease anesthetic requirements (i.e., the minimum alveolar anesthetic concentration is reduced). Thus, anesthetic induction may be extremely rapid, requiring as little as one-fourth to one-fifth the time required for nonpregnant patients, so care must be taken to prevent volatile agent overdose. Local anesthetic requirements are also decreased in the parturient.
As a result of altered gastric function, the risk of regurgitation (both active and passive) and aspiration is greater in parturients. Because increased gastric acidity and decreased gastric muscular tone may be present, metoclopramide and an H 2 antagonist drug (cimetidine, ranitidine, or famotidine) may be administered as part of the preanesthetic medication. Frequently, patients presented for cesarean section have been fed or the time of the last feeding is unknown. Parturients should be regarded as having a full stomach and anesthesia techniques should be selected that produce rapid airway control to prevent aspiration of foreign material. Incidence of vomiting is increased by hypotension, hypoxia, and toxic reactions to local anesthetics. Smooth induction of general anesthesia and prevention of hypotension during epidural anesthesia will decrease the incidence of vomiting. Because silent regurgitation can occur when intragastric pressure is high, a cuffed endotracheal tube is preferred for airway management. Atropine administration may increase gastroesophageal sphincter tone and help to prevent regurgitation, but it may also inhibit the actions of metoclopramide to increase gastric motility and emptying by sensitizing gastric smooth muscle to acetylcholine.
Decreased plasma cholinesterase levels may lead to prolonged action of succinylcholine in pregnant patients, particularly if they have been exposed recently to organophosphate parasiticides in flea collars or dips. Normal or slightly elevated blood urea nitrogen or creatinine levels may indicate renal pathology or compromise in parturient patients. It would appear wise in such patients to avoid the use of drugs with known nephrotoxic potential, such as methoxyflurane, aminoglycoside antibiotics, and nonsteroidal anti-inflammatory drugs (NSAIDs).
Placental transfer of drugs
The placenta is highly permeable to anesthetic drugs. The physiochemical properties that make a molecule a good anesthetic drug also enable rapid transfer across the uteroplacental interface. Anesthetic drugs administered to the mother cross the placenta and induce fetal effects proportionate to those observed in the mother. Placental transfer of drugs can occur by several mechanisms, but by far the most important is simple diffusion.
Diffusion across the placenta is determined by molecular weight (MW), the degree to which the drug is bound to maternal plasma proteins, lipid solubility, and degree of ionization. Drugs with low MW (<500 daltons), a low degree of protein binding, high lipid solubility, and poor ionization diffuse rapidly across the placenta. Most anesthetics and anesthetic adjuncts fit into this category. The muscle relaxant drugs are an exception and are generally regarded as having minimal placental transfer and negligible fetal effects. The placenta does not appear to metabolize anesthetics or anesthetic adjuncts.
The degree to which a drug is ionized is determined by its pKa and the pH of the fluid compartment. Drugs that are weak acids will be less ionized as pH decreases. For example, thiopental is a weak acid with a pKa of 7.6. In acidemic patients (pH < 7.4), a greater proportion of the administered dose is in the nonionized (nonprotein-bound) form. Consequently, acidemia tends to decrease the required anesthetic dose of thiopental and other barbiturates. Weakly basic drugs such as opioids and local anesthetics are more highly ionized at pH values less than their pKa. Thus, a greater fraction will be protein bound in acidemic states, which may potentially increase dose requirements. Because fetal pH is 0.1 pH unit less than that of the mother, weakly basic drugs (i.e., opioids, local anesthetics) are found in higher concentrations in fetal tissues and plasma compared to those of the mother because of ion trapping.
Fetal pharmacokinetics
Fetal drug concentrations are affected by redistribution, metabolism, and protein binding. The drug concentration in the umbilical vein is greater than drug exposure to fetal organs (brain, heart, and other vital organs). As much as 85% of umbilical venous blood initially passes through the fetal liver, where drug may be sequestered or metabolized. In addition, umbilical venous blood containing the drug enters the inferior vena cava via the ductus venosus and mixes with the drug-free blood returning from the lower extremities and pelvic viscera. Therefore, the fetal circulation buffers fetal tissues from sudden high drug concentrations. Binding of drug to fetal proteins may also reduce bioavailability. Fetal drug metabolism is not efficient, as microsomal enzyme systems have not reached full capacity in the fetus. Drug concentration and effects in the fetus can be considerably greater and last longer than in the mother. Fetal drug toxicity can be enhanced by fetal or maternal metabolism to more toxic metabolites and by drug interactions.
The administration of a fixed dose of a drug with rapidly decreasing plasma concentration (such as thiopental, propofol, or succinylcholine) briefly exposes the fetus and placenta to a high maternal blood drug concentration. This is in contrast to the sustained maternal blood levels of drugs administered by continuous infusion or inhalation, which result in continuous placental transfer of drug to the fetus.
Anesthetic drugs in the cesarean section patient
Anesthetic drugs and techniques should be carefully chosen and properly administered to minimize maternal depression and risk and to maximize neonatal vigor and viability. No agent should be used unless distinctly indicated. A brief overview of anesthetic drug classes in periparturient anesthesia follows. Please refer to Table 18.5 for a summary of drugs used in the perianesthetic period and their effects in the cesarean section patient.
Table 18.5.Effects of anesthetic agents in cesarean section patients
Drug | Class | Comments regarding use in cesarean section patients |
Atropine Glycopyrrolate | Anticholinergic | • Recommended in most to counteract ↑ vagal tone associated with uterine traction • May ↓ incidence of emesis? • ↑ gastric pH → may reduce severity of aspiration pneumonia if it occurs • Glycopyrrolate does not readily cross the placenta → fewer fetal effects compared to atropine |
Acepromazine | Phenothiazine | • Induces significant maternal and fetal neurobehavioral depression • Long lasting and not reversible • Contraindicated for routine use |
Medetomidine Dexmedetomidine | Alpha2 agonist | • Induces significant maternal and fetal circulatory and neurobehavioral depression • Contraindicated for routine use |
Diazepam Midazolam | Benzodiazepine | • Induce neonatal depression • Neonatal effects may be dose dependent but safe doses have not been determined for use in dogs and cats • Reversible (in mother and/or neonate) with flumazenil • Not recommended for routine use |
Morphine Hydromorphone | Opioid | • Induce neonatal respiratory and neurobehavioral depression |
Oxymorphone | • Fetus may require 2–6 days to eliminate | |
Fentanyl Butorphanol | • May cause maternal emesis if given in the preanesthetic period (especially morphine) | |
Buprenorphine | • Reversible in neonate with naloxone given sublingually but renarcotization may require redosing | |
Thiopental | Thiobarbiturate | • Rapid induction in the mother • Rapidly cleared from neonatal circulation • Neonatal suckling activity may be decreased post-thiopental |
Propofol | Isopropylphenol | • Rapid, smooth induction in the mother • Rapidly cleared from neonatal circulation • Associated with ↑ puppy vigor, vocalization, and survival postsurgery • Injectable drug of choice for induction of anesthesia |
Etomidate | Carboxylated imidazole | • Rapid induction but myoclonus is possible • Rapidly cleared from neonatal circulation |
Alphaxalone-CD | Steroid | • Rapid, smooth induction in the mother • Appears promising but limited data in this patient population |
Ketamine | Dissociative | • ↑ puppy risk → respiratory depression, apnea, ↓ vocalization, ↑ mortality at birth • Not recommended for routine use |
<allcaps>Telazol®</allcaps> | Dissociative/benzodiazepine | • Limited data available in this patient population • Not recommended for routine use |
Isoflurane Sevoflurane Desflurane | Inhalant | • Dose-dependent maternal and fetal depression • Rapid inductions and recoveries • Drugs of choice for maintenance of anesthesia • Suitable for induction of anesthesia in selected patients |
Nitrous oxide | Inhalant | • Potential adjunct to volatile inhalants • Minimal neonatal depression and no neonatal diffusion hypoxia if administered at concentrations < 60% |
Succinylcholine Mivacurium Vecuronium Atracurium | Muscle relaxant (short to intermediate acting) | • Minimal placental transfer → minimal neonatal effects • May be useful adjunct in a balanced anesthetic protocol • Avoid long-acting agents such as pancuronium |
Lidocaine Bupivacaine Mepivacaine Ropivacaine | Local anesthetic | • Minimal neonatal effects when appropriate doses used for epidural administration • Sympathetic blockade may cause maternal hypotension → uterine hypoperfusion if not treated |
General anesthesia for cesarean section
Cesarean section anesthesia can be accomplished either by regional or general anesthesia. Advantages of general anesthesia include speed and ease of induction, reliability, reproducibility, monitoring, and control. General anesthesia provides optimum operating conditions by providing a relaxed immobile patient. Tracheal intubation ensures control of the maternal airway, thereby preventing aspiration of vomitus or regurgitated gastric contents. In addition, it provides a route for maternal oxygen administration, thereby improving fetal oxygenation. When general anesthesia is administered properly, maternal cardiopulmonary function is well maintained.
General anesthesia may be more appropriate than regional anesthesia in selected clinical situations. These include maternal hypovolemia; prolonged dystocia, in which the mother is exhausted and the fetus is severely stressed; maternal cardiac disease or failure; morbid obesity, cases in which the mother is aggressive or fractious; and in brachycephalic dogs with upper airway obstruction. Finally, most veterinarians are more confident of their ability to induce general anesthesia safely than to use regional anesthesia techniques in dogs and cats.
General anesthesia does have certain disadvantages. It will likely produce greater neonatal depression than will regional anesthesia. Inadequate anesthetic plane causes maternal catecholamine release, which may result in hypertension and decreased uteroplacental perfusion, leading to both maternal and fetal stress and deterioration of cardiopulmonary function. Loss of airway protective reflexes following anesthetic induction may produce aspiration and airway management challenges when the trachea is not properly intubated. Several selected general anesthetic protocols for canine and feline cesarean section patients are provided in Table 18.6.
Table 18.6.Selected general anesthetic techniques for elective and emergency cesarean section patients
Protocol | Comments |
• ± Anticholinergic • Propofol IV to effect for induction • Endotracheal intubation • Inhalant for maintenance • Opioid IV following fetal removal • ± Single-dose NSAID (depending on maternal risk factors) following fetal removal | Suitable for elective cesarean sections |
• ± Anticholinergic • Fentanyl (0.003–0.005 mg/kg IV) • Propofol IV to effect for induction • Endotracheal intubation • Inhalant for maintenance • Additional opioid (because of fentanyl’s short duration) following fetal removal • ±Single-dose NSAID (depending on maternal risk factors) following fetal removal • Treat neonates with Naloxone | Suitable for elective or emergency cesarean sections |
• Inhalant induction via face mask • Endotracheal intubation • Inhalant for maintenance • Opioid following fetal removal • ±Single-dose NSAID (depending on maternal risk factors) following fetal removal | Suitable for emergency cesarean sections |
Maternal oxygen administration can significantly increase fetal oxygen content and can result in more vigorous neonates. Consequently, it is recommended for all cesarean section patients. Maternal tidal volume and minute ventilation (MV) must be critically evaluated during the anesthetic period to avoid either hypoventilation or hyperventilation. The total effect of carbon dioxide on the fetus is not clear, but passive hyperventilation of the dam causes hypocapnia with decreased uterine artery blood flow. This decreased placental perfusion causes fetal hypoxia, hypercapnia, and acidosis. With adequate arterial oxygenation, a modest increase in arterial partial pressure of carbon dioxide (PaCO2) is well tolerated by the fetus. Adequacy of ventilation and oxygenation may be assessed by observing the rate of respiration, excursion of the chest wall and/or reservoir bag, and the color of mucous membranes. Capnometry and pulse oximtery are particularly useful pieces of monitoring equipment.
Regional anesthesia for cesarean section
This is a well-established technique for cesarean section in some species but is used less frequently in dogs and cats. Because there is a decreased dose requirement and enhanced distribution of local anesthetic agents during gestation and parturition, the dose of local anesthetic for epidural or spinal anesthesia can be reduced by approximately one-third in parturients. Regional anesthesia (epidural or subarachnoid) has the advantages of technique simplicity, minimal exposure of the fetus to drugs, less intraoperative bleeding, and, because the mother remains awake, minimal risk of aspiration. In addition, muscle relaxation and analgesia are optimal. In dogs, the subarachnoid space usually terminates at the level of the sixth lumbar vertebra, thereby reducing the risk of subarachnoid (true spinal) injection of the local anesthetic agent. In cats, the termination is variably between L7 and midsacrum, making subarachnoid injection a greater possibility.
Epidural anesthesia has been successfully used in dogs and cats for cesarean section anesthesia. Traditionally, a short-acting local anesthetic (2% lidocaine or mepivacaine) is administered at a dose of 1 mL per 3.25–4.5 kg of body weight into the epidural space to provide surgical site anesthesia. Alternatively, a 1:1 volumetric mixture of lidocaine and bupivacaine can be administered to extend the duration of surgical anesthesia and pain management into the early recovery period. This may be supplemented with epidural opioids and alpha2 adrenergic agonists.
Disadvantages of epidural or subarachnoid anesthesia include hypotension secondary to sympathetic blockade. Hypotension induced by epidural anesthesia can be managed with IV fluid and/or catecholamine administration. An isotonic crystalloid solution can be administered at approximately 20 mL/kg over 15–20 minutes to maintain ABP. When hypotension is severe, ephedrine may be administered (0.05–0.15 mg/kg IV). Ephedrine is considered the catecholamine of choice in this setting as it induces minimal effects on uterine blood flow and perfusion. Nausea and vomiting may also occur during the procedure and are usually associated with hypotension or visceral manipulation. Because the dam remains conscious, the forelimbs and head often move and gentle restraint is necessary. This precludes the use of a regional anesthetic technique in highly excited or fractious patients.
Care of newborns
Following delivery, the newborn’s head is cleared of membranes and the oropharynx of fluid. The umbilical vessels should be milked toward the fetus to empty them of blood, clamped approximately 2–5 cm from the body wall, and severed from the placenta. Neonates can then be gently rubbed with a towel to dry them and stimulate breathing. Vigorous motion should be avoided because amniotic fluid is readily absorbed in the lungs and contributes to distribution of pulmonary surfactant in the alveoli. The head and neck should be supported to avoid whiplash and prevent injury.
Flow-by oxygen administration in the vicinity of the muzzle is helpful to increase HR and oxygen delivery to tissues in distressed, exhausted neonates. Reversal of opioids by sublingual administration of 1–2 drops naloxone is warranted in cases where opioids were administered as part of the general anesthesia technique. An oral dose of 2.5% dextrose (0.1–0.5 mL) is helpful to improve energy substrates required to initiate breathing in stressed neonates. Finally, maintaining warmth is vital because hypothermia can occur rapidly after birth.
A small IV catheter may be used to intubate and support oxygen delivery in neonates that do not spontaneously initiate breathing, and breathing can be artificially supported by using a syringe and three-way valve attached to an oxygen source. As a final measure, doxapram can be used to stimulate breathing in neonates. In pups, a dosage of 1–5 mg (approximately 1–5 drops from a 20–22-gauge needle) is topically administered to the oral mucosa or injected intramuscularly or subcutaneously. In kittens, the dosage is 1–2 mg (1–2 drops). Airways must be clear before doxapram administration. External thoracic compressions may be warranted if HR is slow and does not respond to other supportive measures. A rapid physical examination checking for genetic defects (cleft palate, chest wall deformity, or abdominal wall fusion) is also important to determine viability.
After completion of surgery and recovery from anesthesia, the young can be introduced to their mother. If introduction is delayed, the neonates should be exposed briefly to the mother to provide colostrum and then kept in a warm environment until anesthesia recovery is complete to avoid accidental crushing. If regional anesthesia was used, neonates can be placed with their mother as soon as the surgery is complete.
Pain management
This represents a challenge in cesarean section patients because of concerns regarding transfer of analgesic drugs into milk and its impact on neonates. While data specific to dogs and cats are not available, it appears that short-term perioperative administration of postoperative opioids and NSAIDs is not associated with significant adverse effects in newborns.
Trauma and critically ill patients
General considerations
Anesthetic management in the traumatized or critically ill patient requires advanced planning, an ordered protocol, and efficient use of time and resources. A team approach to patient management in the emergency room, where each team member has specific preassigned duties, ensures rapid evaluation and treatment. The patient’s airway, breathing, circulatory, and neurological status must be assessed immediately upon arrival and reassessed frequently during the initial treatment period.
The initial physical examination must include, at a minimum, evaluation of respiratory rate and effort, HR and rhythm, pulse quality, capillary refill time (CRT), mucous membrane color, ABP, core body temperature, CNS function, and pain. A minimum panel of standard patient-side laboratory tests should be run, typically including assessment of packed cell volume (PCV), total solids (TS), blood urea nitrogen or creatinine, blood glucose, lactate, electrolytes, blood gas analysis or total carbon dioxide, activated clotting time, and urine specific gravity. Other diagnostic procedures including imaging, electrocardiographic (ECG) evaluation, and extended laboratory testing should be completed as indicated.
Severely traumatized or critically ill patients commonly develop serious complications within the first few days of presentation. Such complications may not be directly related to the initial insult but reflect overall tissue destruction, immune suppression, and metabolic imbalances. Critically ill patients are particularly prone to developing some form of shock, which is broadly defined as a generalized state of inadequate effective tissue perfusion.
When anesthetizing critically ill patients, the primary goal is to optimize tissue perfusion and oxygen delivery to vital organs while simultaneously ensuring a level of CNS depression resulting in unconsciousness, analgesia, and muscle relaxation. The ultimate goal should be optimal physiological function rather than restoration of measured hemodynamic parameters to “normal.” In fact, values considered normal for healthy anesthetized patients are associated with higher rates of mortality in high-risk patients. In humans, survivability is associated with cardiac indexes 50% higher and blood volumes greater than 100% higher than normal at the end of surgery.
Traumatized or critically ill patients are at increased risk of cardiopulmonary collapse. Common causes of acute circulatory failure include severe myocardial ischemia, malignant dysrhythmias, hypoxemia associated with severe lung injury or airway obstruction, hemorrhagic shock, acid–base or electrolyte abnormalities, and profound vasovagal responses.
Shock
The shock syndrome is commonly subcategorized into three distinct phases. The compensatory phase occurs early in the time course when the endogenous response is adequate to stabilize hemodynamic parameters. Modest increases in HR and respiratory rate may be noted but ABP and lactate levels remain within normal limits. Because clinical signs are not dramatic, this phase of shock may go unrecognized. The reversible or early decompensatory phase occurs when endogenous mechanisms of compensation are inadequate and hypotension, tachycardia, hypothermia, altered mentation, and lactic acidosis are documented. By definition, this stage remains amenable to intervention and treatment. The irreversible or terminal decompensatory phase is when prolonged tissue hypoxia results in permanent microvascular and organ damage. The sympathetic and neurohormonal response is exhausted and resuscitation during this phase will be ineffective.
There are numerous systems for classifying types of shock based on pathophysiology and more than one type may develop from a single etiology (Table 18.7). As a general rule, anesthesia should not be undertaken until vital organ functions have been assessed and stabilized. If the patient is in shock, the etiology should be determined and corrective measures initiated before anesthesia. A brief discussion of the most common clinical forms of shock in dogs and cats is presented below.
Systemic inflammatory response syndrome and septic shock
Systemic inflammatory response syndrome (SIRS) is a term applied to widespread inflammatory and immune activity occurring in response to sepsis or severe tissue trauma, injury, or necrosis. Criteria for diagnosis of SIRS in dogs and cats based on the presence of various key clinical signs have been proposed and are presented in Table 18.8.
Table 18.7.Classification system for different types of shock
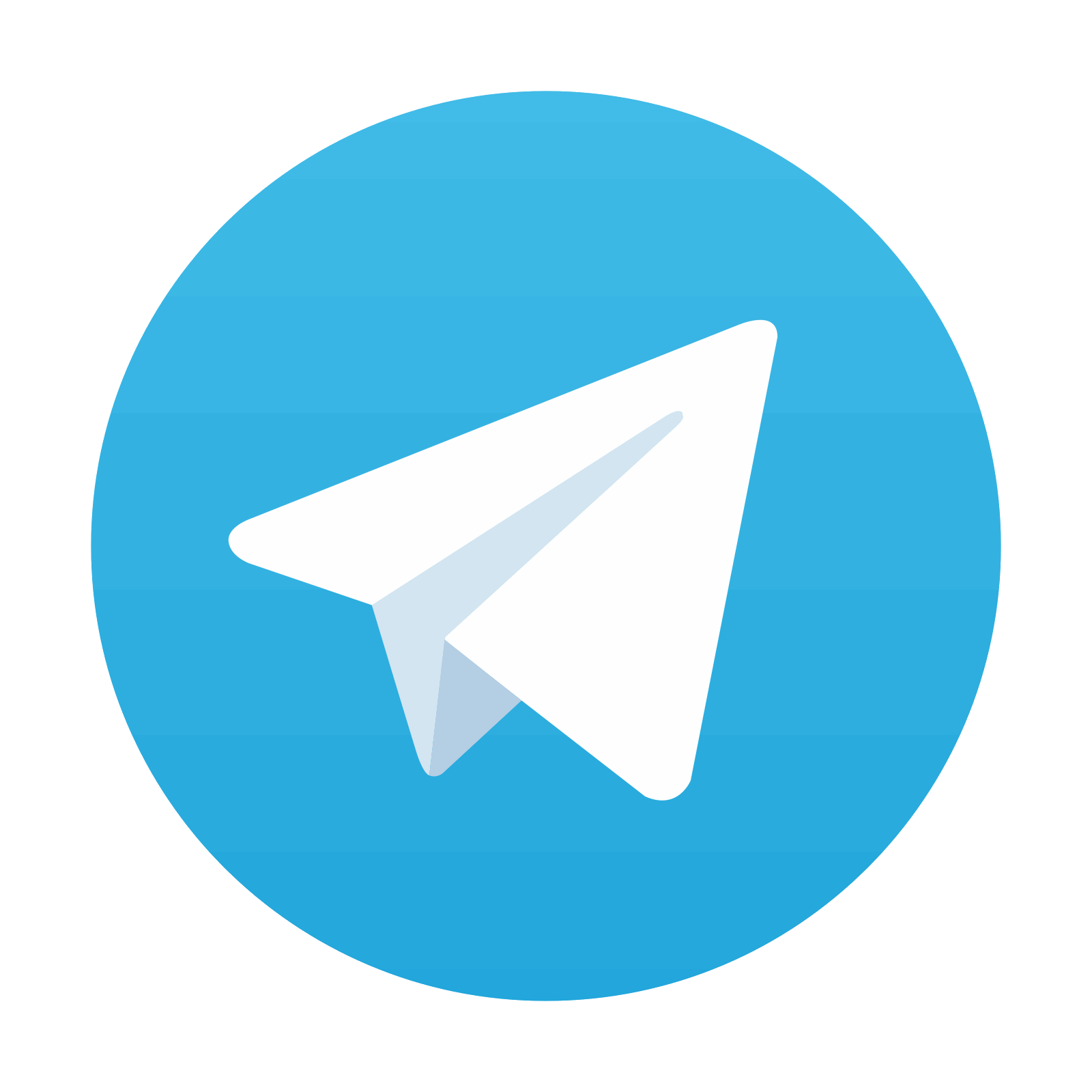
Stay updated, free articles. Join our Telegram channel

Full access? Get Clinical Tree
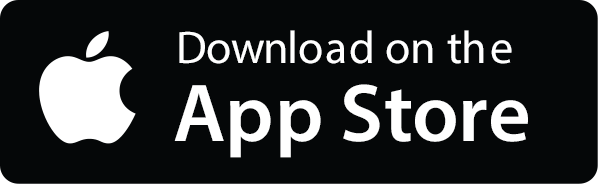
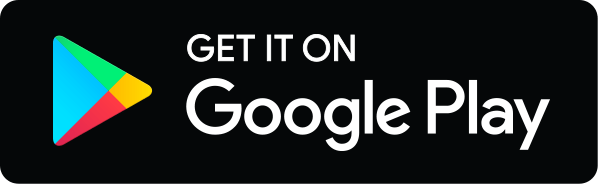